. Introduction
Sugarcane (Saccharum spp.) is a significant global bioenergy crop (Vargas et al., 2014). Various products, including sugar, surfactants, and bioenergy, are produced from sugarcane (Wiedenfeld, 2000). Numerous factors affect sugarcane yield, such as cultivar, physiology, soil quality, economics (Santillán-Fernández et al., 2016), and the watering system management. Climatic features can be predicted and analyzed for proper crop production management based on historical regional meteorological conditions (Van-Ittersum & Rabbinge, 1997), while cultivar selection is also essential (Taratima et al., 2020). Sugarcane production is affected by drought stress in numerous zones (Santillán-Fernández et al., 2016).
Although sugarcane is a very robust species, in the initial growth phase, when it is most sensitive to water scarcity, the availability of water in the soil is an important factor influencing the growth and development of this crop (Mauri et al., 2017; Trentin et al., 2011). In Thailand, sugarcane is grown under rain-fed conditions (Laclau & Laclau, 2009). The planting season is October and November at the beginning of winter, with moisture remaining in the soil (Taratima et al., 2020). However, soil moisture content dramatically decreases due to drought and directly affects sugarcane growth and yield by up to 60% (Robertson et al., 1999).
Numerous studies have investigated the impact of drought on plant growth, including the responsiveness of morphological (Jangpromma et al., 2012), physiological (Schoonees, 2004), and biochemical features (Garcia et al., 2019). Drought stress also affects osmotic stress and disrupts ion transportation uptake systems (Abbas et al., 2014; Bajji et al., 2000; Malinowski & Belesky, 2019). Pressure alteration balancing is controlled by the water uptake process using osmosis. Under arid conditions, the plasma membrane is damaged by free radicals, leading to electrolyte leakage from cells (Taratima et al., 2020). As an adaptation to drought, water potential in plant cells is reduced, while carbon dioxide (CO2) concentration within both the palisade and spongy parenchymatous cells decreases, reducing photosynthetic and growth rates (Shao et al., 2008). Decreasing the photosynthetic rate reduces the content of fructose, glucose phosphate, and triose phosphate, which are important substrates for sucrose and starch synthesis during the day (Du et al., 1998; Garcia et al., 2019). Under water deficit conditions, starch present in the chloroplast may be used to adapt mechanisms to drought stress. Starch and sucrose are hydrolyzed by amylases (Schoonees, 2004) or invertases (Shivalingamurthy et al., 2018), respectively, to provide a carbon source for the production of proline and reducing sugars (Macneill et al., 2017; Thalmann & Santelia, 2017). Not only correlated to water deficit adaptation, invertases are also significantly affected by developmental stage and sucrose accumulation in sugarcane (Shivalingamurthy et al., 2018; Zhu et al., 1997).
Considering the anatomical features, drought stress treatment stimulated bulliform cell expansion, and leaf lamina and epidermal cells increased. Stomatal size decreases while stomatal density increases (Nawazish et al., 2006; Tatatima et al., 2019). Because the anatomical features show plasticity under drought stress, they can be effectively used in breeding programs to select parents of drought-tolerant cultivars (Taratima et al., 2020).
KKU-1999-02 is a hybrid cultivar between SLC92-90 (mother) × Chainat1 (father), while KKU-1999-03 is a hybrid cultivar of K84-200 (mother) × Co775 (father). Both of these cultivars were released according to Khonghintaisong, Songsri, & Jongrungklang (2020), their agronomic traits are high tiller with approximately eight stalks/stool. KKU1999-03 exhibited higher stalk dry weight and biomass than KKU-1999-02. However, both cultivars had high sugar content (Khonghintaisong, Khruengpatee, et al., 2020). Based on the above considerations, this study assessed the leaf anatomical features of sugarcane KKU-1999-02 and KKU-1999-03 under drought stress. Anatomical structure alteration information from this report can be applied to sugarcane breeding programs and for the growth management of sugarcane.
