. Introduction
The allelopathic effects of cover crop species are important during the germination and seedling growth (Cheng & Cheng, 2015; Shekoofa et al., 2020). The allelopathy phenomenon is understood as any influence of one plant on another plant through the release of chemical compounds (Jabran, 2017; Wu et al., 2020). Indeed, autoallelopathy or autotoxicity is a common problem in agriculture, which contributes to reduced yield (Singh et al., 2010). Autoallelopathic influence is intraspecific; chemical compounds of a plant inhibit or suppress the growth of another plant (Gaba et al., 2018; Oraon & Mondal, 2021; Singh et al., 2010). Allelopathy is a physiological and ecological mechanism influencing agricultural crop production (Farooq et al., 2020). Due to the favorable climatic conditions and socioeconomic importance of cereals in the human diet, the cultivation of winter cereals is quite common (Georgieva, 2019; Jalali et al., 2020). Thus, it is important to understand the allelopathic effects influencing the germination and seedling growth of winter cereals (El Beheiry et al., 2019; Sołtys et al., 2010).
Germination and seedling growth are important stages of plant growth (Korotkova et al., 2021; Shahrajabian et al., 2019). New strategies for managing organic production using allelopathy and autoallelopathy are emerging (Karami & Akhzari, 2020). Additionally, there is a lack of data on the influence of elevated CO2 levels on the autoallelopathic influence of cereals, specifically in light of the global greenhouse effect, hence this aspect was also considered in this study. The main purpose of this study was to assess the autoallelopathic potential of four cereals [winter wheat (Triticum aestivum L.), triticale (×Triticosecale Wittm.), spelt wheat (Triticum spelta L.), and barley (Hordeum vulgare L.)] under elevated CO2 conditions.
. Material and Methods
Plant Materials
The specimens of four cereal species: winter wheat (Triticum aestivum), triticale (×Triticosecale Wittm.), spelt wheat (Triticum spelta L.), and barley (Hordeum vulgare L.) at the growth phase of spike/panicle development, were obtained from the experimental station of the University of Agriculture in Krakow (located at Mydlniki-Krakow). The plant materials were air-dried in the shade at room temperature of 24 to 25 °C. The dried materials were then segregated partwise into stem, leaves and spikes/panicles. The separated parts were ground in an electric grinder and weighed on the balance (Radwag, Poland) up to two decimal places. The ground material was stored in plastic containers at room temperature until further use. The aqueous extracts having 0.5%, 1.0%, 2.0%, and 4.0% concentration of the dried plant material were prepared in glass flasks. For the preparation of extract, dry powder (0.25, 0.50, 1.00, and 2.00 g) of each type of cereal meal and 50 mL of distilled water was gently mixed in the flask covered with aluminum paper. The flasks containing the extracts were stored in a laboratory booth maintained at 21 °C. After 24 hr, the extracts were filtered by using a regular filter paper (Ahlstrom-Munksjö, Germany).
Germination Test
The glass Petri dishes (11 cm diameter) were cleaned with ethanol (99.5%) and sterilized in an oven at 105 °C for 1 hr to remove the dust and other impurities. A 5 mL volume of the aqueous extract concentration (0.5%, 1.0%, 2.0%, and 4.0%) of cereal was dispensed in a Petri dish in three replicates per concentration. Distilled water was used as control. Seeds of the four cereal species were surface sterilized with 5% ethanol and washed with 100 mL of tap water for 2 min, rinsed under tap water, and then 15 seeds were placed in each Petri dish on two layers of filter paper as seedbeds. The seeds of the cereal species used in the test were from the same sample batch as those used for the extract. To prevent dehydration, the Petri dishes were sealed with parafilm and then incubated at 21 °C in a laboratory booth, avoiding direct sunlight.
