. Introduction
One of the problems of citrus farmers, including lime farmers, is the high cost of maintenance, especially pruning, which reaches 10–30% of production costs (Goldental-Cohen et al., 2017). Based on the results reported by Martí and González (2010), manual pruning of citrus trees costs 979.67 EUR/ha. A research report by Martin-Gorriz et al. (2021) demonstrated that the manual pruning treatment required an average pruning time of 81 h/ha each year from 2016 to 2019, which was higher than in the mechanical pruning practice. Similarly, in terms of cost, manual pruning costs an average of 807 EUR/ha annually, higher than mechanical pruning. Pruning aims to optimize the radiation received by the plant, in addition to optimizing branch growth, so there are production pruning and maintenance pruning. Radiation is essential in citrus growth and production, especially for flower initiation, blooming, and fruit formation (Abobatta, 2018). Pruning branches make the plant canopy broader and more regular so that every part of the plant gets optimal light intensity. Pruned citrus plants have 85% more flowers, 76% more fruit, and 105.3 g more flesh than trees without pruning (Sugiyatno et al., 2019). To overcome this problem, a cultivation technique that simplifies the process of pruning citrus plants is needed (Martin-Gorriz et al., 2021). Therefore, there are significant efforts to reduce the cost of pruning.
The abscission of plant organs such as leaves, fruits, and flowers occur naturally if they are mature or unnecessary for the plant. Self-pruning is a type of abscission that occurs at the tip of the shoot and the branch. As shown by Paisey et al. (2022), self-pruning naturally occurs in tropical fruit plants, including tamarind, avocado, kapulasan, longan, guava, orange, and lime. It has been observed in oranges, chestnuts, and tomatoes (Jiang et al., 2008; Plummer et al., 1991; Zhang et al., 2014). In tomatoes, the self-pruning gene affects the tomato growth habit at least in part by influencing auxin transport and responsiveness, which can be manipulated for controlling plant growth habit and improving productivity (Silva et al., 2018). Self-pruning is also found in grapevines; it can increase horticultural production efficiency if utilized effectively to reduce manual pruning (Zou et al., 2020). In citrus plants, self-pruning occurs in lateral shoots, which begin to collect nutrients for flower bud differentiation (Zhang et al., 2014). Branch shedding is usually connected with an adaptation to environmental stresses, such as shading and drought, mediated by a decreased hydraulic conductance (Bellani & Bottacci, 1995; Davis et al., 2002; Lauri et al., 2011; Minemba et al., 2019).
Self-pruning can occur if induced by genetic or environmental factors. The hormones ethylene and abscisic acid play an essential role in self-pruning. Ethylene compounds are affected by nutrients, as shown by Kaack and Pedersen (2014), who performed factor analysis of data from an experiment with fertilization of ‘Spartan’ apples using various levels of nitrogen, phosphorus, and potassium. Their interactions exhibited significance between ethylene and potassium, soluble solids and potassium, acidity and phosphorus, ethylene and phosphorus, and ethylene and nitrogen. Ethylene response is highly dependent on the availability of mineral nutrients, and potassium deficiency can increase ethylene production and reduce stomatal response (Benlloch-González et al., 2010). Nitrogen availability also affects photosynthesis, stomatal conduction, and ethylene production. In addition, the synthesis of ABA was influenced by N, P, and K fertilization, which was proved in Camptotheca acuminata plants, where fertilization with N, P, and K (50 g/plant with the N, P, and K ratio of 19:7:12) resulted in a significant increase in the amount of ABA (Liu et al., 1999). Self-pruning can be used as an alternative to pruning citrus plants to reduce production costs. Therefore, it is crucial to investigate the effect of fertilization and growth regulators on self-pruning in lime plants. Many trees prune themselves better than others. In the case of lime plants, self-pruning has not been studied; hence, it is essential to investigate this phenomenon. The study aimed to determine self-pruning incidence with a morphological and biochemical approach to lime plants treated with growth regulators and NPK fertilizers.
. Materials and methods
The research was conducted at the Experimental Garden of IPB Leuwikopo Dramaga (Latitude: −6.564508, longitude: 106.725009), Bogor–Indonesia from February 2020 to April 2022. The study was conducted under a 50 m long, 5 m wide, and 3 m high shade house. The average daily climate conditions during the study (February 2020–April 2022) were as follows: temperature 26.13 °C, RH (Relative Humidity) 83.01%, light intensity radiation 349.08 µmol m−2 s−1, duration of sunshine 13.67 h/d, 11.82 mm/d rainfall.
