. Introduction
The family Orchidaceae is not only one of the most numerous, ecologically and morphologically diverse families of flowering plants, but also one of the most endangered plant taxa (Zhang et al., 2016). Orchids are one of the most popular ornamental plants worldwide. Moreover, within Orchidaceae family, epiphytes or litophytes with beautiful flowers are one of the most threatened groups because high demand leads to their overharvesting from wild populations (Hinsley et al., 2017; Mondragón, 2009).
Valued for their beautiful flowers, many wild epiphytic/lithophytic orchids, belonging to the genus Guarianthe Dressler & W. E. Higgins [G. aurantiaca (Bateman ex Lindl.) Dressler & W. E. Higgins, G. bowringiana (O’Brien) Dressler & W. E. Higgins, and G. skinneri (Bateman) Dressler & W. E. Higgins], are harvested from their natural habitats and sold as ornamental plants (Mondragón, 2009; Ticktin et al., 2020). Within its natural range, Guarianthe plants have been traditionally cultivated in home gardens. Additionally, the natural source of these plants has been significantly reduced due to their collection as medicinal plants, global climate changes, habitat loss, specificity of life history strategies, including specialized pollination syndromes and association with mycorrhizal fungi, and specific substrata (Gravendeel et al., 2004; Hinsley et al., 2017).
As the model organism of the study, we chose one of the Guarianthe species, G. bowringiana (O’Brien) Dressler & W. E. Higgins (“Guarianthe bowringiana,” 2021), which belongs to the Laeliinae subtribe (Epidendroideae, Orchidaceae), encompassing 1,500 species and 50 genera distributed within Neotropics (Dressler et al., 2005). In 2003, the Guarianthe genus was separated from Cattleya Lindl. based on several structural features of the plants and was supported by a recent molecular DNA analysis (Dressler & Higgins, 2003). Therefore, before the paper of R. Dressler and W. Higgins was published, G. bowringiana was known as Cattleya bowringiana Veitch (van den Berg et al., 2000), which is now considered as a synonym of G. bowringiana (Dressler et al., 2005; “Guarianthe bowringiana,” 2021).
Guarianthe bowringiana is native to Guatemala and Belize. These plants grow on rocky cliffs and on slopes of ravines near high-speed streams, where the air is saturated with moisture. In general, they are found on granite and limestone rock formations at heights of 100–1,000 m, but sometimes they are found on trees of mixed, pine-oak forests (Dressler et al., 2005).
Under glasshouse conditions, these plants reach more than 50 cm in height with fusiform pseudobulbs bearing two or three leaves. The leaves are elliptic to oblong-lanceolate. During cultivation, flowering occurs mainly in November and December (Figure 1A). The representatives of Laeliinae subtribe have been heavily harvested for horticultural purposes in recent years (Mondragón, 2009; Ticktin et al., 2020). Guarianthe bowringiana is listed in the Convention on International Trade in Endangered Species of Wild Fauna and Flora (CITES) Appendix II (Convention on International Trade in Endangered Species of Wild Fauna and Flora, 2020; International Union for Conservation of Nature, 2017).
Therefore, in order to conserve orchid plants in the wild and to meet the demands for ornamental and medicinal plant materials, developing new technologies for in vitro plant propagation is urgently needed. In vitro propagation of orchids to produce plants for commercial production and to re-establish the declining populations in the wild is an alternative that might help in decreasing the pressure on natural populations (Avila-Diaz et al., 2009; Coutiño-Cortés et al., 2017). To date, many native orchids species from South America and South-East Asia were propagated at the NBG through asymbiotic seed germination and tissue culture procedures (Buyun et al., 2004; Cherevchenko et al., 2007; Ivannikov, 2012).
Literature searching has revealed that the efforts of scientists are focused mainly on the optimization of various stages of in vitro propagation of tropical orchids (Astarini et al., 2015; Buyun et al., 2004; Coutiño-Cortés et al., 2017; Díaz-Álvarez et al., 2015). Moreover, various approaches towards efficient ex vitro establishment of the in vitro propagated orchids have been employed, such as using different in vitro culture media and various substrata (Ávila-Díaz et al., 2009; Pitoyo et al., 2015; da Silva et al., 2013).
