. Introduction
Pollen grains are found in the flower anthers, which are necessary for sexual reproduction in angiosperms. Estimation of pollen viability and vigor can be achieved by induction of in vitro pollen germination. Pollen grains are considered to be extremely minuscule systems combining anatomical simplicity with rapid growth rate of the pollen tube (Sawidis, 2008; Sawidis & Reiss, 1995). The process of pollen tube growth and elongation serves as an excellent model for studying plant cellular morphogenesis at the single-cell level. This process can be influenced by many external factors, including various toxic substances. The ability of pollen tubes to grow in vitro makes them a practical model for real-time testing of the effect of heavy metals on the ultrastructural level.
For many years, the pollen tube has been used as a well-suited model system for the evaluation of metal toxicity in plant cells (Gur & Topdemir, 2005; Sawidis et al., 2018). The effects of heavy metals on germinating pollen grain are one of the most interesting questions, and propagation experiments as well as studies of in vitro pollen germination in flowering plants are very useful for explaining any lack of plant fertility and the importance of reproduction for the continuity of species. In the past decades, pollen tubes of various plant species have been used to determine the cytotoxic effects of environmental pollutants (Cox, 1988; Röderer & Reiss, 1988; Xiong & Peng, 2001).
Among various metals, manganese is one of the 17 essential elements for growth and reproduction of biological systems, and an overabundance can be harmful. An increased dose of manganese in food has various effects on human physiology, resulting in disturbances in the nervous system (Leita et al., 1996). As a component of more than 35 different enzymes, manganese either stimulates or greatly affects various biochemical and physiological functions in plant metabolism (Andresen et al., 2018; Diedrick, 2010). It is also involved in aspects of plant tip growth, such as pollen tube growth and root hair cell elongation (Somerville & Nicol, 2002). On the contrary, manganese is an important industrial metal, the concentration of which is increasing rapidly in our biosphere. As a result, the metal is accumulated in plants in areas with high anthropogenic pressure. Elevated levels of manganese in pollen have been reported in cases of industrial, urban, and rural sites, with concentrations ranging from 21 to 110 ppm (Kamran, 2000).
In previous studies, the influence of a range of various heavy metals on pollen germination and tube growth in Lilium longiflorum was studied, and the general mechanism of heavy metal action on pollen tube growth was discussed (Sawidis & Reiss, 1995). Among these metals, manganese has shown a particular influence, and this was the reason for a more detailed study that extended the metal concentration range and modified the type of metal treatment. The main goal of the present study was to determine the effects of manganese on Lilium pollen, with a focus on anther morphology, pollen germination, pollen tube growth, and ultrastructural effects at the subcellular level. Such effects are consequential in flower fertilization and fruit set. The aim of the present study was to investigate the effects of low and high doses of manganese on pollen germination and tube growth, which are important for flower fertilization and fruit setting.
. Material and Methods
Flower Bud Treatment
A series of standard solutions of MnCl2 (10−12–10−2 M) was prepared in distilled water. Pedicels of ready-to-open flower buds of Lilium longiflorum Thunb, cultivar ‘Snow Queen,’ were placed in the solutions for 24 hr, simulating the natural uptake of heavy metals. The experiment was repeated three times, and three buds in each replication were tested. The control treatment in each case was the placement of flower buds of the same age in distilled water for the same duration. The flower buds were then placed in a controlled growth chamber (temp. day 24 °C, temp. night 15 °C, light 600 µ mol, humidity 65%, CO2 390 ppm), and the successive weights of the flower buds were taken at 3-hr intervals for 24 hr, starting with the beginning of the experiment. The use of large and well-shaped flowers of Lilium offers a good model for studying the dehydration process after metal treatment because they can be easily handled.
After 48 hr, anthers from the manganese-treated flower buds were removed. The length and width of the anthers were measured using a stereoscope and the results recorded. The pollen grains from these anthers were separated using a small brush, after which the grains were sown onto Petri dishes containing a normal aqueous growth medium of 10% (w/v) sucrose and 10 ppm boric acid and allowed to germinate at room temperature for 1 hr. Boron has a regulatory role in pollen germination and tube growth by affecting H+-ATPase activity (Wang et al., 2003). It is also instantly involved in pectin synthesis and development of the pollen tube wall (Bhattacharya & Mandal, 2004). The experiment was repeated three times, and approximately, 100 pollen grains per culture were evaluated.