The potential allelopathic effects of the aqueous extracts were evaluated using the following growth parameters: germination rate, length of the shoot and root of the seedlings. The germination rate was assessed on the third day after seeding in the Petri dishes by counting the number of germinated seeds (having at least 1 mm of shoot) divided by the total number of seeds used per Petri dish and multiplying by 100. The shoot and root length of the seedlings was measured after 7 days of growth by using a millimeter scale. The length of the shoots was measured from the apex of the shoot to the bottom of the shoot, whereas the length of the roots was obtained by measuring the meristematic apex of the main root up to the lap region.
Pot Experiment in the CO2 Chamber
Pot experiment was conducted in a CO2 chamber at two concentrations of CO2 in the atmosphere, ambient and elevated (2,000 ppm). This experiment was performed in the laboratory of the Department of Agroecology and Crop Production, University of Agriculture in Krakow during the winter season of 2019/2020. The CO2 chamber was a 400-L top-closed container, equipped with an LED GX 300 (PhytoLite, Italy) lamp (288/192 W) and a CO2 source with a CO2 flow sensor NDIR (Omni, UK), operating during the light (day) phase. The photoperiod was 16 hr/8 hr, and the temperature range was 18–25 °C. Water was continuously provided in a closed cycle. Pots of 1 L volume were lined at the bottom with two layers of filter paper and filled with an adequate amount of sand (445 g) of 0.2 mm grain size (BIOVITA, Poland). Dry powder, a mixture of different parts of winter wheat (T. aestivum L.), triticale (×Triticosecale Wittm.), or spelt wheat (T. spelta L.), such as spikes, leaves, and stems, mixed in a 1:1:1 ratio, was added to the upper layer of sand (2.0 or 4.0 g of dry powder) per pot. Next, 15 seeds of cereal species were seeded approximately 2 cm deep into each pot. The control pots contained only sand. The setup was a completely randomized (CR) design with three replicates (pots) per treatment. Plant biomass was determined as the dry weight recorded up to two decimal places by using a balance (Radwag, Poland).
Analyses of Chemical Composition of the Cereals
Fluorescence analysis of phenols of the dried leaves, stem, and spikes from cereal plants was performed according to the method of Lichtenthaler and Babani (2004), by evaluating the fluorescence emission spectra of each sample recorded by using a spectrofluorimeter (Perkin-Elmer LS55B, UK), equipped with an attachment for measuring solids (the so-called Front Surface Accessory – 52123130). Grounded plant sample (2.0 g) was placed on one of the two quartz slides (1 cm in diameter) and covered with the second slide. The slides loaded with the sample were placed in the metal ring of the attachment 52123130. Fluorescence intensity measurements were performed in the range of blue-green light (430–650 nm), with 390 nm excitation, and near-and far-red light (650–800 nm), with 430 nm blue excitation. The gap for the excitation ray was 15 nm, and that for the emitted ray was 20 nm. The measurements were performed in replicates of five, and the results were collected and analyzed using the FL WinLab ver. 3.00.
. Results
Chemical Composition of the Plant Materials for Extracts
The highest concentration of chlorophyll was observed in the leaf extract and the lowest in the spike and the stem. The barley leaves and spelt wheat leaves had the highest chlorophyll content, and the chlorophyll content of winter wheat spikes and spelt wheat spikes was the least (Figure 1). Chemical analysis revealed that phenolic acids were the most predominant chemical compounds in all the cereals. Samples of barley, especially barley spikes were the richest in phenolic compounds. Interestingly, there were phenolic acids were not detected in the spike samples of both wheat and triticale (Figure 2).
Figure 1
Relative content (ratio of emission 690/735) of chlorophyll in cereals’ extracts. The lower bar is presenting higher chlorophyll content. Legend: BL – barley leaf; BST – barley stem; BSP – barley spike) SWL – spelt wheat leaf; SWSP – spelt wheat spike; SWST – spelt wheat stem; TL – triticale leaf; TSP – triticale spike; TST – triticale stem; WWL – winter wheat leaf; WWSP – winter wheat spike; WWST – winter wheat stem.