The study was conducted in a randomized block design. The first factor was the NPK dose (F) and the second factor was growth regulators (Z). Inorganic fertilizer was used at three doses: 22.5:7.5:2.5 g/tree (F1), 32.5:17.5:12.5 g/tree (F2), and 42.5:27.5:22.5 g/tree (F3). The variants of growth regulators were as follows: 500 ppm ichiphon + 100 M ABA (Z1), 750 ppm ichiphon + 50 M ABA (Z2), and 1000 ppm ichiphon (Z3). The treatment was repeated three times, each repetition consisting of 3 plants so that there were 81 individual plants.
The plant material included local lime seedlings obtained from propagation between a rootstock derived from Rough lemon (Citrus jambhiri Lush.) and a scion derived from lime (Citrus aurantifolia Swingle). The plant material was planted in a mixture of wet rice husk, dry rice husk, and chicken manure with a ratio of 1:1:1, which was maintained for six months. Lime plant seeds were transferred into polybags when they were one year old. The media used was a mixture of clay soil, chicken manure, and rice husks with a ratio of 3:2:1 (37.5:25:12.5) in a 75-liter volume planter. ICHIPHON 480 SL (RI 01040120041974/petrosida gresik) and abscisic acid C15H20O4 (PhytoTech labs HHY0102072A) were used as growth regulators. ABA purity was min 95% according to manufacture description. Ichiphon 480 SL contained 480 g/L ethephon (C2H6ClO3P).
The fertilization treatment was conducted two times a year in February and September when the plants were 12 and 19 months old. The fertilizer was applied by creating circular arrays in the root area at a depth of 5 cm; then the fertilizer was spread in the array and re-covered with the planting medium. The ichiphon and ABA spraying treatments were applied only once on 1st July 2021 or when the lime plant was 18 months old. Ichiphon was sprayed on citrus plants and then covered with plastic to reduce the evaporation of ichiphon into the air, whereas abscisic acid was applied to the root area. Observations were made for 9 months after the treatment.
Lime plant maintenance included weeding and spraying with the sidamethrin insecticide against ladybugs and leaf caterpillars. The sidamethrin insecticide contains the active compound cypermethrin (R.S-alpha-cyano-3-phenoxybenzyl (1R.1S), trans-3 (2.2)-dimethylcyclopropanecarboxlyic acid) with the concentration of the active compound of 50 g/L, which is a systemic insecticide that works as a stomach poison. Sidamethrin was given 3 months before the treatment at a dose of 1 mL/L. Spraying was performed when pests attacked the plants. Watering was done every morning and evening when it did not rain. Watering was carried out until the soil moisture reached 80%, as measured by a soil pH-moisture meter (VT05) from China.
Percentage of self-pruning on secondary branches, tertiary branches, and leaves and morphology of branches
The percentage of self-pruning indicates branches and leaves that experience self-pruning. Dead and dry branches remaining on the tree are also known as self-pruning events. Morphological measurements of dead secondary and tertiary branches were conducted by measuring the stem diameter with an electronic digital caliper Sketmat Sigmat Vernier 0–150 mm (Nankai, China). Illustration of lime tree branching is presented in Figure 1. The length of the branches was measured from their base to the tip using a tape measure or a ruler. The number of dead secondary branches was observed and counted from the treatment until four months after the treatment by a hand counter (Kenko, Japan). The number of tertiary branches that died was observed and counted from the time of treatment until four months after the treatment. Self-pruning of leaves was counted every day after the plants were given the treatment. The calculations were conducted until the plants no longer dropped their leaves.
Analysis of ethylene levels
Ethylene analysis was conducted on samples of leaves that dropped on the first day to the 12th day after the treatment. It was conducted using gas chromatography. The sample was homogenized, and then 1 g was weighed and put into a 10-ml tube. The tube was closed with a rubber cover; then the gas was taken in a 1-ml tube. Acetylene gas was injected into the tube with the same volume, and then incubated for 1–2 h. The gas in the sample was injected into a Hitachi gas chromatograph type 263-50, with an initial temperature of 80 °C, final temperature of 100 °C, injection temperature of 150 °C, detector temperature of 200 °C, contents of silicon ov-17 column, a hydrogen and nitrogen carrier, and detector FID (Yu & Yang, 1979; McNair & Miller, 1998).