Nevertheless, the adaptation of juvenile plants passing through in vitro ex vitro stress barrier, including structural adaptation and antioxidant system response was studied on a narrow range of objects, as evidenced by the few references in the scientific literature (Buiun, 2013; Gudiño et al., 2015; Torres et al., 2006; Torres & Sanabria, 2011).
Change of environment from in vitro to ex vitro conditions is considered as one of the strongest environmental stresses, which greatly reduces the success of any biotechnology project (Hazarika, 2006; Pospíšilová et al., 2007). It is believed that the main cause of plant mortality during acclimatization is water stress (Hazarika, 2003; Teixeira da Silva et al., 2017).
Therefore, to study the adaptive capacity of the seedlings obtained in vitro, it is necessary to take into account the changes in the anatomical and morphological structure and features of the antioxidant system (Batsmanova et al., 2008; Hasanuzzaman et al., 2020). Moreover, to the best of our knowledge, no studies have been carried out to assess the structural adaptation of G. bowringiana seedlings to abiotic stress during ex vitro acclimatization from early stage to developmental stages of generative individuals.
In view of the ornamental and conservational importance of G. bowringiana, this study was designed to (i) assess changes in the antioxidant enzyme activities, photosynthetic capacity, and lipid peroxidation levels during the early stages of acclimatization periods and to (ii) conduct a comparative anatomical analysis of juvenile leaves of G. bowringiana plants and adult plants cultivated in the conditions of greenhouse culture to find out directions of structural adaptation of plants as it transitions from in vitro to ex vitro environment.
This investigation was conducted as part of a conservation research program focusing on preventing the extinction of rare and endangered orchid species (Buyun et al., 2020).
. Material and Methods
Collection of Plant Material
The leaves of juvenile Guarianthe bowringiana seedlings grown in vitro and mature plants cultivated under glasshouse conditions were sampled at M. M. Gryshko National Botanic Garden (NBG, Kyiv, Ukraine).
Acclimatization of In Vitro Propagated Seedlings
An efficient protocol for asymbiotic in vitro seed germination of G. bowringiana has previously been established (Buyun et al., 2004; Cherevchenko et al., 2007) (Figure 1B,C). Seeds and young seedlings were grown on Knudson C medium modified in NBG by the addition of 2 g L−1 peptone, 50 mg L−1 potassium hummate, and 1 g L−1 activated charcoal. For proliferation of protocorms the Murashige and Scoog (MS) (1962) medium supplemented by 5 mg L−1 BAP and 2 mg L−1 NAA was used. The culture flasks were kept at 25 ± 2 °C, under a 16-hr photoperiod and a photosynthetic photon flux density (PPFD) of 45 µmol m−2 s−1.
Figure 1
General view of Guarianthe bowringianaflower (A), seeds (B), and successfully propagated in vitro seedlings, maintained at M. M. Gryshko National Botanic Garden (Kyiv, Ukraine) (C).
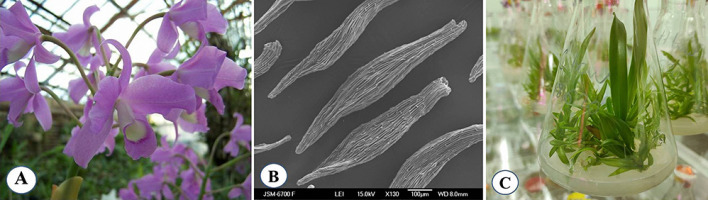
After 42 weeks, the seedlings (5.5–6.0 cm long) with five leaves and four roots were washed gently with tap water, and directly transferred to a glasshouse with 25 ± 2 °C ambient temperature and 75% ± 5% relative humidity. The seedlings were transplanted into square perforated plastic pots containing Sphagnum moss, covered with a plastic film to maintain high humidity, and maintained in the greenhouse under 60% shade. During acclimatization stage, pure Sphagnum moss was used as the sole potting medium.
To monitor the changes in the antioxidant enzyme activities, photosynthetic capacity, and lipid peroxidation levels, the leaves were sampled on Day 1, 2, and 7. Leaf surface micromorphology was evaluated from generative individuals raised from in vitro seedlings , cultivated under glasshouse conditions.
