. Introduction
The world population has risen rapidly over the last decades, accompanied by a constant increase in crop production and excessive pressure on agricultural lands (Singh et al., 2011). To meet the challenge of food production, farmers were compelled to apply additional chemical fertilizers and pesticides, particularly in the developing countries. The use of these chemicals turned out to be unavoidable to provide essential plant nutrients, improve crop quality, and thus expand agricultural production. However, over-fertilization displays many drawbacks such as environmental degradation and soil poverty (Ye et al., 2020) due to the stock of synthetic nutrients in the soil (e.g., accumulation of nitrate and phosphate in the water sources). Consequently, the generation of greenhouse gas and salinization occur. Synthetic products are responsible for destroying beneficial soil microorganisms considered as natural enemies of pests and, hence, strengthen the risk of pest resistance (Kalia & Gosal, 2011). Moreover, the exposure to pesticides and the presence of trace metals in fertilizers represent a serious threat to human health and the environment (Guo et al., 2020; Hussain et al., 2012), in agricultural zones.
In natural conditions, plants are associated with a complex rhizosphere microbiome which exerts highly beneficial effects on plant development. The use of soil microbionts (bacteria, fungi, etc.) known as plant growth-promoting microorganisms (PGPMs) in agriculture has recently gained popularity (Woo & Pepe, 2018) since they play a vital role in enhancing soil fertility and improve crop productivity (Vejan et al., 2016) as well as plant resistance to biotic (Enebe & Babalola, 2019) and abiotic stresses (Basu et al., 2021). They include a large variety of microorganisms including Actinomycetes, arbuscular mycorrhizal fungi, nitrogen-fixing bacteria, phosphate solubilizing microorganisms, and endophytic bacteria (Compant et al., 2019). Direct and indirect mechanisms underlie their ability to improve plant growth, e.g., stimulation of plant’s hormone production, amelioration of plant nutrient acquisition (Dellagi et al., 2020), and decomposition of organic residues. These microbes can establish synergistic and antagonistic interactions with native soil microorganisms that shift the equilibrium to improve soil quality (Finkel et al., 2017).
The use of single strain inoculants in the agricultural sector started earlier with proven plant-growth promoting potential (Nuti & Giovannetti, 2015), and varieties of PGPM have been commercialized, including the species Bacillus, Enterobacter, Rhizobium, and Azotobacter (Ortiz & Sansinenea, 2022). Despite the satisfactory performance of some microbes in specific trials, their efficacy under field conditions is often rapidly lost, due to the dynamic environment involving biotic and abiotic restraints (Khare & Arora, 2015; Król et al., 2019). Thus, a successful use of PGPM relies on their long-extended ability of survival and reproduction in the soil in addition to their compatibility and interaction with indigenous soil microorganisms (Martinez-Viveros et al., 2010). To reach this goal, recent studies focused on the effect of mixtures formed by multi strains of PGPM combined sometimes with non-microbial products. Currently, the application of mixed strains of beneficial microorganisms, so called microbial consortia (MC), seems to have a better efficacy on plant growth amelioration than single strains (Thomloudi et al., 2019; Vishwakarma et al., 2020). Compared with single strain inoculants, the MC product may offer greater flexibility towards environmental factors since they are composed of compatible microbial strains providing a full range of actions (root growth-promoting and/or P-solubilizing strains) (Bradacova et al., 2019). MC products can increase the nutrient uptake capacity and water use efficiency, provide turnover of organic matter to soil, and establish close relationships with the rhizosphere. Currently, the application of MC is the best alternative to agrochemicals since they are ecofriendly, inexpensive, renewable sources of soil nutrients and exert multiple plant growth promoting activities. Despite the advantages of MC compared to single strains inoculants (Nuti & Giovannetti, 2015; Ortiz & Sansinenea, 2022), the critical goal is still the selection of physiologically and ecologically compatible microorganisms that could be applied as a mixture into the soil while conserving a long shelf-life and a bio-stimulant effect. Another challenge consists in ensuring MC establishment and interaction with indigenous microflora in the soil as well as abiotic factors of the inoculated soil (Ortiz & Sansinenea, 2022). To enhance the consistency of PGPM in the field, innovative systems that can be used in practical ways are needed. In this sense, microbial populations originating from various rhizospheres, featuring beneficial associations and synergistic interactions, could represent a valid strategy even though the strains are less defined (Higa & Parr, 1994).
