. Introduction
Rice (Oryza sativa L.) is the second most important agricultural crop and plays an inevitable role in feeding the global population (Taratima et al., 2023). Rice has also the second-largest cultivated area in the world (Fukagava & Ziska, 2019). Blast disease, which is caused by Pyricularia oryzae Cavara (Magnaporthe oryzae Couch), is considered the most serious rice disease in most of the rice-growing countries of the world, including Iran (Safari Motlagh, 2021). Usually, the symptoms of the disease are in the form of spindle-shaped spots that are gray or white in the middle and brown at the edges and are scattered along the length of the leaf. They create bigger spots in suitable conditions by connecting with each other. If the fungus attacks the plant before the formation of a raceme, it prevents the creation of clusters and seeds, and when the pathogen moves towards the neck of the cluster, due to the loss of tissue, the cluster becomes brown and bent down and the field from a distance looks as if it was burnt (Khodaparast & Sahragard, 2004).
The disease is prevalent in all areas where rice is grown and has been documented in over 85 countries. Consequently, rice blast has the potential to significantly impact food security, particularly in Asian nations, where 55% of the global population resides and where 92% of rice production and consumption takes place (Zakaria & Misman, 2017). In Iran, in 1974, almost 10% of the entire rice crop of Guilan (the rice-growing province of northern Iran) was lost due to this disease (Javan Nikkhah, 2001). Therefore, extensive studies are needed to control the pathogen, e.g. by the development and utilization of fungicides, identification of resistance genes, creation of resistant cultivars, or preparation of early warning systems (Guimarães et al., 2017). Due to the variety of local cultivars and their earliness as well as the changing weather conditions in different crop years and places, controlling the disease without consideration of forecasting operations is very difficult and has been unsuccessful in many cases (Duong et al., 2023). On the other hand, due to the significant fluctuations in the prevalence of the disease and the degree of its spread from year to year, from region to region, from cultivar to cultivar, and even from one farm to another, the use of fungicides without considering the prognostic factors will not be useful. Therefore, in recent years, biological control has been conducted by many researchers (Guimarães et al., 2017).
The danger caused by the application of chemical pesticides has been taken for granted, and the effort to provide suitable alternative methods for chemical substances has led to many advances in this regard. The efficiency of the biological control methods depends on the correct management of research, marketing, education, and promotion. Screening for biological control agents is a fundamental step in the development of this strategy (Lahlali & Hijri, 2010). Useful microorganisms for the biological control of plant pathogens have been identified among fungi and bacteria (Safari Motlagh & Usefipoor, 2016). The investigation of fungal and bacterial agents revealed that between 1 and 10% of them have the ability to prevent the growth of pathogens in laboratory conditions, but only a few of them reduce plant diseases in different growth conditions, and even fewer have a wide spectrum of activities against several different pathogens (Lahlali & Hijri, 2010). Cook and Baker (1983) used fungi isolated from the soil and irrigation water of paddy fields against Rhizoctonia solani and found that Trichoderma viride and Aspergillus niger are the most effective in preventing the linear growth of the disease. In another research on the biological control of P. oryzae, it was found that Trichoderma harzianum and Chaetomium globosum caused 70–80% inhibition in the growth of mycelium and germination of pathogen’s conidia (Gouramanis, 1997). In another study, Hajano et al. (2012) evaluated the biological control of P. oryzae by endophytic fungi and concluded that the greatest inhibition in the mycelium growth of this fungus was caused by Paecilomyces lilacinus and some species of Trichoderma, such as T. harzianum and T. polysporum. The antagonistic property of Epicoccum sp. against pathogenic fungi of rice, such as Magnaporthe oryzae and Cochliobolus miyabeanus, was investigated in the laboratory and greenhouse by Sena et al. (2013). In evaluating the effectiveness of biocontrol agents against Magnaporthe grisea, it was found that the use of Trichoderma and Bacillus subtilis strains together had the greatest effect in controlling the disease (Ali & Nadarajah, 2014). Moreover, it was found that the use of Annulohypoxylon, Nigrospora, and Penicillium extracts prevented the germination of M. oryzae (Thi et al., 2015). Nonetheless, the effects of biocontrol agents in natural conditions are complex and depend on numerous interactions with plants, pathogens, antagonists, and environmental conditions. It seems that dealing with the native biological control agents of each region in each agricultural ecosystem is one of the most eminent steps in applying biological control, but more studies in this regard are needed.