Scanning Electron Microscopy (SEM) Investigation of Leaf Surface Micromorphology
Samples for examination by SEM were kept in 100% tert-butyl alcohol for 1 hr at a temperature of −10 °C. Metal boxes with frozen samples were placed on a table cooled to −60 °C in the working volume of the vacuum universal post (VUP-5M). When vacuuming the working volume, the samples were dried due to the sublimation of frozen tert-butyl alcohol. The dried leaf samples were sputter coated with carbon in vacuum universal post (VUP-5M) and platinum in a JEOL JFC-1600 Auto Fine Coater. Visualization of leaf surface micromorphology of Guarianthe bowringiana was undertaken with JEOL JSM-6700F scanning electron microscope at 15 kV acceleration voltage in the high vacuum mode.
Photosynthetic Pigments Evaluation
Chlorophyll a (Chl a), chlorophyll b (Chl b), total chlorophyll (TC), and total carotenoids (Cx + c) concentrations were assessed following the methods described by Porra et al. (1989) using spectrophotometric assays. One hundred milligrams of leaf samples were collected. The sampled leaf material was placed in a 25 mL glass vial, along with 10 mL of 95.5% acetone, and blended using a homogenizer. The glass vials were sealed with parafilm to prevent evaporation and then stored for 48 hr. Samples were kept in darkness at 4 °C to avoid pigment degradation. The Chl a and Chl b concentrations were measured using a spectrophotometer (Shimadzu UV-1500) at 662 and 644 nm wavelengths. The Cx + c concentration was also measured by a spectrophotometer at 470 nm. A solution of 95.5% acetone was used as blank.
Concentration of pigments (in mg g−1 fresh weight of tissue) was calculated using the following equations (Wettstein, 1957):
Chlorophyll a concentration (Ca) = 9.784 × D662 − 0.99 × D664,
Chlorophyll b concentration (Cb) = 21.426 × D644 − 4.650 × D662,
Total chlorophyll concentration (Ca + Cb) = 5.134 × D662 + 20.436 × D644,
Total carotenoids concentration (Cx + c) = 4.695 × D470 − 0.268 × (Ca + Cb),
where D644, D662, and D470 are values of optical densities of the solution at wavelengths 644, 662, and 470 nm, respectively.
Statistical analysis of the data was done using the program Statistica 6.0. Probability of the difference between the arithmetic mean of indicators was established using the Student’s t test. The differences are considered to be significant at a value of p < 0.05.
Assay of 2-Thiobarbituric Acid Reactive Substances (TBARS)
To assess the stage of stress development, the lipid peroxidation process was evaluated by estimating the content of thiobarbituric acid (TBA) reactive substances, such as malondialdehyde (MDA) equivalents (Kumar & Knowles, 1993). Plant tissues (200 mg) were homogenized with 3 mL of 0.1 M Tris-HCl buffer (pH 7.6), and 1 mL of 0.67% TBA solution and 2 mL of 20% trichloroacetic acid was added to the homogenate. The reaction mixture was boiled in a water bath (95 °C) for 30 min. After cooling for 30 min, it was centrifuged at 1,500 g for 10 min at 25 °C. The absorption was estimated at 533 nm wavelength using the Shimadzu spectrophotometer. The content of TBA-reactive substances was estimated using the molar extinction coefficient of MAD with the following equation:
where D = optical density and ε (the coefficient of molar extinction for malondialdehyde) = 156 mM−1 cm−1, and l = the path length of cuvette (1 cm). The results were expressed as nmol MDA equivalents per gram of fresh weight.
Measurement of Antioxidant Enzyme Activities
To determine the activity of antioxidant enzymes, plant tissues were homogenized in liquid nitrogen after adding 50 mM potassium-phosphate buffer (pH 7.8). The obtained homogenate was centrifuged at 12,000 g for 15 min at 4 °C. The activity of superoxide dismutase (SOD, EC 1.15.1.1) was measured according to Giannopolitis and Ries (1977). For this purpose, 50 mL of the extract was added to the reaction mixture containing 1 mL of 0.0015% riboflavin, 1 mL of 5.82% methionine, and 1 mM of 0.154% of nitroblue tetrazolium. The absorption of the solution was estimated at 560 nm after incubation of the reaction mixture in light for 15 min. One unit of SOD activity was estimated as the amount of the enzyme inhibiting the rate of formazan formation by 50%, and expressed as unit U mg−1 protein.
