. Introduction
Tailings are sites of man-made mineral deposits. The Stebnyk tailing in Drohobych District, Lviv region, Ukraine, has formed because of an imperfect method of processing raw mineral material and now contains 22 million tons of waste (Snityns′kyĭ et al., 2015), including clay material, undissolved salts, brine with a high content of sodium chloride, and potassium-magnesium salts (Bilonizhka & Diakiv, 2009). This tailing has a negative influence on the environment because of the incursion of saline water into the surrounding hydrosphere (Pavliuk et al., 2013). Consequently, heavy metals (HMs) migrate from tailings to the soil and nearby reservoirs. On the tailing, there is gradual growth of the plant community, determined by changes in humidity and salinity (Fetsiukh et al., 2018).
Plants grown on polluted soil are subjected to several stresses, such as HM, salinity, lack of nutrients, physiological drought, and ion toxicity. These factors have a negative effect on growth, production, and various physiological and biochemical plant processes (Chutipaijit, 2016). The responses of plants to these stresses depend on the species, genotype, developmental and metabolic state of the plant, and duration and severity of the stress (Babeanu et al., 2017). It is now generally recognized that, due to such stresses, reactive oxygen species (ROS) are excessively accumulated in excess in plant cells (Yang et al., 2021). The neutralization of ROS in plants under oxidative stress is effectively ensured by the functioning of a multistage defense system that consists of high molecular weight compounds, antioxidant enzymes, and low molecular weight antioxidants (phenolic substances, vitamins, amino acids, and sugars) (Chiappero et al., 2021; Das et al., 2020; Eljebbawi et al., 2021; Mushtaq et al., 2020). Catalase (CAT) and peroxidase (POD) are enzymatic components that play a central role in plant defense systems (Chutipaijit, 2016). The balance between ROS production and antioxidant biosynthesis is very important for controlling redox homeostasis in plant cells (Gajić et al., 2018). Moreover, the antioxidant enzyme system is recognized as the main mechanism of plant tolerance to environmental stress (Alencar et al., 2021).
Willow (Salix spp.) is a pioneer plant species that is often used in nature-based solutions and eco-engineering projects. These plants are readily propagated from branches and cuttings, grow quickly in temperate climates, and can thrive in water-logged environments and contaminated soil (Gonzalez-Ollauri & Mickovski, 2020). Such plants play a crucial role in rehabilitating degraded land, restoring forest landscapes, and mitigating climate change (Jia et al., 2020). Moreover, willow plants have shown abiotic stress tolerance. This characteristic makes them suitable for phytoremediation of saline and industrially polluted land due to a variety of advantageous traits (Bilek et al., 2020).
The basket willow (Salix viminalis L.), also known as the energy willow, is characterized by a rapid and large increase in biomass and a broad tolerance to unfavorable environmental conditions (Wróbel & Mikiciuk, 2010). Scientists claim that cultivation of S. viminalis for energy purposes is a carbon sequestration activity and, thus, can help counter climate change (Janicka et al., 2021). Compared to the vast number of salinity experiments conducted with model plants and annual crops, studies of woody plants are limited (Huang et al., 2020). Research has been undertaken to study the problem of growing willows on different types of tailings (Bhattacharya et al., 2006; Harris & Jurgensen, 1977; Shi et al., 2017; Stoltz & Greger, 2002; Varga et al., 2009; Wilcox, 2013); however, there are as yet no published studies of S. viminalis growing on the tailing at Stebnyk. Our research aimed to analyze the salinity effect of the tailing on the antioxidant system of S. viminalis under field conditions.
. Material and Methods
Soil Characteristics and Experimental Design
Areas of the tailing were selected using the route method and according to the salinity and growth of salt-tolerant plants. As control sites, we chose plots with restored biocenosis (49°18′39.8″ N, 23°33′59.3″ E; 49°18′40.0″ N, 23°34′00.7″ E; 49°18′41.1″ N, 23°33′57.7″ E). The experimental plots contained pioneer glycohalophytes (49°18′45.0″ N, 23°34′07.7″ E; 49°18′43.8″ N, 23°34′07.8″ E; 49°18′43.3″ N, 23°34′07.4″ E) (Figure 1). The water-soluble ions in the soil were obtained earlier using the complexometric method (Kyiak, 2018). The main components of the soil in this region are chloride and sulfate (Table 1). The total salt content of the soil was 1.23 ± 0.16 and 2.49 ± 0.32 g kg−1 at the control and experimental plots, respectively. The content of water-soluble carbon (C) in the soil was determined via the Borodulina method (Huzar et al., 2016) and is shown in Table 2.