. Material and Methods
Plant Material and Experimental Details
All experiments were carried out with pot-grown plants between March and June 2018 at the Agronomy Field Crop Station, Faculty of Agriculture, Khon Kaen University, Khon Kaen, Thailand. Anatomical features of KKU-1999-02 and KKU-1999-03 with different drought tolerances were analyzed using probes sampled from July to December. Eighty kilograms of dry soil were filled into four layers in a standard pot (80 cm in height and 50 cm in diameter). Each layer contained 20 kg of soil at a density of 1.55 g/cm3. The soil contained 77.9% sand, 20.0% silt, and 2.07% clay, and was characterized by the following parameters: 11.3% field capacity (FC), 4.06% permanent wilting point (PWP), 0.337% organic matter (OM), pH 6.08, 0.26 ds/m electric conductivity (EC), and 1.55 g/cm3 bulk density (Khonghintaisong, 2018). One sugarcane culm (approximately 6 cm in length, 57–60 g) was used per pot in all treatments. Basic fertilizers at 50 kg N, 50 kg P, and 25 kg K/ha were used during the cultivation period, while weeds and insects were manually controlled. The full water program was applied once a day within 30 days after planting for seedling growth promotion in all treatments. Soil moisture was applied constantly and documented throughout the study period for the control (nonwater stress treatment), while water was restricted from 30–90 days after planting the cultures for the drought treatment. The amount of water followed the crop water requirement (ETcrop), and soil moisture content was determined at 15-days intervals during the drought period using the microauger method at soil depths of 0–80 cm. All water used in the experiment was applied based on the crop water requirements calculated by Jangpromma et al. (2010) as follows:
where ETcrop is the crop water requirement (mm/day), ETo is the evapotranspiration of a reference crop under specified conditions calculated by the evaporation pan method, and Kc is the crop water requirement coefficient for sugarcane.
Anatomical Studies
The fourth mature leaves, approximately 160 cm long from the shoots of all treatments, were used as samples. Fragments of 10 cm length of the median region of the leaf blade were fixed in 100 mL of FAA70 fixative (70% ethyl alcohol, acetic acid, formaldehyde; 90:5:5) (Johansen, 1940). At least three probes were collected for anatomical studies of the leaf margin, midrib, and lamina. Each probe was dissected into small pieces (approximately 1 × 1 cm) before soaking in 15% (v/v) Clorox (sodium hypochlorite) for 24 hr before peeling, washed with distilled water, and stained with 1% (w/v) safranin O in ethyl alcohol for 2 min. Adaxial and abaxial epidermal peels were dehydrated using serial ethyl alcohol and xylene before mounting in DePeX (Taratima et al., 2019). Stomatal size (guard and subsidiary cells), stomatal density, and short and long epidermal cells were measured using a light compound microscope. The representative probes of the three areas of the leaf blade, as mentioned before, were freehand transversally sectioned. Sections were stained using 1% (w/v) safranin O solution in ethyl alcohol for 10 min before dehydration with serial ethyl alcohol and xylene and mounted using DePeX. All anatomical characteristics were observed and measured using a light compound microscope CH30 (Olympus) and a Zeiss Primo Star using the MB2004 configuration AxioVision program. Anatomical features were analyzed and described in accordance with the methods proposed by Nawazish et al. (2006), Taratima et al. (2019), and Zhang et al. (2015).
Data Analysis
Five replicates of each treatment were performed. The paired sample t test was used to determine the statistical significance (Taratima et al., 2019). All anatomical characteristics were investigated using the Drought Tolerance Index (DTI) to compare the values under drought stress based on Nautiyal et al. (2002) (more than 1 = increase, less than 1 = decrease) as follows:
. Results
Epidermis samples stripped from the leaves of both cultivars studied under field capacity and drought stress conditions revealed the anatomical characteristics of grasses, especially epidermal features, such as bulliform cells (Figure 1A,B,E,F), cell wall and cuticle thickness of epidermal cells (Figure 1C,D,G,H), stomatal type, and long-cell and short-cell patterns (Figure 1I–L). Transverse sections displayed C4 plant features, with Kranz anatomy and radial arrangement of mesophyll cells around the prominent Kranz sheath (Figure 2A–D). The vascular bundles were of the closed collateral type and had an angular outline (Figure 2E–H).
Figure 1
Sugarcane leaf transverse (leaf margin) (A–H) and epidermal peels (I–L) sections (lamina) of KKU-1999-02 (A–D,I,J) and KKU-1999-03 (E–H,K,L) cultivars for field capacity (FC) and drought stress (DS) treatments from 30 to 90 days. (A,B,E,F) Adaxial epidermis showing bulliform cells (BC); in (A): vertical length (VBC) and horizontal length (HBC) of bulliform cells. (C,D,G,H) Epidermal cell wall thickness (arrows). (I–L) Abaxial stomata and epidermal cell with FC (I,K) and DS (J,L); in (I): stomatal width of adaxial side (SWA) and stomatal length of adaxial side (SLA).