The antagonistic activity of some strains of endophytic fungi and bacteria, such as T. harzianum, Bacillus amyloliquefaciens, and B. cereus, against P. grisea that causes gray spot disease in Lollium plants was proven in laboratory and greenhouse trials (Dammie, 2017). Evaluation of metabolites obtained from Trichoderma isolates revealed that these compounds increased rice yield and reduced the severity of rice blast disease in the field (Khosravi et al., 2011). The biological control of this disease was studied using local isolates of Trichoderma in Mazandaran province, Iran, and it was found that, in the dual culture method, over 90% of Trichoderma isolates suppressed the growth of pathogenic fungi. In greenhouse experiments, RP1-6 and RP4-2 Trichoderma isolates were the most efficient in reducing the disease severity with 100% and 99% efficiency, respectively (Javadi et al., 2014). It is worth expanding the range of species studied beyond those characterized so far as biocontrol agents. This research aimed to identify and evaluate the fungal isolates obtained from the natural flora of rice plants grown in Guilan province fields, Iran, in the suppression of P. oryzae in vitro and in greenhouse conditions.
. Materials and methods
Sample collection, separation, and identification of fungal isolates
Samples were obtained from infected and uninfected plants of O. sativa cv. Hashemi in the fields and nursery of Guilan province, Iran. Samples collected from the infected parts of the plants (seedlings, leaves, and panicles) were separately packed in plastic packages and sent to the laboratory to be stored at 4–5 °C.
To isolate P. oryzae, 2–5 mm fragments were cut from the space between the infected and uninfected tissues of the leaves and panicles of rice. Surface disinfection was performed with 5% (v/v) sodium hypochlorite (NaOCl) for 1 min, followed by three times of surface rinsing with sterile distilled water for 30 seconds. After draining, the fragments were placed in potato dextrose agar medium (PDA) (Merck Millipore, Burlington, MA, USA) in Petri dishes and incubated at 28 °C for seven days (Javan Nikkhah, 2001). The isolates were purified with the single sporulation method. Then, the Petri dishes were kept at 27 °C. The isolates were kept in test tubes containing a PDA culture medium.
To isolate the studied endophytic isolates, 5–10 mm fragments were sampled from the healthy tissues of leaves and panicles. Next, they were rinsed with sterile distilled water for 30 seconds. After draining, the samples were transferred to PDA-containing Petri dishes in a sterile hood. After incubation at 26 °C for 2–3 days, the fungal colonies were isolated and a disc from each colony was transferred to another Petri dish that contained the PDA culture medium. Following incubation for another 2–3 days, a patch of the fungal colony was put in the 1.5% (w/v) water agar (WA) culture medium. Next, after 2–3 weeks and spore production, the fungi were purified with the single spore method. Diagonal test tubes containing the PDA medium were used to keep the fungal isolates.
After separating and purifying the fungi, their macroscopic characteristics, such as the shape and color of the colony and the growth method on the PDA medium, were investigated. Then, their microscopic characteristics were evaluated by preparing microscopic slides and using different identification keys (Ellis, 1971; Gams & Bissett, 1998; Leslie & Summerell, 2006; Reper & Fennell, 1965; Samson et al., 2007; Simmons, 1967, 2007; Sivanesan, 1987). After the morphological identification, molecular identification was done. For this purpose, genomic DNA was extracted based on the method proposed by Zhong and Steffenson (2001). Polymerase chain reaction (PCR) was performed using the ITS1/ITS4 primer pairs to amplify the ITS-rDNA region by a thermocycler (Bio-Rad, Hercules, CA, USA). The thermal PCR program, based on the method developed by White et al. (1990), was used to amplify the rDNA-ITS genomic region, and electrophoresis was performed using 1.5% agarose gel. Amplified products were sent to Bioneer Corporation - Daejeon, Korea, for purification and nucleotide sequencing. Evaluation of the similarity of the obtained sequences with the sequences in the gene bank (NCBI GenBank) was done using the BLAST search tool.