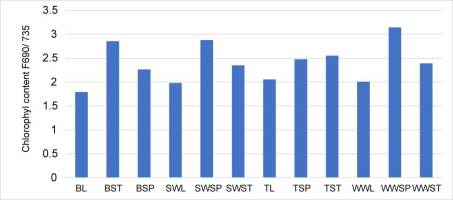
Figure 2
Relative content (ratio of emission F440/530) of phenolic acids in cereals’ extracts. Legend: BL – barley leaf; BST – barley stem; BSP – barley spike; SWL – spelt wheat leaf; SWSP – spelt wheat spike; SWST – spelt wheat stem; TL – triticale leaf; TSP – triticale spike; TST – triticale stem; WWL – winter wheat leaf; WWSP – winter wheat spike; WWST – winter wheat stem.
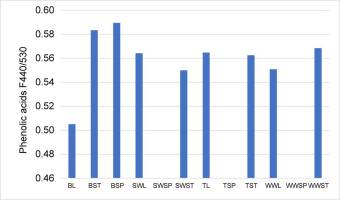
Germination Test
The germination rate was significantly influenced by the treatments of the water extracts. The highest germination rate was observed in the control and the lowest in presence of 4.0% extract. The germination rate of spelt wheat treated with the highest concentration of spelt wheat extract was 98% less than that of the control (Figure 3A).
The shoot and root lengths of spelt wheat were significantly influenced by the water extracts. The maximum reduction in the shoot length of spelt wheat seedlings (98.7% less than the control) was observed in the presence of the highest concentration of the spelt wheat extract (Figure 3B). The maximum reduction in the root length of spelt wheat seedlings (98.2% less than the control) was observed in presence of the highest concentration of spelt wheat extract (Figure 3C).
Figure 3
Germination rate, shoot and root length of spelt wheat seedling after water extracts treatment. (A) Germination rate after seed treatment with water extracts. (B) Mean length of shoot for concentration. (C) Mean length of root for concentration, the differences between treatments are significant. Legend: 0% – control; 0.5% – 0.25 g; 1% – 0.5 g; 2% – 1 g; 4% – 2 g.
* Mean values are shown with whiskers that represent standard error (SE). Differences between means are shown with asterisks in comparison to control (p < 0.05), according to Tukey test (α = 0.05).
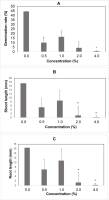
Shoot and root lengths of winter wheat were significantly influenced by the type of water extract used. Winter wheat seedlings germinated poorly in presence of the stem extracts (Figure 4A). In contrast, significantly longer shoots were observed in the presence of the leaf extracts (Figure 4B), and the root length was reduced in the presence of the stem extracts (Figure 4C). The highest concentration of barley extract reduced the germination rate of barley by 31.4% as compared to the control (Figure 5A). The shoot and root lengths of barley were significantly influenced by the water extracts. The maximum reduction in the shoot length of barley seedlings was seen in presence of the highest concentration of the barley extracts, 48.5% less as compared to the control (Figure 5B), while the maximum reduction in the root length of barley seedlings observed in presence of the highest concentration of barley extracts was 51.2% less than that of the control (Figure 5C). The shoot length of triticale was significantly influenced by the water extract. The maximum reduction in the shoot length of triticale seedlings observed at the highest concentration of triticale extract was 69.7% less than the control (Figure 6A). Root length was affected by the water extracts and the maximum reduction of the root length of triticale seedlings was observed at the highest concentration of triticale extracts, which was 23% lower than that of the control (Figure 6B).
Figure 4
Germination rate, shoot and root length of winter wheat seedling after type of extracts treatment. (A) Germination rate after seed treatment with type of extracts. (B) Mean length of shoot for extracts. (C) Mean length of root for extracts, the differences between treatments are significant. Legend: swsp – spelt wheat spike; swl – spelt wheat leaf; swst – spelt wheat stem.
* Mean values are shown with whiskers that represent standard error (SE). Differences between means are shown with asterisks in comparison to control (p < 0.05), according to Tukey test (α = 0.05).