. Results
The Photosynthetic Pigments in Juvenile Guarianthe bowringiana Plants Change After Transplantation to Ex Vitro Environment
Considering that photosynthetic system is the most sensitive indicator of the plant environment determining the plasticity of the species, we have investigated the changes in the content of the major photosynthetic pigments in the leaves of the in vitro grown seedlings transferred to ex vitro conditions. The obtained data is given in Table 1.
Table 1
Chlorophyll a (Chl a), chlorophyll b (Chl b), total chlorophyll (TC), and total carotenoid (Cx + c) content in Guarianthe bowringiana seedlings’ leaves during the early stage of ex vitro acclimation.
The first day after the transfer of G. bowringiana seedlings to the glasshouse, the content of Chl a increased by 21% and that of Chl b by 20%, compared with those grown in vitro (Table 1). On the second day of exposure, the content of Chl a and Chl b decreased insignificantly, but by the seventh day of exposure, the content of Chl a decreased, while Chl b content remained at the same level.
Additionally, the seedlings transferred to the glasshouse also exhibited an increased level of carotenoids (12%) after 2 days of acclimatization. An increase in carotenoid levels specifies that plants sustained the light stress during the early stage of acclimatization. Moreover, such an increase in carotenoids reflects the functional response of photosynthetic apparatus to the altered light environment, since the photoprotective role of carotenoids against photooxidative damage is well documented (Choudhury & Behera, 2001). Nevertheless, by the seventh day, the carotenoid levels decreased significantly (1.2-fold; p < 0.05) and were comparable to the baseline. It could be explained by the destruction of carotenoids due to photoinhibition. Furthermore, photoinhibition of photosystem 2 (PS2) in vivo is often considered as a photoprotective strategy rather than a damaging process (Choudhury & Behera, 2001).
Lipid Peroxidation Levels in the Leaves of Guarianthe bowringiana Seedlings as a Marker of Stress Development
MDA is the principal and most intensively studied product of polyunsaturated fatty acid peroxidation which can indicate the increase in oxidative stress (Batsmanova et al., 2008; Del Rio et al., 2005). Therefore, in order to assess the stage of stress development, the lipid peroxidation process was evaluated by estimating TBARS. Lipid peroxidation levels in the leaves of G. bowringiana seedlings transferred from cultivation vessels to glasshouses were estimated by determining the concentration of MDA, as presented in Figure 2. The lowest MDA value was detected in the nonstressed plants (in vitro). MDA content increased significantly after transferring to ex vitro conditions. After one day of exposure to glasshouse conditions, MDA levels increased about 1.5-fold (p < 0.05). Furthermore, a significant increase of MDA was found after 2 days (2.13 times compared to the in vitro conditions; p < 0.05). On the seventh day, this indicator returned to baseline, which may indicate a successful adaptation to altered growing conditions. Analysis of the obtained results of changes in the TBARS content in the leaves of G. bowringiana, showed that the transfer of plants propagated in in vitro culture to ex vitro conditions is a certain stress for them (Figure 2).
Figure 2
Changes in malondialdehyde (MDA) content during acclimatization of Guarianthe bowringiana seedlings after transferring from in vitro to ex vitro conditions. Bars represent the mean ± SE; a, b, c – different letters indicate significant differences at p < 0.05.
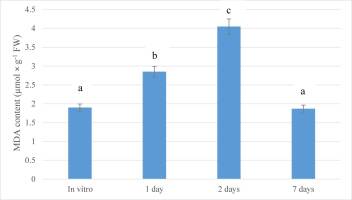
According to some authors (Ayala et al., 2014; Prasad et al., 1994; Sharma et al., 2012), the activation of lipid peroxidation (LPO) is a universal result of the impact of various stressors on the living system as the result of an enhanced oxidative catabolism of complex organic compounds. The products of this reaction act as the primary mediators of stress because the primary activation of lipid peroxidation is observed in the first stage of stress (“anxiety stage”), and is typical for both plants and animals, so the assessment of LPO intensity by accumulation of secondary products, namely TBARS, can be one of the criteria for the early diagnosis of stress (Del Rio et al., 2005).