Pollen Germination in Medium Containing Manganese
In another experiment, pollen grains from freshly opened flowers of the same lily cultivar were left to germinate in the above-described series of growth media, which contained additionally 0 M (control) and 10−12–10−2 M MnCl2. Pollen grains were sprinkled on the medium by brushing on each Petri dish. The germination rate was measured using a dissecting microscope 1 hr after the start of cultivation. The experiment was performed in triplicate, and the percentage of pollen germination for each Petri dish was calculated (Ahmad et al., 2012; Gandadikusumah et al., 2017). From each culture, approximately 100 pollen grains were observed and identified as germinated or nongerminated depending on whether a pollen tube could be seen emerging and whether the length of the pollen tube was greater than the diameter of the pollen grain (Rodriguez-Enriquez et al., 2012).
Microscopical Observations
For ultrastructural study, manganese-treated and control pollen grains were fixed with 2% glutaraldehyde buffered in 0.1 M cacodylate (pH 7.2) and postfixed in 1% osmium tetroxide in the same buffer. After dehydration in an ethanol series, the specimens were finally embedded in Spurr’s epoxy resin (Sawidis & Reiss, 1995). Ultrathin sections (0.08 µ m) were investigated using a Philips EM 400 and a Jeol (JM10) transmission electron microscope (TEM).
. Results
Water evaporation was significantly reduced in all manganese-treated flower buds in comparison to the control (Figure 1). Water loss was diminished by the progressive increase of the manganese concentration. The highest decrease in water evaporation was observed at 10−2 M. After manganese treatment, the water tissue capacity increased, and the dehydration was delayed as the concentration increased. Low concentrations showed lower water loss reduction, similar to the control (Figure 1). The anthers of the treated flower buds became shorter than those in the control, and they did not open fully. The longitudinal axis decreased while the diameter of the anther increased, resulting in its more-or-less oval shape. This was most obvious at high manganese concentrations (10−6 and 10−4 M), whereas at a concentration of 10−2 M, anthers showed incomplete development (Figure 2).
The pollen germination rate of lily flower buds after treatment with manganese ions is shown in Figure 3. The germination ability of pollen grains taken from treated flower buds was reduced to some degree at high concentrations (10−8–10−2 M) in comparison to the control. In contrast, the manganese treatment at low concentrations (10−12–10−10 M) increased the pollen germination rate.
Figure 3
Lilium longiflorum pollen germination after manganese treatment with various concentrations for 24 hr.
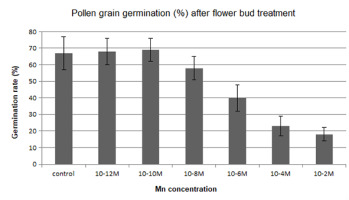
In another series of experiments, pollen grains were left for 1 hr to germinate in the culture medium containing manganese. Pollen germination was adversely affected in the presence of this metal, and a reduction in the percentage of germinated pollen grains was generally observed in comparison to the control (Figure 4). On the contrary, pollen germination was stimulated by the manganese ions at low concentrations (10−12–10−10 M).
The presence of manganese ions in the culture medium resulted in significant disorders, characterized by an uneven pollen tube growth showing varying morphological responses. Initially, a slight swelling was observed at the tip in most of the examined tubes (Figure 5). A number of mitochondria, plastids, and some small-sized vacuoles were also present near the tip region. The swelling progressed basally, and an irregular increase of tube diameter was observed in close distance to the tip region. However, at the basal region, the tube diameter remained at normal size (Figure 6). The pollen tube growth pattern differed among tubes, revealing a great variability. In the next stages, the formation of cell wall thickening in the tip region prevented further swelling and tube constriction in this area. The swelling continued basally, depending on the thickness and elasticity of the cell wall (Figure 7).