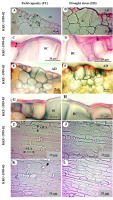
Under drought stress, KKU-1999-02 and KKU-1999-03 showed different anatomical adaptation characteristics. Lamina thickness of the two cultivars significantly decreased (DTI = 0.98), while the cell wall and cuticle thickness at the midrib region of the adaxial sides of KKU-1999-02 and abaxial sides of KKU-1999-03 significantly increased, DTI was 1.59 and 1.56, respectively (Table 1). Almost all measured traits of major vascular bundles in the stress treatment were higher than those in the control (unstressed), in KKU-1999-02 (Table 1, Figure 2), while other anatomical characteristics were significantly higher than the control. However, some traits were significantly lower than those of the control. For major vascular bundles of midrib measurements, only four parameters [vertical length, horizontal length, first vessel diameter (metaxylem), and second vessel diameter (metaxylem)] were significantly lower in the cultivar KKU-1999-02 than the control. Moreover, in stomatal size measurements of the KKU-1999-02 cultivar, only three characteristics [stomatal width (ad), stomatal length (ad), and stomatal width (ab)] were significantly lower than those of the control.
Figure 2
Sugarcane leaf transversal sections – lamina (A–D) and midrib (E–H) – displaying leaf blade thickness and vascular bundle size of KKU-1999-02 (A,B,E,F) and KKU-1999-03 (C,D,G,H) cultivars for field capacity (FC) and drought stress (DS) treatments from 30 to 90 days. (A–D) Leaf transversal sections; in (A): leaf thickness (LT) between adaxial (AD) and abaxial (AB) side. (E–G) Vascular bundle size; in (E): major vascular bundle size in vertical size (VV) and horizontal size (HV).
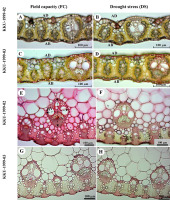
Table 1
Forty anatomical characteristics of Saccharum KKU-1999-02 and KKU-1999-03 under drought stress or field capacity (control) conditions.
The percentage changes in some interesting characteristics of the two cultivars are compared in Figure 3. In KKU1999-02, the major vascular bundle of midrib-vertical length (MVB-MR-VL), major vascular bundle of midrib-horizontal length (MVB-MR-HL), major vascular bundle of midrib – first vessel diameter (MVB-MR-Fr-VD), and major vascular bundle of midrib – second vessel diameter (MVB-MR-Se-VD) decreased, while the major vascular bundle of lamina-vertical length (MVB-LA-VL), major vascular bundle of lamina-horizontal length (MVB-LA-HL), major vascular bundle of lamina-phloem vertical length (MVB-LA-PhVL), and major vascular bundle of lamina-phloem horizontal length (MVB-LAPhHL) increased.
A comparison of the percentage of anatomical character measurement, increasing or decreasing percentage of some significant anatomical traits of sugarcane KKU-1999-02 and KKU-1999-03 are presented in Figure 3. The positive values indicate that the anatomical traits of the DS treatment showed higher measurements than the control, while negative values were lower than those of the control. Considering stomatal characteristics, the adaxial and abaxial stomatal densities of the two cultivars increased, especially in KKU-1999-03 (Table 1, Figure 3). The adaxial short-cell length (SCL-ad) and abaxial short-cell width (SCW-ab) of KKU-1999-03 increased under drought stress treatment. However, bulliform cell horizontal length (BCHL) increased in KKU-1999-02 but decreased in KKU-1999-03.
Figure 3
Increasing or decreasing percentage of some significant anatomical traits of sugarcane cultivars, KKU-1999-02 and KKU-1999-03, after drought stress treatment. MR-CWCT-ad = midrib epidermal cell wall and cuticle thickness (adaxial); MR-CWCT-ab = midrib epidermal cell wall and cuticle thickness (abaxial); MVB-MR-VL = major vascular bundle of midrib (vertical length); MVB-MR-HL = major vascular bundle of midrib (horizontal length); MVB-MR-Fr-VD = major vascular bundle of midrib (first vessel diameter of metaxylem); MVB-MR-Se-VD = major vascular bundle of midrib (second vessel diameter of metaxylem); MVB-LA-VL = major vascular bundle of lamina (vertical length); MVB-LA-HL = major vascular bundle of lamina (horizontal length); MVB-LA-PhVL = major vascular bundle of lamina (phloem vertical length); MVB-LA-PhHL = major vascular bundle of lamina (phloem horizontal length); ISCL-ad = interstomatal cell length (adaxial); ISCL-ab = interstomatal cell length (abaxial); STD-ad = stomatal density (adaxial) (No./mm2); STD-ab = stomatal density (abaxial) (No./mm2); SCL-ad = short cell length (adaxial); SCW-ab = short cell width (abaxial); LCL-ad = long cell length (adaxial); BCHL = bulliform cell horizontal length.