Analysis of abscisic acid levels
Abscisic acid was determined on the first day after the treatment and 12 days after the treatment with the procedure proposed by Yokota et al. (1994). A standard solution was prepared by weighing 0.0020 g of abscisic acid into a 5-ml volumetric flask and adding the eluent to the mark. The stock standard was 40 ppm. That standard solution was made of a standard mixture of abscisic acid with a concentration of 12.5, 25, 50, 100, 250, and 1000 ppb. About 1 g of lime leaves was finely chopped, and 25 ml of 6.8 mH H3PO3 methanol was added. 25 mg BHT/500 was added to methanol and the pH was set to 8.5 by adding 1 M NaHCO3. The solution was evaporated at a temperature of 35 °C so that the liquid phase remained. The solution phase was purified with a seppak column with C18, then added to 10 or 25 ml with the mobile phase, and filtered with 0.45 µM. The solution was ready to be injected into HPLC. The HPLC specifications were as follows: column C18 eluent H3PO4 68 mM:MeOH (50:50), flow 0.5 ml/min, PDA detector, and a wavelength of 260 nm. The samples were analyzed at 1 and 12 days after treatment.
Chlorophyll analysis
Chlorophyll content was analyzed 14 days after the treatment using the Warren (2008) method. Fresh leaf discs (0.56 cm2) were ground in a mortar. Chlorophylls were extracted from the ground samples by adding 1.00 mL of methanol and shaking for two minutes at 30 Hz. The samples were centrifuged for 2 min at 16873 g (Micro centrifuge 5418, Eppendorf, Hamburg, Germany) and the supernatant was transferred to another micro centrifuge tube. The pellet was re-extracted from a second 1.00 mL aliquot of methanol by adding 1.00 mL of methanol to the pellet, shaking for another two minutes, centrifuging, and removing the supernatant. The pellet was discarded while the two supernatants were pooled and used for measurement of chlorophylls.
Micro plate measurements were generally made with the lid on the micro plate so as to reduce evaporation. Spectrophotometer measurements were made by transferring 200 µL of the sample (or blank) into a 1-cm path length quartz cell and reading absorbance in a spectrophotometer with 1 nm bandwidth (UV-2550, Shimadzu, Kyoto, Japan).
The absorbance of 200 µL of the sample in a micro plate (A652 micro plate, A665 microplate) was converted into a 1-cm path length corrected absorbance using the measured path length:
A652, 1 cm = (A652, microplate − blank)/path length
A665, 1 cm = (A665, microplate − blank)/path length
The chlorophyll concentration was calculated from a 1-cm corrected path length:
Chl a (µg/mL) = −8.0962 A652, 1 cm + 16.5169 A665, 1 cm
Chl b (µg/mL) = 27.4405 A652, 1 cm − 12.1688 A665, 1 cm.
. Results
Self-pruning of leaves, and secondary and tertiary branches
Based on the results of the observations, the treatment caused self-pruning in secondary and tertiary branches. Thinning at the secondary and tertiary branches resulted in even distribution of sunshine on the plant canopy (Figure 2). The average percentage of the incidence of self-pruning on secondary branches of lime plants receiving ichiphon and abscisic acid and fertilization treatment was about 6.70%, which was higher than the self-pruning of tertiary branches (only 5.17%). Based on the results of the further DMRT test, the fertilization factors and regulatory substances applied to the lime plants significantly affected the mortality of tertiary branches (Table 1). Secondary and tertiary branches experiencing self-pruning were located at the bottom of the canopy; hence, they were shaded by the top branches. Due to this condition, the leaves were exposed to lower intensity of radiation to conduct photosynthesis, causing the branches to become weak.
Table 1
Percentage of self-pruning branches and leaves.
As shown by the results of further tests on the percentage of self-pruning of leaves (Table 1) due to the fertilizer application and PGR, a higher percentage of leaves were found in the F1 and Z3 treatments, but it was not significantly different from the F2Z3 and F3Z3 treatments. These treatments included fertilization below the standard and ichiphon growth regulators of 1000 ppm without ABA.