Figure 5
A slightly swelling pollen tube of a Lilium longiflorum with thick cell wall at the tip region (arrows). Scale bar = 2 µ m.
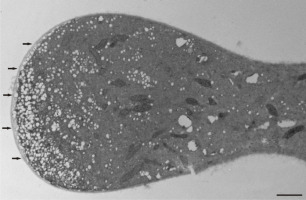
Figure 6
Abnormal increase of a Lilium longiflorum pollen tube diameter below the tip area. At the basal area the pollen tube, diameter is normal. Scale bar = 10 µ m.
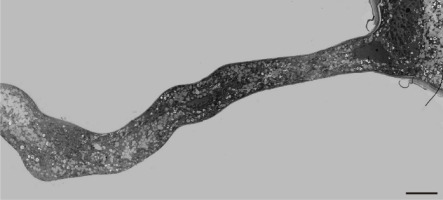
Figure 7
Thick cell wall in a Lilium longiflorum tip prevents further tube swelling in this region (arrows). Scale bar = 10 µ m.
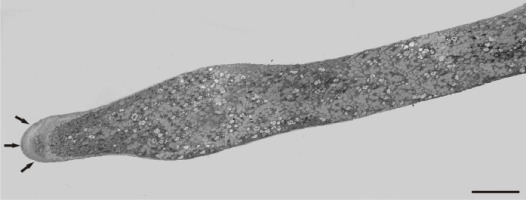
Under normal conditions (control), a large number of Golgi vesicles was visible at the tip (Figure 8). The most significant effect of manganese treatment was the anomalous pollen tube cell wall formation, mainly in the tip region. Abundant, densely compact mitochondria, plastids, and lipid droplets (clearly distinguishable from vacuoles) were found at a short distance from the tip within the apical clear zone. The dictyosomal ultrastructure was not visibly disturbed (Figure 9). An intense presence of vesicles was observed, and the cell wall began to thicken abnormally in the apical region. A prominent effect was the abnormal cell wall organization. Distinct cell wall layers were visible: the outer ones were striated with a fluffy, fibrillar network on the outer surface, whereas the inner layers contained distinct wall globules. On the inner side of the tip region, the apically accumulated vesicles create an additional wall layer directly above the plasma membrane (Figure 10).
Figure 8
Control pollen tube of Lilium longiflorum at the tip area. Numerous Golgi vesicles (arrows) approaching the cell wall and a few mitochondria (asterisks) at a short distance from the tip. Scale bar = 1 µ m.
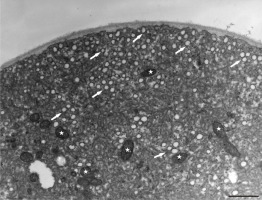
Figure 9
Disruption of organelle organization at the tip area of Lilium longiflorum pollen tube. Plastids (arrow) and lipid droplets (asterisk) are present in a short distance from the tip. Scale bar = 1 µ m.
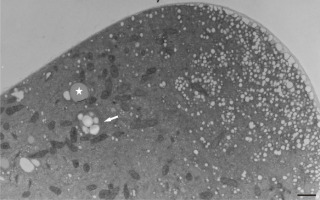
Figure 10
Abundant vesicles (white arrows) at the tip region of Lilium longiflorum pollen tube create an additional wall layer (asterisks) directly above the plasma membrane. The outer cell wall layer showed a fluffy appearance, with dispersed fibrillar material (grey arrows). Scale bar = 1 µ m.
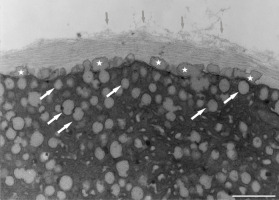
After prolonged manganese exposure, globular aggregates were embedded in a fine fibrillar network at the pollen tube tip (Figure 11). These globules were interpreted as Golgi secretion vesicles. Gradually, this accumulated material was loosened, beginning with the outer layers, until its final dispersion in the medium (Figure 12). The protoplast at the tip was compressed, as the functional space was limited by the overgrowth of the cell wall, the thickenings of which consisted of several layers of condensed material (Figure 13). In the pollen tube cell, there were numerous mitochondria and plastids, completely filled with starch (Figure 14). Plastids have been repeatedly observed in close proximity to strongly electron dense mitochondria, whereas rough ER cisternae also appeared near these organelles at the inner cell wall site (Figure 15).