Antioxidant Response of Guarianthe bowringiana Seedlings During the Early Stage of Ex Vitro Acclimatization
As summarized in Figure 3, the plants of G. bowringiana activated their antioxidative system in response to the altered conditions. In particular, just after 1 day of acclimation (drought stress), SOD activity increased significantly (1.7-fold; p < 0.05), compared to the values measured in control (in vitro) plants. The same trend was observed after 2 days (2.0-fold; p < 0.05) and seven days (2.2-fold; p < 0.05) (Figure 3). In general, the activity of the enzyme increased linearly during the experiment. Thus, it is indicated that the function of the defense enzymes in seedlings was intensified under altered conditions. After 7 days of exposure, this parameter was not monitored.
Comparative Micromorphology of the Leaves of Juvenile Plants Propagated In Vitro and of Adult Plants Cultivated Under Glasshouse Conditions
The leaf surface micromorphology of G. bowringiana juvenile plants propagated in vitro from seeds as well as that of adult plants cultivated in glasshouse was analyzed by SEM. SEM analysis of the leaf micromorphology of G. bowringiana plants under various cultivation conditions (in vitro and ex vitro) demonstrated that they all had the same basic structural characteristics (Figure 4), which are common for the species of Laeliinae subtribe in general and have been described previously (Stern & Carlsward, 2009). In vitro propagated G. bowringiana plants showed the presence of irregular epidermal cells with curved walls of varying sizes on both the adaxial and abaxial surfaces (Figure 4A,B). Stomata were located only on the lower surface of the leaf (hypostomatous leaves). The type of the stomata is mostly tetracytic which is characterized by two guard cells surrounded by four epidermal cells. Stomata are distributed regularly throughout the leaf surface. Stomatal distribution patterns on the leaves of both juvenile and mature plants grown under glasshouse conditions were the same. Stomata are usually scattered or distributed in small groups.
We observed a single stomata on the adaxial surface of the leaves in juvenile plants from in vitro culture (amphistomaty), apparently, induced by in vitro conditions (Buiun, 2013; Pospíšilová et al., 2007). It should be noted that the adaxial leaf surface view revealed the presence of stomata 6 months after the transfer of juvenile plants from the greenhouse to ex vitro conditions.
Nevertheless, on the leaves newly formed in G. bowringiana seedlings after the plants were transferred to glasshouse, stomata were not observed.
The adaxial leaf surface of adult plants is moderately undulate and pavement cells in the adaxial epidermis are difficult to recognize due to well-developed cuticle. Nevertheless, epicuticular wax structures on the adaxial surface have not been revealed. The cells of the abaxial epidermis of the leaves of adult plants are mostly rectangular or irregularly shaped, the walls are straight or curved, and the angles within adjacent boundaries are straight or pointed (Figure 4D).
During the acclimation of in vitro propagated plants to glasshouse conditions, the following changes in the leaf surface micromorphology were observed: (i) configuration of epidermal cells changed; (ii) dimensions of typical epidermal cells reduced; (iii) stomata density significantly decreased (p < 0.05), while their dimensions significantly increased (p < 0.05).
These data suggest that structural changes, can probably be regarded as an adaptation to avoid excessive rate of transpiration during a period of acclimation of the G. bowringiana juvenile plants to the glasshouse conditions. Furthermore, acclimation to CO2 enrichment often involves changes in leaf morphology, stomatal patterning, and structure of the leaf epidermis (Woodward & Williams, 1987).
Figure 4
Scanning electron microscopy micrographs of leaf surfaces of Guarianthe bowringiana juvenile in vitro seedlings (A,B); adult plants grown ex vitro (C,D); adaxial surface (A,C); abaxial surface (B,D).
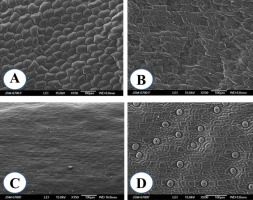
Investigation of leaf surfaces of both in vitro propagated seedlings and mature plants cultivated at glasshouse conditions has revealed that the number of epidermal cells in adult plants, derived from glasshouse conditions, significantly exceeded the number of cells in juvenile plants, 953.8 ± 47.1 and 781.5 ± 38.4, respectively (p < 0.05).