Over the Mediterranean rim countries, Lebanon is the major consumer of chemical fertilizers. The application of pesticides and chemical fertilizers increases each year in order to obtain the maximum crop yield. Subsequently, pesticides and heavy metals were detected in various samples of soils (El-Alam et al., 2018) and water in the largest Lebanese river Litani (Nehme et al., 2014) as well as the Lake of Qarraoun (Haydar et al., 2014a, 2014b). Moreover, the agricultural sector, economically important in this country, has been neglected for many years without a reliable strategy for food security (Skaf et al., 2019). Hence, the adoption of sustainable cultivation practices is imperative. The present study aimed to evaluate the potential of natural microbial consortia on the growth and production improvement in two crops (C. annuum L. and S. lycopersicum L.). A comparative efficiency evaluation of natural microbial consortia versus a chemical fertilizer was established under natural production conditions in the field.
. Material and methods
Microbial collection and preparation
The microbial collection was obtained starting from soil samples collected from fertile unpolluted forests in Lebanon, precisely from the rhizosphere soil of a field having wild fruit trees. After collection, the soil samples were mixed with distilled water (50 g of soil to 250 ml of distilled water) and placed on a selective medium without dilution. MRS medium with 3% of glucose was used for selection of Lactobacilli spp. and yeast with a pH 6 at 25 °C. For Azotobacter spp., the medium used was Azotobacter Agar Mannitol composed of 0.2 g/L ammonium sulfate, 0.8 g/L dipotassium hydrogen phosphate, 0.2 g/L MgSO4, 0.1 g/L CaSO4, 0.1 ml Mo solution (0.1 mg/ml), 1 ml FeSO4 (1 mg/ml), 20 g/L of mannitol, and 15 g/L of Agar, pH adjusted to 7 at 25 °C. Morphological and biochemical characteristics were used for identification of the different microorganisms. Selected colonies were set for multiplication in bioreactors after being placed in liquid nutritive solutions. The identification of strain genera was carried out by the Microbial-Ecotoxicology team (L2GE laboratory, Faculty of Sciences 2, Lebanese University) and the pure cultures were cryopreserved in glycerol at −20 °C. A combination of microbial consortia was realized, providing different products available commercially (NESCO, National Environmental Solution Company, Lebanon) and referred to as LBM (Lebanese beneficial microorganisms). The LBM products used in this field experiment comprised three microbial products (SA, SR, NFPC) and an organic fertilizer (Isdar):
SA (Soil Activator) declared active ingredients: different beneficial bacterial strains including lactic acid bacteria, Actinobacteria, and yeast. SR (Soil Regenerator), active ingredients: lactic acid bacteria, acetic acid bacteria, Cyanobacteria, phototrophic bacteria, symbiotic nitrogen-fixing bacteria, Saccharomyces, enzymes, organic acids, and minerals. NFPC (Nitrogen Fixator and Pests Controller): lactic acid bacteria, nitrogen-fixing bacteria, acetic acid bacteria, phototrophic bacteria, yeast, alcohol, enzymes, and organic metabolites. Isdar (Organic fertilizer) was prepared from chicken manure with 4% nitrogen.
Experimental site and microbial inoculation
A field trial was conducted at Chekka (34°19′N and 35°44′E) in the north of Lebanon during the 2020 summer (May to September) to study the effect of the LBM consortium on plant growth. Three treatments in plots of 24 plants each were planted on a raised bed at a spacing of 43 m × 43 m at the end of May. Seedlings having uniform size (6–8 cm high) were used with spacing of 45 cm between plants. The treatments were as follows: Treatment A: SA+SR+NFPC+ISDAR; Treatment B: SA+SR+NFPC; Treatment C (control): NPK. For application of the different LBM products, suspensions were prepared freshly by dilution in non-chlorinated water at the dose recommended by the manufacturer and used as inoculums at the time of planting the seedlings (for SA, 500 ml/plant) one time per week with the irrigation water during the experiment (for SR, 500 ml/plant) and by spraying the leaves at 10-day intervals (for NFPC). The organic and chemical fertilizer were added to the soil and mixed well before planting (25 g/plant Isdar and 10 g/plant NPK). The soil sample from a depth of 0–20 cm was collected from the experimental site before treatment and analyzed for the major chemical composition with standard methods. The soil composition is mentioned in Table S1 (supplementary materials).