Pathogenicity test
The pathogenicity test was performed according to Koch’s principles in a desiccator environment; for details see a paper by Safari Motlagh and Mohammadian (2016).
Biological control studies
Dual culture method
The method proposed by Sivakumar et al. (2000) was used to perform the experiment. A mycelium disc (5 mm diameter) taken from the marginal parts of the 5–7-day-old culture of P. oryzae was placed under a sterile hood in a 12 cm Petri dish with the PDA medium, at a distance of 2 cm from the wall of the dish. The dish was incubated at 26 °C for 48 h to start the growth of the pathogen. Then, a 5 mm mycelium disc taken from the sides of the 5–7-day culture of endophytic fungi was placed at a distance of 3 cm from the pathogen. In the control Petri dishes, a mycelium disc from the sides of the 5–7-day-old P. oryzae culture was placed in the center of the Petri dish only. All dishes were incubated at 26 °C. After 10 days, the diameter growth of P. oryzae was measured in the control and treatments. The decrease in the diagonal growth in relation to the control was calculated based on the following formula:
where: C and T are the radial growth of P. oryzae in control Petri dishes (C) and the radial growth of P. oryzae in the presence of endophytic fungi (T), respectively (Sivakumar et al., 2000).
Culture filtrate method
The method developed by Dennis and Webster (1971a) was used to perform the analysis. For this purpose, isolates of the studied fungi were cultured in 250 mL vessels containing potato dextrose broth (PDB) culture medium and placed on a shaker at 70 rpm for 10 days at a temperature of 26 °C. Extraction was done using a biological filter and a vacuum pump. The extract (25%) was added to the PDA culture medium. The extract added to the PDA medium in the control Petri dishes did not contain any fungi. A mycelium disc from the 3-day-old P. oryzae culture was placed in the center of the treatment and control Petri dishes. The diameter growth of P. oryzae was measured after 10 days in the incubator with a temperature of 26 °C, and the percentage of inhibition of mycelial growth was calculated.
Volatile metabolites method
A 5 mm mycelium disc from the margins of the 3-day-old P. oryzae culture was placed in the center of a Petri dish containing the PDA culture medium. A 5 mm disc from the 3-day culture of the studied endophytic fungi was placed in the center of another Petri dish containing the same culture medium after 48 h. Then, the lids of these Petri dishes were removed under the sterile hood and the dish containing P. oryzae was placed upside down on the Petri dish of the studied fungi. In the control, a Petri dish containing PDA culture medium without any endophyte was placed in front of the Petri dish containing P. oryzae. The inhibition percentage was calculated after 10 days (Dennis & Webster, 1971b; Sivakumar et al., 2000).
Slide culture method
A clean laboratory slide was placed on two L-shaped glass rods inside a 12 cm Petri dish and sterilized. Then, a drop of 2% molten agar-water culture medium was poured on the slide to form a thin layer of agar. Small mycelium discs (10 mm) of the endophytic fungus and P. oryzae were placed on the slide at a distance of 2 cm from each other. To prevent drying, a few milliliters of sterile distilled water were added to each Petri dish. The dishes were incubated at 26 °C (Sivakumar et al., 2000). From the third to the seventh day, the slides were placed under the light microscope and such features as the fungal hyphae reaching each other and their interaction with each other were examined.