Morphology of self-pruning secondary and tertiary branches
Based on the DMRT test results, there was no significant difference in the morphological characters of the diameter and length of secondary and tertiary branches after the treatment (Table 2). The results showed that, after the treatment with ichiphon and abscisic acid at various doses and the fertilizer, the secondary branches had on average a diameter of 1.72 mm and an average length of 10.03 cm to die. Smaller branches are unable to survive the loss of leaves as a source of photosynthesis due to their lesser size than the others, which causes these branches to die. This also occurred in tertiary branches with an average diameter of 1.55 mm and a length of 9.98 cm (Table 2). Death only occurred in the case of branches with a diameter of less than 2 mm; in addition, the stem was still juvenile. Self-pruning began with the fall of leaves on the branches the day after the treatment. The assimilation needed by the lime plant branches became unavailable. After four months, the branch died, which was indicated by a change in the color of the branch from green to blackish brown, and then the branch dried up and broke quickly. This caused secondary and tertiary branches to die slowly.
Table 2
Size of self-pruning branches.
Endogenous ethylene and ABA hormones
Based on the DMRT test results on the concentration of ethylene hormone due to the fertilization treatment and the concentration of ichiphon and ABA (Table 3, Table 4), the highest average concentration was found in combination F2 (32.5:17.5:12.5 g/tree) and Z2 (750 ppm and 50 µM ABA) from the first to the 12th day after the treatment. The highest ethylene content was found in F2Z2 on the third day (126.44 ppm). In all treatments, there was an increase in the ethylene concentration on the second, third, and fourth days except for the F1Z1, F2Z2, F2Z3, F3Z2, and F3Z3 treatments, which exhibited a decrease in the ethylene concentration on the fourth day. The fertilization recommendation given by the Indonesian Subtropical Fruit and Citrus Research Institute (BALITJESTRO) was implemented in the F2 treatment, causing self-pruning events in addition to the administration of the growth regulators. Moreover, the lowest hormone concentrations were found in all treatments on the 12th day.
Table 3
Ethylene concentration (ppm) on the days after application.
Table 4
ABA concentration (ppm) on the days after application.
Based on the results of the further DMRT test, it was found that the application of the fertilizers and growth regulators produced a difference between the treatments: the highest ABA concentration was found in the F3Z1 (10.67 ppm) variant on the first day after the treatment. Meanwhile, the highest concentration of ABA was found in the F3Z3 treatment (5.94 ppm) on the fourth day, but it was not significantly different from that detected in the F3Z1 treatment (5.68 ppm). On day 12, there was a decrease in the ABA concentration in all treatments. The lowest ABA concentration was found in the F1Z3 treatment (0.58 ppm), but it was not significantly different from that in F2Z2 (0.75 ppm).
Stomatal conductance
There was an increase in stomatal conductance in all treatments compared to the controls (Figure 3B). Although there was no significant difference between the treatment with ethylene hormone, abscisic acid, and fertilization, the highest stomatal conductance was found in treatment F3Z2 (0.55 mol H2O m−2 s−1).
Figure 3
(A) Photosynthesis rate. (B) Stomatal conductance. (C) Transpiration 5 months after treatment. Fertilizer combinations (nitrogen, phosphorus, potassium): F1 (32.5:17.5:12.5) g/tree; F2 (22.5:7.5:2.5) g/tree; F3 (42.5:27.5:22.5) g/tree and growth regulators (Z): Z1 (500 ppm ichiphon + 100 µM ABA); Z2 (750 ppm ichiphon + 50 µM ABA), and Z3 (1000 ppm ichiphon).
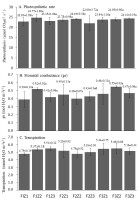
Chlorophyll a and b
The observation of chlorophyll a after the treatment showed its highest amount (166.08 mg/g) in the experiments using fertilizers above the recommendation from BALITJESTRO (F3) accompanied by 500 ppm ichiphon spray and 100 µM ABA, compared to the F2Z1 treatment (77.60 mg/g). However, the result was not significantly different from the other treatments (Table 5). In turn, the highest content of chlorophyll b was found in the F3Z1 treatment (96.99 mg/g), but it was not significantly different from the other variants. There was a significant difference in total chlorophyll caused by the F3Z1 (263.07 mg/g) and F2Z1 (124.18 mg/g) treatments. However, there was no difference between both treatments and the other treatments.