Plant parameters studied
The growth promoting effect of the microbial treatments on both crops was evaluated weekly by determining the shoot length (cm), the number of leaves per plant, and the number of flowers and fruits. Yield was also calculated in terms of kilograms of fruits obtained per m2 (equivalent to 4 plants). In addition, the tomato plants were uprooted at the end of the season, packed in polyethylene bags, and brought to the laboratory for analysis. The average root lengths (cm) and the fresh and dry weight (g) of the roots were recorded.
Statistical analysis
All experiments were run in triplicate. The data are presented in terms of an average of 20 plants ± standard deviation. The data collected were subjected to analysis of variance at a significance level p < 0.05 using Data Analysis Tool of SPSS (version20.0). The Duncan test was adopted to determine the difference between the three treatments, while the paired-sample t-test was used to compare the results with the control.
. Results
Soil properties
To investigate the influence of the LBM combinations versus the control chemical fertilizer in the field, we first evaluated the texture and chemical content of the soil. The obtained results indicate brown sandy soil (63%) with clay (26%) and loam (11%). The assessment of its chemical properties showed a neutral pH and a normal range of electrical conductivity. The carbon and available potash contents were high. Out of the micronutrients estimated, only the nitrogen content was relatively low, but the other nutrients were sufficient.
Effect of LBM consortia on shoot length and number of leaves
A comparative account of plant growth parameters upon the LBM treatments versus the chemical fertilizer was determined using two plants C. annuum and S. lycopersicum. We chose a widely used method to evaluate the vegetative growth and to compare two LBM combinations: supplemented with an organic fertilizer (treatment A) or not (treatment B). The normality of the distributions was verified by the Shapiro–Wilk test. The average values of the shoot length and leaf number differed significantly between the three fertilizer variants. However, Anova did not show significant differences in the shoot length between the fertilizer’s variants applied in S. lycopersicum. Since Anova showed an overall statistically significant difference in the group means, the Duncan Post-Hoc test was conducted.
The results represented in Table 1 indicate a significant increase in the shoot length (in treatment A) and the number of leaves (in treatments A and B) of C. annuum plants at day 14 after transplantation, compared to the chemical fertilizer group (treatment C). We noted that treatment A was characterized by the highest values amounting to 13.07 cm of shoot length and 24.05 leaves per plant, compared to the other treatments. The trend was slightly different with S. lycopersicum plants since the number of leaves increased in treatment A only, whereas no significant variation in the shoot length was observed on day 14. For this reason and to best assess the influence of the LBM treatments, we chose to determine the growth parameters until the flowering period.
Table 1
Effect of chemical fertilizer and LBM consortia on shoot length and number of leaves.
As can be seen from the data recorded on days 20, 35, and 50 (Figure 1a, Figure 1b), both combinations contributed to a significant improvement in the shoot length and leaf number in C. annuum over the control chemical fertilizer. Regarding S. lycopersicum shoot length, no significant effect was noticeable for both LBM consortia on days 20 and 35, while a significant effect was noticeable only in treatment B on day 50 and above (Figure 1c). Nevertheless, a significant increase in the number of leaves was achieved in S. lycopersicum with treatment A at day 14 (Table 1) and for both treatment at day 20 (Figure 1d). Afterward, the positive effect of treatments A and B was narrowed down, and the variation was not significant against the control. In both treatments, the results on the shoot growth in S. lycopersicum were not beneficial, except for the number of leaves punctually; consequently, a possible effect of LBM on the root system was supposed for this plant species.