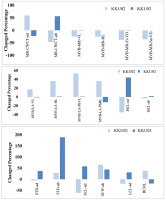
. Discussion
Adverse environmental stresses, both biotic and abiotic stresses, such as pathogen infections, stalk borer injuries, weed competition, improper temperatures, poor soil aeration and drainage, water deficit conditions, or vulnerable genotypes, affect the plant growth and development (Showler, 2016). However, drought stress also stimulates plant adaptation to growth, development, and survival (Boaretto et al., 2014). As shown in the present study, the lamina thickness of both experimental sugarcane cultivars decreased after drought stress treatment. This result concurred with our previous studies on sugarcane KK3 (Taratima et al., 2019), UT12, and UT13 (Taratima et al., 2020), but not with Junior et al. (2019), who reported that the specific leaf areas of some sugarcane genetic lines (RB931011, RB855536, and RB72454) were clearly reduced after drought stress treatment. A decrease in specific leaf area under water deficit indicates higher leaf thickness, which may facilitate the preservation of water in the leaf tissues, consequently allowing a more effective response to drought stress (Junior et al., 2019; Trujillo et al., 2013). However, drought tolerance levels may involve various factors, such as plant species, due to their leaf type, habit, developmental stage, and environmental status. The decrease in lamina thickness is an adaptation mechanism that protects plants from water deficit caused by leaf transpiration and affects the conductance of CO2 diffusion (Boughalleb et al., 2014). A reduction of water in the plant body promotes plant cell wall softening and reduces turgor pressure (Kapoor et al., 2020). Midrib major vascular bundle measurement of the studied cultivars, including vessel diameter, significantly decreased mainly in KKU-1999-02, but in both cultivars, vessels with thicker cell walls differentiated. Anatomical adaptation under drought stress was shown more clearly by KKU-1999-02 than KKU-1999-03. A decrease in vascular tissue size observed in this study concurred with Bosabalidis and Kofidis (2002), but not with our previous reports on sugarcane KK3 (Taratima et al., 2019), UT12, and UT13 (Taratima et al., 2020). A decrease in the size of some major vascular bundles in the midrib in this study was similar to that reported in wheat cultivars in which drought stress affects their vascular bundle size (David et al., 2017; Terletskaya & Kurmanbayeva, 2017). Under water deficit conditions, xylem cavitation is often induced, which results in embolism and transpiration inhibition (Qaderi et al., 2019). Drought stress may decrease vessel diameter (Pinto et al., 2012), which may help avoid cavitation (Zwieniecki & Secchi, 2015). Moreover, reductions in metaxylem diameter and vascular bundle size are normally found in plants under stress conditions, especially drought stress (Da Cruz Maciel et al., 2015) which may result in better resistance to water transportation (Pittermann, 2010). This is in accordance with the results of the present study that the metaxylem diameter of KKU-1999-02 decreased after drought stress treatment.
Bulliform cell size expansion is an important anatomical feature used by plants to mitigate drought stress. In this study, the bulliform cell sizes of the two cultivars expanded, except for the horizontal length of KKU-1999-03. The expansion of bulliform cells under drought stress was previously reported by De Micco and Aronne (2012), Nawazish et al. (2006), and Taratima et al. (2020). However, this result did not concur with sugarcane F127 and YL6 studied by Zhang et al. (2015) and KK3 Taratima et al. (2019), where bulliform cell size decreased as a result of drought stress treatment. Under drought stress, plants close their stomata to reduce transpiration (Medeiros et al., 2013), which results in low CO2 fixation and photosynthetic rates (Junior et al., 2019; Machado et al., 2009). However, the photosynthetic rate per leaf area unit did not change due to anatomical adaptation, such as reduced leaf thickness, increased chloroplast density per leaf area unit, increased stomatal density, and reduced stomatal size (Bosabalidis & Kofidis, 2002; Nawazish et al., 2006). Nevertheless, the stomatal response to drought stress may differ depending on the plant species.
. Conclusion
Adaptation under water deficit conditions is an essential process for plants. Various factors, including increasing cuticle thickness, epidermal cell size, vascular bundle, and stomatal density combined with reduced leaf area, leaf thickness, and stomatal size, are anatomical adaptations of plants to drought stress. These anatomical changes are important mechanisms to protect the plants from water deficit conditions by increasing the photosynthetic rate and reducing the water loss. This is the first report detailing the anatomical adaptation of sugarcane cultivars, KKU-1999-02 and KKU-1999-03, under drought stress. The results showed that the two sugarcane cultivars showed moderate drought tolerance. Therefore, these results contribute valuable basic knowledge for improving the understanding of anatomical adaptation devices of plants under water deficit conditions. The benefits of this study include a more profound knowledge of physiological traits to boost sugarcane breeding programs.