The stomatal density of in vitro and ex vitro transferred plants was 77.01 ± 12.97 and 39.16 ± 6.74, respectively (p < 0.05). In vitro propagated plants showed leaves with stomata that had raised, rounded guard cells (Figure 5A), compared with the normal elliptical, sunken guard cells (Figure 5B). The length of the stomata (guard cell length) was significantly shorter in in vitro propagated plants than in adult plants cultivated under glasshouse conditions, 24.3 ± 0.8 µm and 38.1 ± 0.7 µm, respectively (p < 0.05). In juvenile plants, the stomata protruded above the surface of the leaves (Figure 5A), while in adult plants they were located in shallow crypts (Figure 5B).
It is believed that a more elliptical shape is characteristic of a functional stomata, while a more spherical shape is frequently considered to be associated with a less functional stomata (Hassankhah et al., 2014).
. Discussion
Micromorphological Leaf Changes and Antioxidant Defense System of In Vitro Propagated Plants During Ex Vitro Acclimation
The conservation of endangered orchid species threatened with extinction in the wild can be aided by in vitro propagation, a procedure that enables massive production of plants for ex situ conservation, reintroduction or commercial purposes, thus reducing the pressure on natural populations (Ávila-Díaz et al., 2009; Lando et al., 2016; Lesar et al., 2012; Ortega-Loeza et al., 2011).
Environmental conditions for ex vitro growth are quite different from those used for in vitro cultivation (Hazarika, 2003, 2006; Kozai et al., 1991). Therefore, the advantages of the in vitro orchid propagation method can be fully realized only if the plants propagated in the sterile culture successfully adapt to ex vitro conditions. The altered leaf micromorphology, anatomy, and physiology of orchid plants under in vitro culture environment and during acclimation stage are rarely reviewed and predominantly restricted to plants of commercial importance like Dendrobium and Phalaenopsis (Cha-um et al., 2009; de Fario et al., 2004; da Silva et al., 2013; Teixeira da Silva et al., 2017).
An important functional and reliable diagnostic marker of the adaptation process in plants is the content and quantitative composition of pigments. It was revealed that plantlets cultured in vitro often show a low rate of photosynthesis and incomplete autotrophy, and these may be the reasons for low survival rates of plants during the acclimatization stage (de Fario et al., 2004; Jeon et al., 2006; Teixeira da Silva et al., 2017). Thus, the ability to photosynthesize, to maintain water balance, and respond to stress during and after in vitro culture determines the final performance of regenerants. It was revealed that the photosynthetic pigment contents of acclimatized Phalaenopsis plantlets decreased significantly after transplantation to in vivo environments (Cha-um et al., 2009). Our results are quite similar to a previous study on photosynthetic pigment stabilization in seedlings of Calanthe cardioglossa and Paphiopedilum appletonianum subjected to short-term cold stress after transferring from in vitro to ex vitro environment (Batsmanova et al., 2008).
On the contrary, the photosynthetic pigment content in the leaves of in vitro propagated G. bowringiana seedlings during the initial days was high. However, chlorophyll a/b ratio remained unchanged during the initial 7 days, while the total chlorophyll/carotenoids ratio increased. Thus, our findings suggest that photosynthetic capacity of in vitro propagated seedlings was not greatly affected by transferring to ex vitro environment. Consequently, we believe that by 300 days of in vitro cultivation of G. bowringiana seedlings, having 5.0 ± 0.24 leaves and 4.0 ± 0.19 roots, we will be able to ensure fully autotrophic growth. The survival rate of propagated in vitro seedlings was about 95%.
Recently, there has been a growing interest in the study of reactive oxygen species (ROS) and the antioxidant defense mechanisms during shoot organogenesis, embryogenesis, and ex vitro acclimatization of in vitro propagated plants (Eslami Fard et al., 2020; Gupta & Datta, 2003).
Under in vitro conditions, plants experience significant stress when exposed to extreme culture conditions (low light intensity, high relative humidity, limited gaseous exchange, higher sucrose or nitrogen levels, and exogenous growth hormones) resulting in morphological and physiological disorders (Ahmed & Anis, 2014).
Because plants are sessile organisms and cannot easily avoid stressful conditions, they have evolved numerous mechanisms for accommodating changes arising in their fluctuating growth conditions to enable functional adaptability under the influence of environmental factors without affecting cellular and developmental physiological processes (Arnold et al., 2019; Monforte, 2020).