Greenhouse experiments
The seeds of rice cv. Hashemi were disinfected in 30% Clorox (v/v) for an hour and then washed with sterile distilled water. Plastic pots (72) with a diameter of 11 cm were filled with rice field soil. Ten seeds were planted in each pot and the pots were watered and placed inside the greenhouse. The air temperature inside the greenhouse during the growth and development of seedlings was in the range of 27–34 °C during the day and 17–20 °C at night, and the relative humidity was between 80 and 100%. During this period, irrigation was done as usual, so that the pots were permanently flooded. When the seedlings reached the four-leaf stage, they were inoculated with fungal spores. For this purpose, sterile distilled water was sprayed on all seedlings by hand sprayers; then, the spore suspension necessary for inoculation was prepared and sprayed on the plants.
To prepare the suspension, the spores were used as an inoculum. A hemocytometer was used to count the spores, and for inoculation, a suspension containing 5 × 105 spores in 100 mL of distilled water was used for P. oryzae, 108 spores for Trichoderma spp., and 2.5 × 105 spores for the other fungi investigated in this experiment. In addition, Tween-20 was used at a concentration of 1% to increase the surface absorption of the plant (Safari Motlagh et al., 2005). In each set, there were three pots for control plants that were inoculated only with distilled water (negative control). Three other pots included control plants inoculated solely with P. oryzae (positive control) and, finally, three pots were used for plants inoculated with both P. oryzae and the studied fungus. Inoculation of the studied fungi was done immediately after the inoculation of the pathogen.
Before the start of sporulation, the seedlings (treatments and controls) were separated from the rest of the pots by means of transparent plastics. The air relative humidity was kept above 95% after inoculation, and the temperature was in the range of 28–32 °C during the day and 20–24 °C at night. Evaluation of the symptoms 24 h after inoculation was done in order to describe them, but the final evaluation was done 10 days after inoculation using the Horsfall–Barratt scale (Horsfall & Barratt, 1945) to determine the severity of the disease with the following formula:
where: N is the leaf number in each disease degree (Bertrand & Gottwald, 1986; IRRI, 2013; Safari Motlagh & Mohammadian, 2016).
Finally, biometric traits, such as the height, fresh and dry weights of rice plants (including roots) affected by the disease-agent fungus alone and along with the studied endophytic fungi, were measured (Ghorbani et al., 2000).
Data analysis
The experiments were established in a completely randomized design (CRD) with eight treatments and three replicates for each treatment. The data underwent analysis of variance (ANOVA), and the means were compared using the least significant difference (LSD) test with a significance level of p < 0.05 using the SAS 9.3 (SAS Institute) statistical software.
. Results
Identification of fungal isolates
After the preliminary morphological identification of all fungal isolates at the genus level, 30 isolates that did not cause disease in the pathogenicity test on rice (according to Koch’s principles) were further evaluated and identified at the level of species. The outcomes of the examination of the endophytic fungal isolates, involving comparisons of DNA sequence similarities within the NCBI database and the construction of phylogenetic trees using the neighbor-joining technique, revealed that the isolates were related to Alternaria citri (Penz.) Mussat (three isolates), Alternaria infectoria Simmons (three isolates), Aspergillus fumigatus Fresen (six isolates), Fusarium verticillioides (Sacc.) Nirenberg (three isolates), Trichoderma harzianum (Mill., Giddens & Foster) Arx (four isolates), Trichoderma virens (four isolates), Trichoderma viride Pers. (four isolates), and Ulocladium consortiale (Thum) Simmons (three isolates) (Figure 1).
Figure 1
Phylogenetic tree resulting from the ITS-rDNA sequence analysis with the neighbor-joining method represents the location of the searched isolate in the vicinity of the cluster related to: (A) Alternaria citri; (B) Alternaria infectoria; (C) Aspergillus fumigatus; (D) Fusarium verticillioides; (E) Trichoderma harzianum; (F) Trichoderma virens; (G) Trichoderma viride; (H) Ulocladium consortiale.