Figure 1
Variation of plant growth parameters related to time after transplantation and LBM treatments. Measurement of shoot length (a) and number of leaves (b) of C. annuum plants at different days after transplantation (dat). (c) S. lycopersicum shoot length and number of leaves (d). Treatment A: SA+SR+NFPC+Isdar, treatment B: SA+SR+NFPC, treatment C: control (NPK). The asterisk on the top of the bars indicates significantly different mean values at p < 0.05, compared to the control.
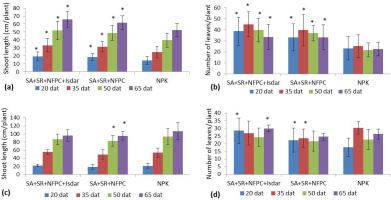
Root growth evaluation
The root length in the treated plants (treatment B) increased significantly, compared with those grown in the control conditions (Table 2). However, the root weight showed significantly higher values in both treatments (A and B). The highest mean values of the root length and fresh and dry weight, i.e., 30.1 cm, 7.06 g, and 1.8 g, respectively, were recorded in tomato plants in treatment B. The roots of the S. lycopersicum plants exhibited a remarkable visual improvement, compared to plants treated with the chemical fertilizer (Figure 2). Beyond root shortening, it is also interesting to notice the lateral root development in the presence of the LBM consortia. The number of visible lateral roots increased in treatments A and B, compared to treatment C, where the chemical fertilizer was applied.
Table 2
Influence of the LBM consortia versus the chemical fertilizer on the roots of S. lycopersicum.
Influence of LBM consortia on crop productivity
The recorded LBM effects on vegetative plant growth determined in the previous experiments were reflected by a significant increase in total fruit yield (Table 3) compared to the control with the chemical fertilizer, except for S. lycopersicum plants in treatment A.
Table 3
Effect of the chemical fertilizer and the LBM consortia on the number of flowers and production of fruits.
Interestingly, both LBM combinations had a positive effect on the final fruit production although treatment A exhibited the highest values for both crops. Up to 37.5% and 31.7% of total yield increase in S. lycopersicum and C. annuum, respectively, was obtained in treatment A, while treatment B resulted in a 23.8% and 18.6% yield increase, respectively, against the control. In terms of the number of flowers per plant, a 51.2% and 28.9% increase was observed in C. annuum on day 50 in treatments A and B respectively, compared with the chemical fertilizer-treated group (treatment C). Similarly, the number of C. annuum fruits per plant increased by 73.4% and 69.3% in the respective treatments on day 72.
To assess whether a similar trend in the number of flowers and fruits could be recorded earlier, we took into consideration these parameters starting from days 35 and 50 respectively. As can be seen in Figure 3, for both parameters, the C. annuum plants performed in a similar manner at the early stages, as a significant increase in the flower number was observed starting from days 35 and 50 in both LBM treatments (Figure 3a, Figure 3b), whereas only treatment A resulted in a significant increase in the fruit number. Regarding S. lycopersicum, only treatment A led to a significant increase in the number of flowers (10.5%) and fruits (34.6%) against the control, whereas a negative influence was exerted by treatment B (Table 3). Irrespective of the day of data recording, similar results were shown by S. lycopersicum (Figure 3c, Figure 3d), where treatment A contributed to the highest numbers of fruits and flowers, while treatment B exhibited lower numbers than in the control (chemical fertilizer).
Finally, regardless of the crop species, the maximum values of parameters related to fruit production (flowers number, fruits number, and total yield) were recorded in treatment A than in treatment B.
Figure 3
Variation of plant productivity related to LBM treatments at different days after trans-plantation (dat). Number of flowers (a) and fruits (b) in C. annuum plants. Number of flowers (c) and fruits (d) in S. lycopersicum plants. Treatment A: SA+SR+NFPC+Isdar, treatment B: SA+SR+NFPC, treatment C: control (NPK). The asterisk on the top of the bars indicates significantly different mean values at p < 0.05, compared to the control.