Table 5
Chlorophyll a, b, and total in leaves at 7 days after application.
Rate of photosynthesis
The highest photosynthetic rate, i.e. 24.77 µmol CO2 m−2 s−1, was found in treatment F1Z2 (22.5:7.5:2.5) g/tree and 750 ppm ichiphon + 50 M ABA, and the lowest value was noted in F2Z3 (22 µmol CO2 m−2 s−1) (Figure 3A). Nonetheless, there was no significant difference in the average rate of photosynthesis among the treatments.
Transpiration
There was no difference in transpiration among the lime trees subjected to the treatments. The lowest transpiration of 4.79 mmol H2O m−2 s−1 occurred in the F2Z2 treatment (32.5:17.5:12.5 g/tree and 750 ppm ichiphon + 50 µM ABA) and the F1Z1 treatment (22.5:7.5:2.5 g/tree and 500 ppm ichiphon + 100 µM ABA). Meanwhile, the highest transpiration rate of 5.51 mmol H2O m−2 s−1 was recorded in the F1Z3 treatment (Figure 3C).
. Discussion
Self-pruning occurs not only through the abscission zone. Branches in lime plants that experience self-pruning persist on the parent tree for months until external factors such as wind, rain, and animals make them fall. The ethephon and abscisic acid applied did not form abscission zones on the branches but only on the lime leaves. This is consistent with the findings of previous studies (Ferrante & Francini, 2006; Goldental-Cohen et al., 2017) showing that abscission is referred to as the process of natural separation of leaves from the parent plant, which is induced by the hormone ethylene and abscisic acid. It is currently known that ethylene and ABA act as activators in plant organ abscission (Li et al., 2019). In olives, leaf abscission was accelerated by ABA or ethylene treatment, and the combination of ABA and ethylene treatments showed an effective additive effect in leaf abscission. Abscisic acid can accelerate abscission directly (Estornell et al., 2013; Taylor & Whitelaw, 2001), while some studies suggest that ABA promoting organ abscission depends on interactions with ethylene and auxin (Roberts et al., 2002; Wilmowicz et al., 2016; Dimitrova & Nacheva, 2021). Moreover, ABA can promote abscission through ethylene (Gómez-Cadenas et al., 2000). Ethylene is probably the main hormonal activator of abscission (Gómez-Cadenas et al., 1996; Gómez-Cadenas et al., 2000).
Fallen lime leaves left the branches bare and caused them to lose the source of photosynthate needed for growth (Figure 2). This condition caused the branches to die and dry out, and then they were exposed to wind, rain, or animals and became broken. In other words, the branches were led to a slow death. The death that occurred is called cladoptosis (Hearn, 2016). Leaves that self-prune generated abscission areas, specifically on the petiole and leaf blades. This finding is in accordance with Estornell’s report (2013) that abscission occurs at a specific position called the abscission zone (AZ). It can be divided into the following four main stages: AZ ontogeny, acquisition of competence of AZ cells to respond to abscission signals, activation of cell separation, and differentiation of the protective layer. Generally, abscission is a gradual process and is accompanied by cell wall degradation, which depends on the activity of certain cell wall hydrolases (Roberts et al., 2002). Homogalacturonan (HG), as the main pectin, is one of the main components of the primary cell wall and can be degraded by polygalacturonase (PG), which is important for organ abscission in many fruit plants (Atmodjo et al., 2013; Liljegren, 2012; Tan et al., 2013).
The fertilization treatments conducted twice during the experiment did not directly cause leaf fall, but the leaves were shed the day after the ethephon and abscisic acid treatments. Based on the field observations, the leaf fall was dominated by leaves located in the lower canopy shaded by the upper crown, and leaves that were young and small also experienced self-pruning. This is consistent with the findings reported by Raden (2009), i.e. if there are too many leaves, the lower leaves do not receive enough light for photosynthesis; hence, the leaves only function as sinks and do not function as sources. In addition, the self-pruning that occurred was due to external factors, such as soil fertility, as nutrients play an essential role in the formation of the hormone ethylene and abscisic acid. As reported by Iqbal et al. (2011) and Kaack and Pedersen (2014), low doses of potassium can cause endogenous ABA concentrations to decrease, and the effect of ethylene will decrease when plants experience nitrogen deficiency. Moreover, nitrogen, phosphorus, and potassium are positively correlated with endogenous ethylene in apple plants, and these minerals play a significant role in several biochemical reactions that occur in apple plants; therefore, this compound is essential.