Figure 11
Multiple layered cell wall (asterisks) at the tip of Lilium longiflorum pollen tube. From the external layer a fine fibrillar material (arrows) was separated and dissolved in the environment medium. Scale bar = 1 µ m.
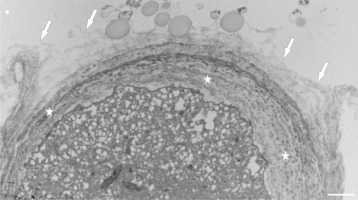
Figure 12
Electron dense clumped wall material of globular aggregates (arrows) immersed in the thick cell wall at the tip of a Lilium longiflorum pollen tube. The outer layers of the cell wall (asterisks) are gradually disorganized. Scale bar = 500 nm.
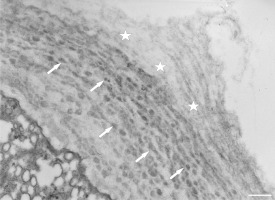
Figure 13
Extremely thick cell wall at the tip of a Lilium longiflorum pollen tube, with distinctly visible individual layers. Scale bar = 1 µ m.
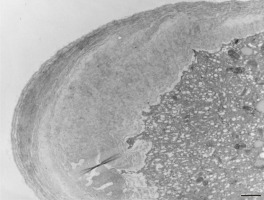
Figure 14
Numerous electron-dense mitochondria and plastids containing starch granules (amyloplasts) in a Lilium longiflorum vegetative cell. Scale bar = 1 µ m.
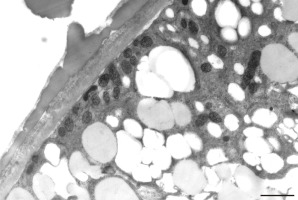
Figure 15
Starch-rich plastid (P) coupled with a mitochondrion (M) near the cell wall of a Lilium longiflorum pollen tube. ER cisternae are also present. Scale bar = 500 nm.
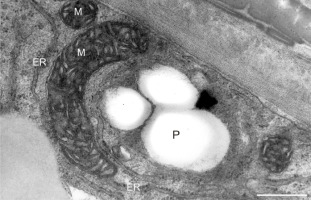
In addition to the abnormal cell wall structures, electron dense globular (EDG) intracellular precipitates could be observed along the plasma membrane. The Golgi vesicles did not appear to fuse with the cell wall. On the contrary, they merged with each other, creating inactive aggregates (Figure 16). Vacuoles near the plasma membrane functioned as a primary compartment for a temporal EDG internalization (Figure 17). The marginalization of EDG and vacuoles containing EDG along and in close contact with plasma membrane started from the pollen grain and progressed distally. When EDG approached threateningly the tip region, the vegetative cell reacted with formation of an internal, mechanical barrier to prevent metal permeation in the tip area (Figure 18). For this purpose, mitochondria were mainly recruited, accompanied by other organelles, such as vacuoles and dictyosomes, but not plastids, which remained behind (Figure 19–Figure 21). In many cases, mitochondria supporting the formation of the mechanical barrier were so electron dense that their cristae were obscured (Figure 22).
Figure 16
EDG (arrows) along the plasma membrane in a Lilium longiflorum pollen tube. A small vacuole (V) contained some of them. Golgi vesicles do not fuse with the plasma membrane but rather agglomerate in an aggregate (asterisk). Scale bar = 500 nm.
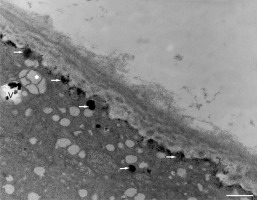
Figure 17
Deposition of excess manganese in the form of EDG along the plasma membrane in a Lilium longiflorum pollen tube and their compartmentalization into the vacuoles. Scale bar = 500 nm.