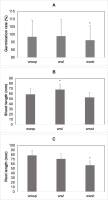
Figure 5
Germination rate, shoot and root length of barley seedling after water extracts treatment. (A) Germination rate after seed treatment with water extracts. (B) Mean length of shoot for concentration. (C) Mean length of root for concentration, the differences between treatments are significant. Legend: 0% – control; 0.5% – 0.25 g; 1% – 0.5 g; 2% – 1 g; 4% – 2 g.
* Mean values are shown with whiskers that represent standard error (SE). Differences between means are shown with asterisks in comparison to control (p < 0.05), according to Tukey test (α = 0.05).
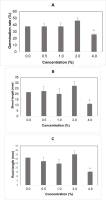
Figure 6
Shoot and root length of triticale seedling after water extracts treatment. (A) Mean length of shoot for concentration. (B) Mean length of root for concentration, the differences between treatments are significant. Legend: 0% – control; 0.5% – 0.25 g; 1% – 0.5 g; 2% – 1 g; 4% – 2 g.
* Mean values are shown with whiskers that represent standard error (SE). Differences between means are shown with asterisks in comparison to control (p < 0.05), according to Tukey test (α = 0.05).
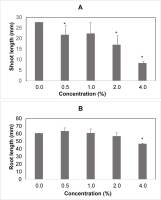
Seedling Growth Test-Pot Experiment in the CO2 Chamber
Effect of Elevated CO2 and Powdered Winter Wheat on Winter Wheat
On an average, the mean dry weight of the shoot of winter wheat was lower at an elevated level of CO2 compared to the ambient level. At the same time, the mean dry weight of root was the same for both the CO2 treatments, whereas the mean length of the shoot and root was greater in the elevated CO2 treatment (Table 1). A detailed analysis of the effect of dry powders on the growth of winter wheat revealed that, in comparison to the control, at the ambient CO2 level, the addition of powders significantly stimulated biomass accumulation in both shoots and the roots, without any effect on plant height (Table 1). At elevated CO2 concentration, the effects of the powders were unclear. At a lower dose of powder, the biomass of both the shoot and root of winter wheat was either higher or same as the control; however, the length of the shoot and root was significantly lower than that of the control. In the case of higher powder dose (4.0 g), the biomass accumulation was significantly lower but increase in plant height was significant (Table 1).
Table 1
The effect of elevated CO2 on growth and dry mass accumulation of winter wheat growing in sand mixed with a 2 or 4 g of a powdered winter wheat in the growth phase of earing.
Effect of Elevated CO2 and Powdered Spelt Wheat on Winter Wheat
On an average, at elevated CO2 concentration, a significant increase in dry weight of root, and shoot and root lengths of winter wheat was observed compared to the values at ambient CO2 concentration (Table 2). At ambient CO2 concentration, the addition of spelt wheat powder to the sand caused a significant increase in shoot and root dry weight compared to the control plants. The height of the plants was unaffected by change in CO2. In the elevated CO2 condition, addition of spelt wheat powders caused a significant increase only in the winter wheat root dry weight. All other traits were unaffected (Table 2).
Table 2
The effect of elevated CO2 on growth and dry mass accumulation of winter wheat growing in sand mixed with a 2 or 4 g of powdered spelt wheat in the growth phase of earing.
Effect of Elevated CO2 and Powdered Triticale on Winter Wheat
On average, elevated levels of CO2 increased the root dry weight in winter wheat, as well as the length of roots and shoots, compared to the ambient conditions. On an average, the control plants of winter wheat were taller and with higher biomass of shoots and roots at elevated levels of CO2 than the control plants under ambient CO2 conditions (Table 3). Under ambient CO2 conditions, the addition of triticale powder to the sand caused a significant increase in winter wheat shoots only in comparison to the untreated control (Table 3). Under elevated CO2 conditions, in the presence of 2.0 g of triticale powder, a significant increase in winter wheat shoot length was noted, with shoot dry weight remaining same as that of the control plants. Addition of 4.0 g of powder resulted in a significant increase in root dry weight and root length compared to the untreated control plants (Table 3).