High light intensity supports the occurrence of stomatal conductance of CO2, hence it has a considerable influence on the maximum photosynthetic rate. As indicated by Raden’s research report (2009), at low light intensity, there is almost no CO2 absorption because the rate of CO2 absorption through photosynthesis is lower than the rate of CO2 evolution from mitochondrial respiration. The plants given the treatment had high stomatal conductance, although it was not significantly different. According to Budiarto et al. (2019), stomatal conductance increases, and the concentration of CO2 in the chloroplasts also increases. Stomatal conductance in plants is also influenced by ambient temperature. As shown by Raden (2009), an increasing environmental temperature increases the rate of photosynthesis due to an increase in enzyme activity, which enhances the capacity for CO2 utilization. CO2 fixation is a reaction controlled by enzymes. It increases with enzyme activity due to increasing temperature until it reaches a temperature that causes denaturation of the enzymes.
The higher dose of ethephon and abscisic acid caused the degradation of the chlorophyll concentration, which was caused by the increase in enzymatic activity increasing the incidence of self-pruning in the lime plants. The findings reported by An et al. (2021) revealed that that MdABI5 works with its positive or negative interaction partners to regulate ABA-mediated leaf senescence in apple, in which it acts as a core regulator. As explained by Edgerton and Blanpied (1968) and Hu et al. (2021), strigolacton (SL) and ethylene interactively regulated leaf senescence, mainly by controlling chlorophyll degradation induced by darkness in perennial ryegrass.
The enhancement in photosynthesis by the ichiphon, abscisic acid, and fertilizer application was mediated through ethylene-induced changes. It was also associated with a higher level of intercellular CO2. As demonstrated by Edgerton and Blanpied (1968), ethephon is a direct source of ethylene release when applied to plants and elicits a response identical to that induced by ethylene gas. Ethephon application to plants grown with variable nitrogen, phosphor, and potassium levels induced stomatal and photosynthetic responses. In addition, there was an interaction between ethylene, N availability, and photosynthetic characteristics. Kumar et al. (2002) showed a strong positive correlation with 1-aminocyclopropane carboxylic acid synthase, a rate-limiting enzyme in ethylene biosynthesis. Nitrogen influences the quantity, structure, and composition of the photosynthetic apparatus. It also plays a crucial role in determining the plant’s photosynthetic capacity in natural and agricultural environments.
The changes in the photosynthetic rate, transpiration, and stomatal conductance indicated a response of the lime plants to the treatments. The more leaves the plant produces, the higher the transpiration rate. The transpiration rate is closely related to leaf area. As shown by Akmal et al. (2018), plants with larger leaves have a higher transpiration rate, in turn, according to Dulbari et al. (2018), the relationship between stomatal conductance and the ability of photosynthesis and transpiration is important aspect in relation to environment responses. Thus, lime plants still need to be studied further with consideration of some influencing factors, such as the greenness of leaves/chlorophyll content, water availability, and solar energy factors to better understanding on self-pruning incidents.
. Conclusions
Self-pruning events can occur in various treatment applications. The F1Z3 treatment resulted in the highest percentage of self-pruning in leaves (53.55%), which was not different from the other treatments except F3Z2 (30.67%), where the percentage was lower than in the other treatments. There was no significant difference in the self-pruning events of the secondary branches, whereas the F2Z3 treatment caused the highest percentage of self-pruning events in the tertiary branches (5.17%), which was significantly different from the F1Z2 treatment (1.63%), which was lower than the others. The combined treatment variant F2Z2 was characterized by the highest ethylene content and the combined F3Z1 treatment resulted in the highest abscisic acid content one day after the treatment. The highest ABA concentration was recorded in the F3Z3 treatment (5.94 ppm) on the fourth day, but it was not significantly different from the F3Z1 treatment (5.68 ppm), likewise on the 12th day. This proves that self-pruning can occur due to the induction of compounds that play a role in abscission.