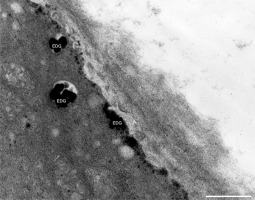
Figure 18
Internal barrier (arrows) separating the tip region from the rest of the vegetative cell in a Lilium longiflorum pollen tube. Scale bar = 1 µ m.
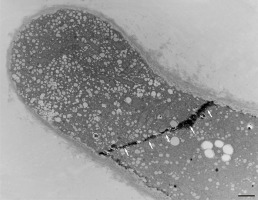
Figure 19
Manganese-treated Lilium longiflorum pollen tube: details of the internal barrier made mainly by mitochondria (M). Scale bar = 500 nm.
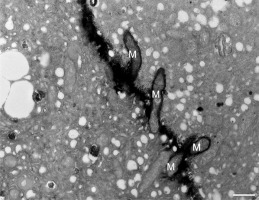
Figure 20
Manganese-treated Lilium longiflorum pollen tube: Longitudinal and cross section of barrier mitochondria (M). Scale bar = 500 nm.
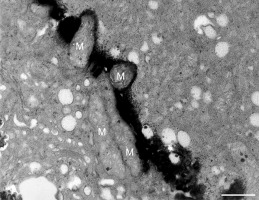
. Discussion
In nature, the rate of evaporation from flower parts (e.g., petals, sepals) depends on radiation level, temperature, humidity, and wind speed. Under experimental conditions, these factors were kept constant. Water evaporation rate was significantly diminished by progressive increase of the manganese concentration. Water capacity is significant in the maturation of pollen, and some trace metals are required for water balance within the anther tissue (Sawidis et al., 2014). After heavy metal uptake, water molecules are strongly adhered to positively charged metallic ions, causing an overhydration of the tissue and hence the delay of natural evaporation. Moreover, the limited opening of flowers at high metal concentrations also slows the evaporation of water.
The manganese requirement for the development of anthers is significant, and deficiency of this ion decreases pollen production and pollen fertility (Mousavi et al., 2011). Alternatively, excess external manganese results in alterations in anther size and shape, although among flower parts, the anthers showed the lowest metal uptake values (Sawidis, 1997). At the molecular level, excessive manganese can reduce the number of meristematic cell divisions (Zhao et al., 2017). The inhibition of anther development is closely connected to the mitotic activity of the tapetum layer, as demonstrated in meristematic tissues (Fusconi et al., 2007). After manganese treatment, the cell wall becomes more compact, with limited elasticity, preventing the lengthening of the anther. Changes in the size or shape of the anther create additional problems in pollen transport and pollination process.
Anther development requires a sufficient amount of manganese; its deficiency delays pollen grain production, viability, and fertility (Reuter et al., 1988; C. P. Sharma et al., 1991). As was shown in the present study, the external supply of manganese increased the pollen germination rate at low concentrations (10−12–10−10 M), whereas high concentrations had negative effects. Anthers can provide some protection to the developing pollen grains, especially the intermediate tissues, allowing only a small proportion of the applied substance to penetrate them. It is suggested that the increased rate of pollen germination, in the presence of low magnesium concentrations, might be due to higher enzymatic activity and mobilization of stored sugar inside the pollen grain (Bhattacharya, 2017).
Manganese affects pollen germination depending on its concentration. Low metal concentrations can stimulate enzymatic activities, exhibiting an activation of pollen germination. This phenomenon, known as “hormesis,” was defined as a stimulus caused by a low dose of a toxic substance (Sawidis, 2008, Sawidis et al., 2018; Stebbing, 1998; Xiong & Peng, 2001). In the case of manganese, “hormesis” was extended to somewhat high concentrations, whereas negative results were recorded at a concentration of 10−6 M. In vitro germination of pollen grains was reduced significantly under lack of manganese (P. N. Sharma, 1992). Thus, it is necessary to determine the optimal concentration of various trace metals for pollen germination (Dafni & Firmage, 2000).