Table 3
The effect of elevated CO2 on growth and dry mass accumulation of winter wheat growing in sand mixed with a 2 or 4 g of a powdered triticale in the growth phase of earing.
. Discussion
Chemical Composition of the Plant Material for Extracts
The identification of chemical components of the plant material in the water extracts is important to understand the effect on the target plants (Ahmad et al., 2020). Chemical analysis revealed that the chlorophyll contents in the cereal (donors of allelopathic compounds) were different. Leaves of the cereals contained the highest concentration of chlorophyll, and spikes and stems the lowest. Barley and spelt wheat had higher chlorophyll content than the other cereals. Physiological factors affect the chemical composition of plants, such as the chlorophyll content of crops as reported by Sid′ko et al. (2017). Chemical analysis also showed that ferulic acid was the most predominant type of phenolic compound in all the four cereal species. Boutigny et al. (2008) mentioned that in cereals, phenolic compounds represent an important group of secondary metabolites besides carotenoids and tocopherols. However, research on the presence of phenolic compounds in cereals has shown that their composition depends on the cereal species or varieties (Ndolo & Beta, 2014; Pihlava et al., 2015).
Germination Rate
Drought enhances allelopathic effects on the seed germination and seedling growth (Wu et al., 2020). The allelopathic evaluation in our study showed that the four cereal species responded to the application of different concentrations of water extracts, as seen by the considerable effects on the seed germination. The different concentrations of aqueous water extracts significantly suppressed the germination rate of spelt wheat, followed by barley; though the germination rates of triticale and winter wheat were also reduced, the difference from the control was not significant (p > 0.05). Therefore, spelt wheat and barley appear to be more sensitive to the allelopathic effects of water extracts. In general, the concentrations of 1.0 and 2.0 g caused the maximum inhibition of the germination rate for all cereals studied. The effect of several concentrations of water extracts on plant growth has been explored, and higher concentrations are known to inhibit sprouting (Ahmed et al., 2008).
The extent of inhibitory effects of water extracts from the tissues of spelt wheat, winter wheat, barley, and triticale on the germination rate depended on the type of extract and the target seed species. The germination rate of winter wheat was found to be the most sensitive among the cereals when subjected to autoallelopathy stress, and differences in the effects may be due to the different crop characteristics. The water extract from the stems of winter wheat showed a greater inhibitory effect on winter wheat seed germination than did the extract from leaves and spikes. Moreover, the water extracts of the other cereals showed an inhibitory effect on their seed germination, excluding the differences among treatments, which were not statistically significant (p > 0.05).
Shoot and Root Length
Allelopathic interactions accounting for the inhibitory effect of shoots on wheat growth in dense clay subsoils have been recorded (Wang et al., 2019). The plant parts showed an inhibitory effect on root and shoot growth. Statistical analyses showed that the maximum reduction observed at the highest concentration had a significant effect on the shoot and root length of spelt wheat, barley, and triticale. However, in winter wheat, we did not record significant differences, even though the shoot and root lengths of the seedlings were inhibited. From the study on cereals in relation to the source of the extracts, the statistical analysis revealed that the different parts (leaf and stem) had a significant effect on the shoot and root length of winter wheat (p < 0.05). The effects on the other cereal species were not statistically different (p > 0.05). Shoot and root length of cereals spelt wheat, barley, and triticale were more sensitive to the highest concentration of aqueous extracts. While for the source of extracts, the results suggest that winter wheat leaf extract might improve shoot length and stem extracts might reduce root length.
In general, the effects of different concentrations and types of extracts are indispensable factors affecting the shoot and root length of winter cereals. The findings demonstrated that such effects might be associated with autoallelopathic influence due to the presence of secondary metabolites (ferulic acids) in the crops used in the study. Thus, the results support the explanation that the negative effect of autoallelopathy phenomenon due to secondary metabolites released from maternal plants could hinder and inhibit directly or indirectly the growth of their seedlings (Chomel et al., 2016; Fernandez et al., 2016). Furthermore, reports provided by Amb and Ahluwalia (2016) explained that the inhibitory effect of autoallelopathy in some cereals is caused by both living and dead plants, which directly or indirectly affect the growth of the plants.