Figure 1
View of Stebnyk tailing (Drohobych District, Lviv region) (1 – control plots; 2 – experiment plots).
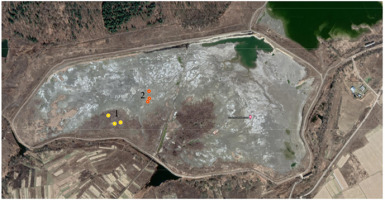
Table 1
Content of water-soluble ions in soil at the Stebnyk tailing, mg 100 g−1 of soil.
Table 2
Content of water-soluble carbon in soil at the Stebnyk tailing, mg kg−1.
Ctotal | Corganic | Cinorganic | |
---|---|---|---|
Control | 22.73 ± 0.52 | 16.47 ± 0.22 | 6.26 ± 0.73 |
Experiment | 10.88 ± 0.61 | 4.16 ± 0.12 | 6.72 ± 0.48 |
The area of one plot was 30 m2. Each plot was replicated thrice. The 150 rooted cuttings of S. viminalis with a length of cuttings ±25 cm and roots ±2 cm were planted in each plot. The content of enzymatic and nonenzymatic antioxidants in the leaves, stems, and roots of S. viminalis was determined after 120 days of growth.
Soluble Sugars Analysis
To determine the content of sugars in the roots, stems, and leaves, fresh tissue (0.5 g) was used. To extract alcohol-soluble sugars, fresh plant tissue (0.5 g) was crushed using 95% EtOH, after which the samples were boiled three times and centrifuged at 3,000 g for 15 min. Ethanol (80%) was then added to the samples. The contents were stirred and heated twice to boiling, cooled, and centrifuged. The dry material was dissolved in 5 mL of water and transferred to a 50-mL flask and made up to the mark. Next, a tenfold dilution of the resulting solution was performed. The solution was transferred to test tubes, and 1 mL of 5% phenol solution and 5 mL of H2SO4(conc) were added. The samples were shaken and incubated for 10 min at room temperature. For the determination of water-soluble sugars, the samples were extracted with water at a 1:20 ratio for 2 hr and then filtered. The filtrate was collected, and 1 mL of 5% phenol and 5 mL of H2SO4(conc) were added, mixed thoroughly, and cooled. Sugar content was determined using a spectrophotometric method at a wavelength of 490 nm (Dubois et al., 1956). The sugar content was determined according to the calibration schedule, which was based on sucrose.
Proline Content
Proline extraction was performed by boiling plant samples [300 mg of plant material (H)] in 5 mL of dH2O (10 min). After centrifugation (20 min, 1,000 g), 1 mL of supernatant was added to 1 mL of CH3COOH and 1 mL of ninhydrin reagent. The samples were incubated for 1 hr in a boiling water bath and cooled rapidly on ice. Color intensity (A) was measured at a wavelength of 510 nm (Bates et al., 1973). The proline content was estimated using the following formula:
Estimation of Ascorbic Acid Content
To determine the ascorbic acid content, 5 g of plant material (H) was homogenized in 2% metaphosphoric acid, with an adjusted volume of 100 mL, and then centrifuged. The extract of the samples (10 mL) was transferred to conical flasks, after which 1 mL of 0.025% solution of 2,6-dichlorophenolindophenol was added. After 35 s, the ascorbic content was measured at a wavelength of 530 nm in a cuvette with a working length of 10 mm against 2% metaphosphoric acid. The calculations were performed according to the calibration schedule (A) (Musiienko et al., 2001). Ascorbic acid (AsA) content (mg 100 g−1 fresh weight) was estimated using the formula:
Determination of Catalase and Peroxidase Enzyme Activities
CAT activity (µmol H2O2 mg−1 protein) was assayed as described previously by Koroliuk et al. (1988). Fresh plant material (1 g) was homogenized in 3 mL of buffer and then centrifuged for 15 min at 1,000 g. The catalase reaction was initiated by adding 20 mL of 0.03% H2O2 to 1 mL of homogenate. The reaction was stopped after 10 min by adding 10 mL of 4% ammonium molybdate solution.