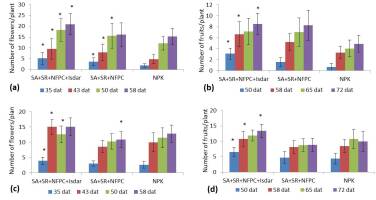
. Discussion
Multi-strain inoculants and combinations of different beneficial microbial species appear to be the current frontier in the field of biofertilization (Bashan et al., 2014), likely because of their complementary activities that strongly influence the outcomes of inoculations (Liu et al., 2017). However, the effectiveness of inoculants relies on various parameters, including plant and soil ecology, geographic location, host interactions, and environmental factors (Dellagi et al., 2020; Giovannini et al., 2020). For instance, microbial strains having plant growth promoting properties continue to be discovered and tested separately or as a mixture (Castanheira et al., 2017). Yet, a reliable path to exploit inoculants for agricultural purposes has not been well developed, mainly because of the complex biological and physiological processes underlying the establishment of a synergistic beneficial effect between microorganisms (Dey et al., 2014). Consequently, isolation of soil and plant-associated microorganisms preserving their natural community and efficient interactions is receiving particular interest nowadays (Chauhan et al., 2015).
In the present study a mixture of local strains retrieved from a proper non-cropped soil in Lebanon rich in wild Crataegus plants was applied as two combinations of liquid inoculants and evaluated for their plant-growth promoting effect in a field trial in comparison to a chemical fertilizer. Remarkably, plants inoculated with both microbial combinations exhibited a significant effect on vegetative growth. The minimum shoot height and number of leaves on day 14 were recorded in the chemical fertilizer treatment (control). The integrated use of LBM produced a moderate increase in the shoot length and number of leaves over the chemical fertilizer at day 14 in the green bell pepper and tomato, respectively. Previous studies have also reported a significant increase in the growth parameters of various species after microbial application, including tomato (Bradacova et al., 2019; Oancea et al., 2017) and pepper (Han et al., 2021; Yu et al., 2011). Usually, plant growth promoting microorganisms can stimulate growth via direct and indirect mechanisms. A hormonal imbalance in plants reflected by elevation of auxins/cytokinins and a decrease in ethylene/abscisic acid can enhance cell division and elongation and increase N and P availability to roots, contributing to amelioration of shoots and roots development (Ruzzi & Aroca, 2015). Strains like those found in LBM, including nitrogen-fixing bacteria, are commonly found associated with plants and are known to improve plant growth and enhance the rhizosphere microbial activity (Aseri et al., 2008). Moreover, Saccharomyces cerevisiae have been previously reported to enhance N and P availability and increase root and shoot biomass in tomato plants (Lonhienne et al., 2014). The amelioration of the tomato root system growth detected in our study may be linked to the presence of yeast in combination with other microorganisms, resulting in enhancement of nutrient uptake favored by a higher roots volume. Consequently, the number of leaves increased, generating a boost in the photosynthetic activity and, in turn, plant growth improvement. Besides, a possible interaction between LBM consortia and other beneficial microorganisms in the soil (bacteria or arbuscular mycorrhizal fungi) cannot be excluded in the explanation of the positive impact of LBM on plant growth. Further in-depth studies of the ecological impact of LBM inoculums on the native soil microbiome should be conducted to have more insights into their modes of action related to their ability to increase nutrient uptake.
The results obtained in this work highlight the efficiency of the native microbial consortium not only in boosting plant growth but also in increasing their productivity even in the absence of organic fertilizer. Based on this investigation, the LBM consortia revealed a 32 to 38% increase in total fruit yield in the presence of the organic fertilizer against 18.6 to 23.8% in its absence in both crops, respectively, compared to the chemical fertilizer variant. Our results corroborate with others showing a greater fruit yield following inoculation with bacterial strains in C. annuum or microbial consortia in tomato plants (Bradacova et al., 2019; Thilagar et al., 2018). Various studies have reported an increased fruit yield at different percentages related to strain inoculation, ranging from 15.5% (Chiquito-Contreras et al., 2017) to 31.7% (Reyes-Ramírez et al., 2014). Another study showed an increase in total tomato yield by 10% achieved using a mix of strains (Oancea et al., 2017). Thus, the yield-increasing potential of LBM is acceptable even in the absence of a chemical fertilizer. The authors speculated that the observed effects were related to the accelerated vegetative growth where the fruits ripened more quickly. It remains to be elucidated whether the improved crop performance after LBM inoculation is reflected by the observed beneficial yield effects. An indirect proof of the LBM potential effect on soil microbial communities is the extended period of vegetative growth and fructification of both crops (data not shown), which is in correlation with the improved nutrient uptake and efficient use.