Plant Biomass
The effect of CO2 levels and the source of cereal powder used influenced the growth parameters of winter wheat, such as the shoot and root dry weight, as well as shoot and root length. Statistical analysis of the results revealed significant differences among the treatments (p < 0.05). The dry weight of the shoots and roots was significantly altered by elevated CO2 in presence of the cereal powders, with a higher biomass accumulation in the roots at 2.0 and 4.0 g of powdered spelt wheat and triticale. The longest shoots and roots of winter wheat were observed under elevated CO2 at 2.0 g of powered triticale. The results of the study showed that the effect of elevated CO2 combined with winter wheat dry powder on the initial growth of winter wheat resulted in an increase in shoot and root length and decrease in shoot and root dry weight. Elevated CO2 combined with spelt wheat powder decreased shoot dry weight and increased root dry weight in winter wheat. Elevated CO2 associated with triticale powder increased the shoot and root length, and root dry weight but decreased the dry weight of the shoot of winter wheat.
These results are consistent with previous studies (Geiger et al., 1999), which reported that the pattern of growth and development of plants subjected to different CO2 concentrations are different among species. Indeed, some studies have reported the effects of elevated CO2 on plant growth and development, and several studies demonstrated that, in general, elevated CO2 increases root dry mass (Reddy et al., 2010; Seneweera, 2011). Furthermore, Rogers et al. (1995), based on 264 observations, reported that elevated CO2 causes several responses, where the positive responses on shoots and roots are 59.5% higher, as compared to 37.5% negative response, and 3.0% without any response. Interestingly, our results showed significant statistical differences in the biomass accumulation in the shoots and roots of powdered winter wheat grown under elevated CO2. Effects on shoot and root length were observed and the shortest length was recorded in presence of 2.0 g of powdered winter wheat. These results might be related to the autoallelopathy influence of powdered cereals, as this phenomenon is observed among plants of the same species that release autotoxins from sources such as plant residues, root exudates, leachates of plants, and volatile oils (Yu, 2001).
According to Fatholahi et al. (2020), seed germination and root elongation are the primary effects of autoallelopathic influence. Furthermore, the influence on germination and roots is manifested as dwarf plants and lesions on the tap and lateral roots. In addition, the presented results are supported by studies that have demonstrated that autoallelopathy phenomenon inhibits plant growth in two main ways: direct (affecting photosynthesis and plant defense systems) and indirect (affecting nutrient absorption) (Ahmed et al., 2008; Feng et al., 2019; Mitić et al., 2018; Wardle et al., 2004; Yang et al., 2010).
In the present study, winter wheat responded to the allelopathic effects when elevated CO2 was combined with dry cereal powder in pot experiments. Our results confirm higher reduction in shoot dry weight than in root dry weight, while beneficial effects (e.g., increased shoot and root length) were also observed.
. Conclusions
Ferulic acid was identified as the main phenolic compound in all the four cereals selected for this study. The highest concentration of chlorophyll was observed in the leaves of barley and spelt wheat. The autoallelopathic effects of the cereals are confirmed by the results of the experimental studies. The highest concentrations of water extracts (2.0% and 4.0%) reduced the germination rate of spelt wheat and barley, as well as the shoot and root lengths of spelt wheat, barley, and triticale seedlings. Among the sources of extracts, the stem extracts negatively affected root length, and the leaf extracts positively affected shoot length; both effects were observed in winter wheat. The combination of elevated CO2 level with spelt wheat powder or triticale powder decreased shoot dry weight but increased the root dry weight and shoot and root length of winter wheat. The elevated level of CO2 combined with winter wheat powder decreased shoot and root dry weight; however, the shoot and root length in winter wheat was increased.