To measure POD activity (µmol H2O2 mg−1 protein), fresh plant material (0.5 g) was homogenized in acetate buffer (pH = 4.7). After 10 min of infusion with periodic stirring, the extract was centrifuged at 1,000 g for 15 min. The enzyme solution, 2 mL of benzidine, and 2 mL of distilled H2O were poured into tubes. Enzyme activity was determined as described by Gavrilenko et al. (1975, pp. 284–285) by measuring the absorbance at 625 nm.
. Results
The data shown in Table 3 and Table 4 indicate that tailing pollution influenced the leaves, stems, and roots of S. viminalis. Salinity reduced all assessed growth parameters. The number and length of stems were less than twice that of the control. Furthermore, the number of leaves was 25% lower than that of the control plants. The leaf area and root system of the plants were similarly affected. The volume of the root system was two times smaller than that of the control plants.
Table 3
Growth of Salix viminalis at the Stebnyk tailing, control and experimental plots.
2 months of growth | 4 months of growth | |
---|---|---|
Control | ![]() | ![]() |
Experiment | ![]() | ![]() |
Table 4
Effect of salinity on morphometric parameters of Salix viminalis under field conditions at Stebnyk tailing, 120 days of growth.
The results of our research show that the proline content in the stems of S. viminalis was higher than that in the leaves and roots of these plants under high salinity (Figure 2). The proline content in the stems of S. viminalis was 75% higher than that in the control group. In contrast, the content of proline in the roots decreased by 19% compared to the control. However, the proline content in the leaves was similar to that in the control.
Figure 2
Content of proline in Salix viminalis under salinity condition (field condition, 120 days of growth). * Significant difference compared with the control, p < 0.05.
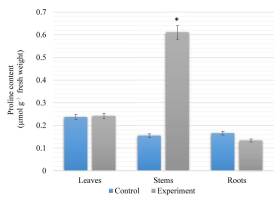
The data presented in Figure 3 indicate that there was an accumulation of sugars (alcohol- and water-soluble) in the organs of willow plants under field conditions. The highest amount of total sugar was detected in roots under salt stress conditions. In contrast, no changes were observed in the stems. The total sugar content in the leaves and roots was 7.69% and 35.74% higher, respectively, than that of the control plants.
Figure 3
Content of soluble sugars in Salix viminalis under salinity condition (field condition, 120 days of growth): leaves (A); stems (B); roots (C). * Significant difference compared with the control, p < 0.05.
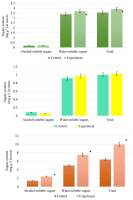
Our results show the differences in ascorbic acid (AsA) accumulation in the leaves, stems, and roots of S. viminalis under salinity (Figure 4). The highest content of AsA, similar to proline content, was found in stems, which was 79% higher than that in control plants. In leaves and roots, the content of AsA was slightly higher than that of the control by 13% and 20%, respectively.
Figure 4
Content of AsA in Salix viminalis under salinity condition (field condition, 120 days of growth). * Significant difference compared with the control, p < 0.05.
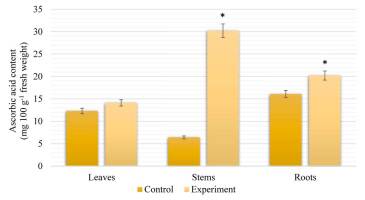
The response of antioxidant enzyme activity of S. viminalis plants to salinity is shown in Figure 5 and Figure 6. The highest catalase activity was observed in the roots of S. viminalis under salinity, which was 63% higher than that in the control plants. Nevertheless, the enzyme was less active in the leaves (14%) than in the control. In the stem, CAT activity was 48% higher than that in the control. Concurrently, salinity led to a 28% increase in POD activity in the stems (Figure 6). However, in the leaves, it showed the lowest peroxidase activity compared to the control. No significant difference was found in POD activity in the roots of S. viminalis under stress conditions.