Conditions in cultivation vessels under in vitro conditions (high humidity (over 95%), low light (30–80 µmol m−2 s−1), high sugar content in the culture medium, etc., cause the formation of juvenile plants with abnormalities in the anatomical structure of the leaf (malformed stomata, amphistomaty), which leads to certain changes in water status and even 100% plant death in greenhouse or field conditions (Hazarika, 2003, 2006; Pospišilová et al., 2007).
In vitro acclimatization is one of the key factors in producing healthy plantlets before they are transplanted to ex vitro conditions (Hazarika, 2003, 2006; Pospíšilová et al., 2007).
It is generally suggested that maintaining homeostasis in plants depends on the functions of regulatory systems on the various organizational levels. The increase in ROS levels resulting from many types of impact leads to the induction of antioxidant activities as part of the general adaptation syndrome, first described in medicine for human stress (Selye, 1950). Antioxidant enzymes are an essential part of the defense response.
It is believed, that in vitro orchid micropropagation is an efficient biotechnological strategy for conservation and commercial plantlet production. However, micropropagated plantlets generally need to adapt in order to survive the severe changes in humidity, irradiance, and growth medium that accompany the transfer to ex vitro conditions (Hazarika, 2006; Pospíšilová et al., 2007). The structural changes in the leaves of in vitro cultivated and ex vitro acclimatized plants using qualitative and quantitative analyses have been evaluated in Cattleya xanthina (L.) Van den Berg (Lando et al., 2016). It was observed that during acclimatization, a higher accumulation of dry mass, a greater convexity of the outer surface of epidermal cells, an increased deposition of epicuticular waxes, and a greater elongation of mesophyll parenchymatic cells occurred. Nevertheless, stomatal density was not changed. Furthermore, a need of gradual acclimatization, allowing the species to adapt its best to the new environment, has been emphasized (Lando et al., 2016).
Comparative vegetative anatomy of representatives of Laeliinae subtribe in vivo was studied by Stern and Carlsward (2009). In this subtribe, the exterior surface of the leaf becomes coated with a waterproof layer of cuticle and an extended ledge or lip forms around each stomatal pore, which is known as the outer cuticular ledge (OCL). It should be noted that in our study, the outer stomatal ledge was observed on the leaves’ surface of both juvenile and adult plants. The exact functions of this cuticular ledge are unknown, but it has been suggested this structure serves to prevent water loss by sealing the pore when the stomate is closed, and to prevent water droplets from entering when the pore is open (Pautov et al., 2017).
Comparison of the leaf micromorphology of in vitro and ex vitro grown G. bowringiana plants did not reveal malformed stomatal apparatus in the former. Moreover, the stomatal apparatus of in vitro seedlings was normal, although the stomatal density was increased, which indicates that these plantlets were more likely to be able to adapt to environmental conditions of the glasshouse.
The decreased stomatal density in adult plants cultivated under glasshouse/field conditions probably could be explained considering the fact that elevation of atmospheric carbon dioxide concentration often results in low stomatal density (Woodward & Williams, 1987). In addition, the higher stomatal density under in vitro conditions may also be influenced by growth hormones as reported in Leonurus cardiaca by Stefanova and Koleva (2014).
Alterations in the shape of the stomata directly affects its functionality. It is believed that a more elliptical shape is characteristic of functional stomata, while a more spherical shape is frequently associated with less functional stomata (Hassankhah et al., 2014).
Our findings are in line with data of Torres and Sanabria (2011), who observed that in Cattleya jenmanii Rolfe and C. lueddemanniana Rchb. f. (Orchidaceae) the adjustment to ex vitro conditions were not completed during eight weeks of acclimatization.
MDA, a lipoxidation end product of polyunsaturated fatty acids, is very often used as a suitable biomarker for lipid peroxidation to measure the level of oxidative stress (Bailly et al., 1996).
The response of plants to the action of stressors and the preservation of homeostasis is the basis of adaptability (Arnold et al., 2019; Monforte, 2020). When there is a change in the environment, seedlings primarily react by transforming the physiological and biochemical processes in order to adapt to altered new environment.