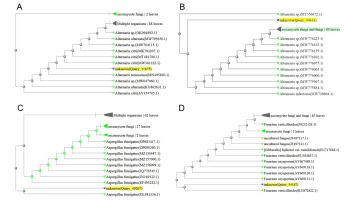
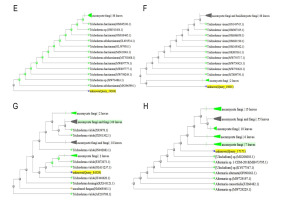
Pathogenicity test and biological control studies
The following isolates were used in the biological control trials: isolate A-3-27 from A. citri, isolate A-2 from A. infectoria, isolate 87S-3-1 from A. fumigatus, isolate 129S-5 from F. verticillioides, isolate S′-3-2-68 from T. harzianum, isolate S-3-2 from T. virens, isolate S-3-1 from T. viride, isolate 65S-1-2 from U. consortiale, and isolate P-729 from P. oryzae.
Dual culture, culture filtrate, and volatile metabolites tests
Based on the variance analysis of the pathogen’s mycelial growth inhibition percentage, there was a significant difference between the efficiency of the tested fungi at the 5% probability level. According to the LSD test in all three in vitro methods (dual culture, culture filtrate, and volatile metabolites), T. harzianum showed the highest percentage of mycelium growth inhibition in P. oryzae (51.25–56.25%). T. virens, T. viride, A. fumigatus, A. citri, U. consortiale, A. infectoria, and F. verticillioides were ranked next (Table 1). Accordingly, there was no significant difference between the species of the Trichoderma genus (except for the dual culture test, where T. viride was less effective than T. harzianum), but a significant difference was observed between T. harzianum and the other tested fungi (Table 1).
Table 1
Comparison of mycelium growth inhibition of P. oryzae in the dual culture, culture filtrate, and volatile metabolites methods.
Slide culture test
The mycelia of the Trichoderma species did not twist around the mycelium of the pathogenic fungus and were not capable to penetrate into it. The mycelium of F. verticillioides penetrated the mycelium of P. oryzae, but it was not able to change its shape. On the other hand, the mycelium of Ulocladium, Alternaria, and A. fumigatus, after reaching and penetrating the mycelium of P. oryzae, caused the disintegration of the pathogenic mycelium.
Greenhouse studies
The control plants that were treated with sterile distilled water displayed no symptoms of the disease. On the other hand, in the rice plants that were inoculated with P. oryzae, the first symptoms appeared three days after inoculation in the form of pinpoints, which gradually expanded on the following days and became spindle-shaped necrotic spots in the middle. They were gray or white in color and brown in the margin. In some cases, larger spots were observed along the length of the leaves. In rice plants that were inoculated with P. oryzae and one of the antagonistic fungi studied in this research, the first symptoms appeared five days after inoculation in the form of white spots and gray to brown pinpoints on the leaf surface. The number of these brownish-gray spots was lower than in the plants inoculated with P. oryzae only.
The average disease severity of P. oryzae isolates in the presence and absence of the fungi used in the experiment is presented in Table 2. According to the results obtained from the greenhouse investigations, all the studied isolates were able to reduce the severity of blast disease. Trichoderma harzianum was the most efficient by reducing the average severity of rice blast disease to 3.85 (27.36% reduction). Trichoderma virens, T. viride, A. fumigatus, A. citri, U. consortiale, A. infectoria, and F. verticillioides ranked next (Table 2). The effect of various treatments on the development of rice blast disease is shown in Figure 2. Moreover, significant differences were observed between the treatments in terms of such traits as plant height, fresh weight, and dry weight.
Table 2
Comparison of reduction in rice blast disease severity in greenhouse conditions.
Figure 2
Symptoms of rice blast disease in the greenhouse after inoculation with the spore suspension of P. oryzae (A); P. oryzae and A. fumigatus (B); P. oryzae and A. citri (C); P. oryzae and A. infectoria (D); P. oryzae and F. verticillioides (E); P. oryzae and T. harzianum (F); P. oryzae and A. infectoria (G); P. oryzae and T. virens (H); P. oryzae and U. consortiale (I).