. Discussion
Plants are routinely subjected to a combination of different abiotic stresses. Plant adaptation to environmental conditions primarily affects the growth process and functions of physiological and biochemical reactions (Vahdati & Lotfi, 2013). The responses of plants to combined stress are unique, and the responses to each stress cannot be applied individually. Overall, the stresses can be modified synergistically or antagonistically. Salinity interferes with plant growth as a result of both physiological drought and ion toxicity (Rosa et al., 2009). First, salinity affects the root system of plants and, as a result, plant development is inhibited by increasing salt concentrations in the soil (Zhou et al., 2013).
The leaves, stems, and roots of S. viminalis, which had been growing on experimental sites of the tailing at Stebnyk for 120 days, were inhibited in growth. This result is in agreement with previous studies, wherein tree species, including S. tetrasperma, were subjected to soil water deficit. Owing to the stress condition, morphometric parameters decreased significantly (Rasheed et al., 2021). Wang et al. (2013) reported that the growth of leaves, shoots, and lateral roots of S. matsudana, which were exposed to salt-stress conditions, was inhibited, whereas shoot height and lateral root length of Salix L0911 were greater than those of S. matsudana. In contrast to our findings, Hangs et al. (2011) suggested that willow plants can tolerate saline soils, having found no reduction in growth under salinity conditions.
Plant physiological and biochemical responses to multiple stresses at tailing sites may indicate their capacity to thrive under extreme environmental conditions (Gajić et al., 2018). Importantly, plants respond to abiotic stresses by accumulating compatible solutes, such as sugars, proline, and antioxidant enzymes, which are among the first and strongest responses to stress (Qaseem et al., 2021). Moreover, water- and alcohol-soluble sugars and amino acids accumulate in different plant species and function not only as osmolytes but also as antioxidants, helping in ROS detoxification and ultimately improving plant resistance to abiotic stress (Khaleghi et al., 2019). Nonenzymatic antioxidants are vital for plants because some highly toxic ROS, such as 1O2 and OH , cannot be scavenged by antioxidant enzymes; rather, plants rely on the nonenzymatic components of the antioxidant system to scavenge them (Akyol et al., 2020).
As expected, our experiments showed an increase in antioxidant activity in S. viminalis organs under stress conditions at Stebnyk tailing. The present study revealed that the stems of S. viminalis were exposed to the greatest salt load because stress caused a significant increase in proline accumulation. Our results are consistent with the findings of Babeanu and Dodocioiu (2018), who reported a higher content of proline in Swedish and Romanian clones of Salix grown on fly ash, which also contains potentially toxic elements such as heavy metals. High concentrations of proline contribute to the survival of plants under these conditions. Admittedly, the accumulation of free proline is an indicator of the intensity of stress and a factor that determines the ability to restore the organism of plants (Hernandez et al., 2000). In contraction with stems of willow, we found a decrease in the content of proline in the roots. This indicates the adaptation of roots to pollution during the development of the adaptive process. According to Ivashchenko (2013), this may be due to the lower water availability caused by physiological drought connected with salinity, which in turn increases the accumulation of proline. However, other studies have found that, due to soil salinity, accumulation of proline mainly occurs in plant leaves (Stolarska & Klimek, 2008). Our results differ considerably, whereas we found no substantial difference in the content of amino acid in the leaves under salinity. It can be argued that the content of proline may vary within different species and also within varieties of the same species (Bandurska, 2001). Therefore, the higher proline content found in plants exposed to severe and moderate stress conditions may have played an essential role in plant recovery after stress (Khaleghi et al., 2019).