A considerable reduction in pollen germination might affect seed set if infertile pollen grains are transported to the stigma. It is also possible that the pollen from metal-stressed plants might have a short lifespan and reduced tube growth rate (Turner et al., 2004). Excess of manganese reduces cell wall plasticity and extensibility, causing morphological and ultrastructural anomalies in developing pollen tubes. The inactivation of Golgi vesicle material is the main reason for anomalous tube growth, and the negative effects of manganese might be related to the capacity of the cell wall to bind metals, thus inhibiting normal cell wall formation (Sawidis, 2008; Sawidis et al., 2018; Serregin & Kozhevnikova, 2009). The main ultrastructural changes are disorganized intracellular organelle distribution, confirmed by electron microscopical studies (Polevova et al., 2014; Speranza et al., 2007), whereas biochemical or physiological investigations on pollen germination and tube growth in vivo are rather difficult.
The pollen cell wall is composed of several layers as an effective barrier to the entry of toxic substances at high doses (Führs et al., 2010). An additional barrier might be represented by lignin deposition, and the polar growth of the pollen tube requires the continuous delivery of new materials carried by Golgi vesicles to the apex. Excess external manganese causes abnormal callose accumulation at the pollen tube tips, resulting in reduced pollination success (Çetinbaş-Genç et al., 2019). Additionally, depolymerization of the actin cytoskeleton inhibits normal pollen tube growth (Cai et al., 2015).
Our observations of the components of the vegetative cell showed that plastids often became coupled with mitochondria near the cell wall. These organelle arrangements minimize distances for traversing signals from one to the other. Thus, mitochondrial and plastid peptidases are functionally interacting in pollen germination and tube growth, because the signal translocation becomes much easier by direct contact between the two organelles. The production of enzymes involved in cell wall formation by consumption of plastid starch is consistent with the growth and expansion of the cell wall, a critical process for the developing pollen tube. Heavy metal stress might cause inactivation of these enzymes in the chloroplast and mitochondria, resulting in pollen tube deformation.
If a dangerous amount of metal enters the cytoplasm of a vegetative cell, it must react quickly. Precisely, the excess external manganese amount must be isolated to an appropriate location away from the tip region, as the continuous growth of the pollen tube is essential for the delivery of sperm cells to the female gametophyte. For this purpose, vacuoles can serve as a reservoir to regulate cellular manganese homeostasis (Dou et al., 2009; Dučić & Polle, 2007). Vacuoles, which generally function as a primary compartment for metal internalization to avoid toxicity, serve as a temporary storage pool (Lanquar et al., 2010). Metal sequestering in vacuoles or Golgi vesicles is a mechanism of tolerance under excessive conditions (Pittman, 2005).
At the molecular level, excessive manganese can lead to an increase in the accumulation of oxidized manganese and oxidized phenolic compounds (Fecht-Christoffers et al., 2006). These precipitates were found in the form of electron-dense globoids (EDG) along the plasma membrane and in vacuoles (the present study and Bruch et al., 2015; Donner et al., 2012; Mary et al., 2015). In such cases, pollen tubes activate mechanisms to isolate the metal and protect the tube tip. Ultimately, the internal barrier is considered as a part of the response mechanism against penetration of manganese to the tip.
Given the multitude of mitochondrial functions in plant cells, it is not surprising that this mobile organelle acts as a primary structural element of the internal barrier. Moreover, mitochondria are the site of essential biosynthetic pathways, controlling cellular homoeostasis in multiple ways (McBride et al., 2006). The manganese transport and efflux strategies are not completely understood, despite the crucial role of manganese in the activity of mitochondrial superoxide dismutase (SOD). Under excess manganese, elevated SOD activity limits cell damages (Alejandro et al., 2020; Corpas et al., 2017), thus protecting pollen tube growth.
. Conclusion
Manganese toxicity influences pollen germination and tube growth, adversely affecting both processes above a certain low concentration. As a pollutant in industrial, urban, and rural sites, manganese might impact pollen viability and vigor of various plant species. Enhanced manganese levels can affect the quality of the pollen, a precondition for fertilization and fruit setting. Decrease in seed production and loss of biodiversity are critical aspects in the life cycle of all plant organisms. Knowledge regarding the effects of manganese on pollen germination and tube growth will have practical benefits in reproductive biology, especially the prevention of reduced fruit set, which ultimately lowers yield.