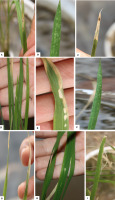
Plants treated with T. harzianum, T. virens, T. viride, A. fumigatus, and A. citri were the tallest and had highest dry and fresh weight that did not differ from the negative control (treated only with distilled water). Lower values of these parameters were found, in most cases, in plants inoculated with A. infectoria, U. consortiale, and F. verticillioides, although even these experimental objects were of better quality than the positive control treated only with the pathogen (Table 3). Based on the mean comparison of the height as well as the fresh and dry weight of the plants inoculated with the tested fungi with the control, it was determined that all of the studied fungi were able to increase the biometric parameters of infected rice.
Table 3
Comparison of biometric traits affected by antagonistic fungi in rice.
. Discussion
Harnessing the potential of endophytes emerges as a promising strategy for controlling rice diseases, providing a natural and ecologically sound alternative to traditional methods of disease management (Malinowski & Belesky, 2019). In this study, fungal isolates were collected from O. sativa plants from rice fields in Guilan province, Iran, and after preliminary identification at the genus level, they were used for pathogenicity studies. The pathogenicity of all isolates of P. oryzae (33 isolates) was confirmed. Among the fungal isolates used in the laboratory tests, T. harzianum, T. virens, T. viride, and A. fumigatus inhibited the mycelial growth of P. oryzae to a greater extent than U. consortiale, A. citri, A. infectoria, and F. verticillioides. In all three in vitro methods used in this research, i.e., dual culture, culture filtrate, and volatile metabolites, T. harzianum was the most effective endophyte in inhibiting the mycelium growth of the pathogen, which is in agreement with the findings reported by Javadi et al. (2014) and Safari Motlagh and Usefipoor (2016), who showed that T. harzianum significantly inhibited the mycelium growth of P. grisea in laboratory trials.
The isolates used in all the laboratory methods were effective in the biological control of the pathogen, especially fungi of the Trichoderma genus. The effectiveness of the isolates was the highest in the volatile metabolites test, which is consistent with the findings described by Safari Motlagh and Mohammadian (2016). Therefore, it can be concluded that the effectiveness of fungal isolates, such as T. harzianum and T. virens, in the biological control of the pathogen is due to the release of a significant amount of volatile gas compounds by these isolates. The consistent performance of T. harzianum across all three in vitro methods reinforces its potential as a potent antagonist against P. oryzae.
The mycelia of the three species of Trichoderma did not twist around the mycelium of the pathogen and were not capable of penetrating its mycelium, which is similar to the findings shown by Javadi et al. (2014). These observations additionally confirm that the mechanism of biological control by the most effective Trichoderma species in this study must be due to the production of chemical compounds. According to Kumari et al. (2023), many antimicrobial compounds that belong to various structural groups like terpenoids, steroids, quinines, and phenols can be isolated from endophytes. Trichoderma species are known for their ability to produce antifungal metabolites and enzymes that can inhibit the growth of pathogenic fungi (Stracquadanio et al., 2020). Contrastingly, F. verticillioides demonstrated mycelial penetration into the mycelium of P. oryzae, although it did not induce a change in the shape of the pathogenic fungus. This suggests a limited antagonistic effect, possibly involving competition for nutrients or space, which is in agreement with the low efficiency of this endophyte in the in vitro tests. On the other hand, the other fungi used in this study, especially Ulocladium and Alternaria species, exhibited a more aggressive mode of action and caused structural changes in them, which is in agreement with the reports provided by Hajano et al. (2012). The observed mycoparasitic and disruption effects could be attributed to the production of lytic enzymes or other antifungal metabolites by these endophytes (Witkowska & Maj, 2002).