Salt stress usually increases soluble sugars, mainly in the leaves and roots of many plants, and the most tolerant cultivars accumulate more soluble sugars (Song & Su, 2017). This is in complete agreement with our results, which showed a high level of soluble sugar accumulation in the leaves and roots of S. viminalis under salinity. Hence, this may indicate adaptive processes in plants. Our findings are in line with the results of Lu et al. (2021) as well as Song & Su (2017) who reported an increase in soluble sugars in the leaves of H. ammodendron and T. ramosissima with increasing NaCl concentration. The authors also noted an increase in the total sugar content in the in vitro culture of S. gracilistyla under salt stress. The accumulation of soluble sugars in the roots of S. viminalis under tailing conditions is a protective reaction of plants, which provides an increase in osmotic potential in cells. In addition, our results are confirmed by published data on the positive role of increasing the amount of sugar in plant tissues under the action of salinity and other stressors (Bilek et al., 2020; Nemati et al., 2011; Rosa et al., 2009).
Notably, we found a significantly high content of AsA in the stems of willow under stress conditions compared to other organs of S. viminalis. Our results are in agreement with those of other studies reporting increased AsA content in response to stress in woody plants (Kriger et al., 2013). The increase in AsA content may be due to the higher rate of ROS production (Karmakar et al., 2021). In addition, this may indicate plant resistance to pollution.
The antioxidant enzyme system is recognized as the main mechanism of plant tolerance to environmental stress (Alencar et al., 2021). To investigate the effect of stress conditions on the content of enzymatic antioxidants in the organs of S. viminalis, the enzymatic activities of catalase and peroxidase were determined. Catalase is among the most powerful mechanisms for ROS neutralization in cells (Jahantigh et al., 2016). Remarkably, CAT activity was the highest in the roots of willow plants under salinity as compared to the control plants. However, the present study demonstrates an increase in CAT activity in leaves under salinity. Our results are in agreement with those of Jahantigh et al. (2016), who defined a significant increase in total CAT activity in the leaves and roots of hyssop plants. According to Arsenov et al. (2017), in the presence of HM, CAT activity was significantly increased in the leaves of willow plants. Following this, the overexpression of antioxidant enzymes is an important tool for plant survival in conditionally elevated HM.
Peroxidase is widely distributed in higher plants and is involved in various processes, including salt tolerance and HM stress (Jahantigh et al., 2016). Peroxidase reactions in the cell are nonspecific responses of plant organisms to the action of stress factors, and the direction of changes in the activity of these enzymes indicates the state of adaptation of the organism and the level of free radical oxidation processes (Kolupaiev & Oboznyĭ, 2013). We found that enzyme activity was highest in the stems of S. viminalis, in contrast to other organs. However, other studies found significantly higher POD activity in the roots and leaves of S. matsudana under NaCl treatment (Li et al., 2018; Wang et al., 2013). Munawar et al. (2021) noted a significant increase in POD activity in cotton plants under salt stress conditions.
The present study clearly showed that the enzymatic and nonenzymatic antioxidants of S. viminalis were significantly affected under field conditions of Stebnyk’s tailing. In particular, we observed an increase in the activity of POD and the content of AsA and proline in stems under abiotic stress. This can be explained as the greatest salt load in the willow stems. In the roots, an increase in CAT activity and soluble sugar content was observed under salinity stress. Salix viminalis L. is a stress-tolerant plant with great antioxidant potential. The information obtained on enzyme activity and nonenzymatic antioxidant content in the organs of S. viminalis can further our understanding of plant tolerance mechanisms to Stebnyks’ tailing conditions, which will provide valuable and scientific information to use willow plants in nature-based solutions and eco-engineering projects on industrially polluted lands in Ukraine.
Our study has two limitations. The first is a relatively small number of replicates for each plot that was used for growing on the tailing. This can be explained by the harsh field conditions at Stebnyk tailing and the difficulty of collecting samples. Another important limitation is that we analyzed the influence of tailing salinity on growth and antioxidant content in organs of S. viminalis only after 120 days of growth. Thus, we cannot completely assert or analyze the conditions of plant growth and adaptation in the early stages of growth. Therefore, further data collection is needed to determine exactly the influence of the tailing on growth and physiological processes in S. viminalis under field conditions. Despite this, we believe our research could be a starting point for understanding the adaptive mechanisms of energy plants, namely S. viminalis, and trying to use them in nature-based solutions and eco-engineering projects on saline and industrially polluted lands in Ukraine.