Overall, the values of all plant growth parameters (plant height and leaf number as well as the number of flowers and fruits) were higher when LBM was incorporated along with the organic fertilizer. This result may be attributed to an increase in the availability of substrate through organic material resulting in high microbial activity and release of enzymes into the soil (Meena & Rao, 2021). The soil enzyme activity is recognized as an indicator of soil health. A regular addition of organic amendments is often an important part of the strategy aiming to enhance the predominance of beneficial microorganisms in the soil (Higa & Parr, 1994). On the other hand, our results showed that the amendment of the organic fertilizer was accompanied by an increase in roots biomass in the tomato explants. The strengthening and root biomass promotion of tomato plants after LBM inoculation may therefore improve the use of the applied organic fertilizer.
Microbial biofertilizers represent an alternative strategy to promote plant growth, but their output is still controversial. In some reports, the application of microbial consortia resulted in similar yields as conventional mineral fertilizers (Adesemoye et al., 2009; Pagnani et al., 2018), whereas other studies showed the necessity of NPK application along with microbial strains to improve plant growth and yield (Esitken et al., 2010; Thilagar et al., 2016). Nevertheless, the addition of a chemical fertilizer to microbial consortia does not seem to ameliorate the soil properties after harvest (Thangasamy & Lawande, 2015), neither chemical (organic carbon, available nitrogen, phosphorus, and potassium) nor microbial (bacterial, Actinomycetes, and fungal count) (Tinna et al., 2020). Contrary to previous reports, the most interesting outcome in the current study is the efficiency of the natural consortia in the absence of a chemical fertilizer. Hence, the use of natural inoculants offers the scope of saving 100% of mineral fertilizers while promoting plant growth parameters and yield most probably through the amelioration of the soil conditions.
This study indicates that the LBM inoculants exerted stimulatory effects on vegetative growth and yield production in both crops, although a superior effect was detectable in C. annuum. Species-specific responses to LBM inoculation cannot be excluded in the explanation of the observed differences between tomato and pepper. Studies have shown that the growth promoting ability of some bacterial strains may be highly specific to certain plant species, cultivars, and genotypes (Bashan & Holguin, 1998).
The LBM consortium showed good performance in the field trial, which is an important condition and prerequisite for commercialization of products. Various parameters, such as plant species, soil characteristics, and weather conditions, determine the effectiveness of inoculation; hence, inoculants should also be harmonized appropriately. Therefore, the use of native strain assemblies seems to have a greater effect on plant growth promotion, since the mixture maintains a harmonious compatibility between microorganisms (Cappellari et al., 2015). Hence, it is highly probable that these microorganisms become established in the soil and become effective as an associative group more than the use of a single strain.
. Conclusions
The present study demonstrates that LBM composed of naturally assembled multi strain species exert a plant growth-promoting effect and have yield-increasing potential in tomato and green-bell pepper cultures. The results indicate a direct and rapid effect of the inoculums on the growth conditions, manifested by improved starting conditions from earlier growth phases (2 weeks after planting for C. annuum), leading to remarkable yield increases. Both LBM combinations exerted a beneficial effect on crop performance not only during vegetative plant development but also in the flowering and fruit development phases. The organic amendment enhanced the outcome of microbial consortia and seems an essential condition to enhance crop productivity, at least in the tested plant species.
The findings presented in this experiment indicate that natural microbial consortia are efficient even in the total absence of chemical fertilizers. The precise mode of action of LBM remains unclear; however, their ability to improve crop growth and yield was clearly demonstrated, particularly under challenging environmental conditions. Exploiting the efficacy of the combined LBM-organic compounds is very promising in the development of new plant biofertilizers required for sustainable agriculture.