The comparison of the results of biological control studies at the laboratory and greenhouse stages revealed that the T. harzianum and T. virens isolates showed the highest efficiency in both. On the other hand, F. verticillioides was at the lowest rank of usefulness in blast disease control, which is consistent with the findings reported by Safari Motlagh and Mohammadian (2016), who concluded that this fungus does not have much ability to control rice brown spot disease. The order of usefulness of the other isolates used in the current research in the laboratory and greenhouse was the same. In a study carried out by De Figueirêdo et al. (2010), biological control of Sclerotinia sclerotiorum using eight isolates of Trichoderma spp. and an isolate of Ulocladium atrum was investigated. With the exception of U. atrum, all Trichoderma isolates showed good antagonistic potential against the disease-causing fungus, and isolate 3601 had the greatest effect, which is in agreement with the findings of this research in terms of the comparison of the effect and efficiency of Trichoderma and Ulocladium isolates. The low efficiency of some isolates in controlling the disease in greenhouse conditions may be due to various factors, including non-living environmental parameters, such as soil type, soil temperature, and water potential, and living environmental parameters, such as plant cultivar, diversity and soil microbial activity, method and time of using fungal isolates etc. (Akrami et al., 2011). Moreover, previous studies have shown that the type of inoculum also affects the antagonistic ability of fungal isolates. For example, Verma et al. (2007) reported that Trichoderma species applied in the form of spores (especially conidia), which are resistant to unfavorable environmental conditions in the field, are much more effective when used as propagules in mycelium forms. For this reason, the conidia of antagonistic endophytes were used to prepare the inoculum in the present study.
In our study, A. fumigatus showed the highest ability to control the disease agent, after Trichoderma species, both in the laboratory and in the greenhouse, which was in accordance with the study conducted by Cook and Baker (1983). These researchers also reported the effectiveness of Aspergillus species, e.g. A. niger, in the biological control of rice leaf sheath blight. In the present study, A. citri showed a good ability to control the pathogen both in the laboratory and the greenhouse, which is consistent with the findings shown by Safari Motlagh and Mohammadian (2016). These researchers reported the usefulness of the aforementioned fungus in the biological control of Bipolaris victoriae, the causal agent of rice brown spot disease in the laboratory and the greenhouse, and it was found that this fungus reduces the severity of this disease.
In the investigation of such traits as the height, fresh weight, and dry weight of rice plants, all of the fungi used had the capability to increase the yield of the plants compared to those infected only with the pathogen. Researchers showed that various species of fungi, such as Trichoderma, stimulate the plant’s defense system and its growth (Guzmán-Guzmán et al., 2023). This may be related to the common genes that control these traits. Fungi have also been used as enhancers of plant defenses, as they can trigger systemic resistance, safeguarding plants from various pathogens and ultimately resulting in heightened crop yields (Guzmán-Guzmán et al., 2023). In this context, fungi have played significant roles in promoting plant growth and increasing agricultural output (Phour et al., 2020). The results of the current experiment provide valuable insights into the correspondence between taxonomic assortment and ecological functions, particularly in the context of plant-microbe interactions and disease management. Trichoderma harzianum emerged as the most effective isolate in reducing the severity of blast disease (by 27.36%) in greenhouse conditions, which is in line with the findings reported by Javadi et al. (2014). This highlights the importance of specific taxonomic groups in providing effective biological control against plant pathogens.
. Conclusions
According to our results, T. harzianum, T. virens, and T. viride are the most effective fungal isolates in the control of P. oryzae in both laboratory and greenhouse conditions. The effectiveness of specific fungi in reducing disease severity and enhancing plant growth highlights the importance of understanding the ecological roles of microorganisms for sustainable and integrated disease management strategies in agriculture. The present study showed that the isolation and identification of fungi in the natural flora of rice plants and the study of their antagonistic effects offer a sustainable and environmentally friendly approach to rice blast disease control. The studied biocontrol agents can be considered an important component in the integrated management of this severe disease (not only by reducing the damage caused by the pathogen, but also by increasing the crop yield in surface unit) and in preserving the biological reserves of the environment.