The accumulation of MDA, a byproduct of oxidative damage of membrane lipids, in G. bowringiana seedlings under altered (water stress) conditions is indicative of increased lipid peroxidation. These findings are in agreement with the observations reported for other orchid plant species. For example, Batsmanova et al. (2008) showed increased MDA contents in cold-stressed (15 °C, 7 days) in vitro propagated seedlings of orchid species, namely Calanthe cardioglossa, Guarianthe bowringiana, and Paphiopedilum appletonianum, during the early stage (7 days of exposure) of acclimatization to ex vitro conditions.
As a marker that indicates response to oxidative stress, superoxide dismutase, considered as the first line of antioxidant defense enzymes are usually monitored. SOD converts the superoxide anion into hydrogen peroxide. Therefore, this enzyme is used as a biomarker of both oxidative damage and antioxidant defense enzymes in plants (Bose et al., 2017).
In this study, the intensity of SOD in the seedlings exposed to ex vitro conditions was much higher than in the in vitro seedlings, which justifies the ability of the plants with developed antioxidant enzymatic defense system to cope up with oxidative stress during ex vitro transfer (Ahmed & Anis, 2014; Laxa et al., 2019). The exhibited rate of increase of SOD activity allows us to suggest that the activity of this enzyme reached the highest value on the seventh day of acclimation stage. The further decline in the SOD activity may be correlated with the reduced oxidative stress and membrane peroxidation, which signifies adjustment of plants to the external stress during the latter phase of the acclimatization period (Bose et al., 2017).
Our findings are in accordance with the studies reported on enhanced activities of antioxidant enzymes during in vitro culture and acclimatization in micropropagated orchids such as Cymbidium giganteum Wall. (Kumaria et al., 1990) and Dendrobium fimbriatum var. oculatum (Kumaria & Tandon, 2000).
A similar pattern of increasing SOD activity during the acclimatization period has been previously documented in other orchid species such as Cattleahybrid and Cymbidiumhybrid (J. Li et al., 2001; Y. Li et al., 2004), Calanthe cardioflossa Schltr., and Paphiopedilum appletonianum (Gower) Rolfe (Batsmanova et al., 2008) when exposed to cold treatment (15 °C) for 7 days after transferring from in vitro culture to ex vitro conditions.
Implications for Conservation
The in vitro propagation and acclimatization of G. bowringiana and other orchids to produce plants of exceptional quality is an alternative that might facilitate conservation by decreasing the pressure on natural populations. Orchids, especially epiphytes with sympodial habit and inherited long life span, are well adapted for long-time cultivation under glasshouse conditions. Our findings suggest that orchids being long-lived plants are more suitable for long-term conservation than the plants from any other group. The samples of G. bowringiana have been maintained at NBG since 1973. Many limitations which are considered to restrict applicability of ex situ approach to conservation, such as loss of species integrity through spontaneous hybridization, are not so crucial in this case.
Since 1999, the whole collection of tropical and subtropical plants (including orchids) has had the status of National Heritage Collection of Ukraine and is supported by a State Funding. Besides, the NBG collection of tropical orchids is registered at the Administrative Organ of CITES in Ukraine (Ministry of Environment Protection, registration No. 6939/19/1-10 of June 23, 2004).
. Conclusions
Thus, the G. bowringiana plants adapting to the altered conditions passed through two successive phases of stress response. During the first phase (“anxiety phase”) lipoperoxidation process was activated, while the second phase was characterized by stabilization of pro-oxidant and antioxidant balance. In this case, it occurred on the seventh day of exposure to ex vitro conditions. It can be defined as a phase of resistance in which the intensity of LPO decreased due to the increase in antioxidant resources.
The new leaves formed during the ex vitro acclimatization stage (in glasshouse) exhibited the development of adaptive micromorphological features in in vitro propagated plants (decreased stomatal density and normal stomatal apparatus), which promotes the autotrophy enabling plants to survive under altered conditions (high light intensity and low relative humidity in comparison with in vitro culture conditions).
In conclusion, our findings indicate that the MDA content and SOD activity level could be used as early stress markers (the first line of defense) to assess the stress development, particularly during early acclimatization stage in orchids of different ecological groups and to estimate the tolerance limits when changing from in vitro to ex vitro conditions.
The evaluation of structural characteristics and specificity of their interactions with the environment allows us to optimize the growth conditions and enhance plant resistance through induction of natural defense mechanisms.
Additionally, these findings may serve as a basis for the commercial production of this threatened species.