. Introduction
Plant species that can be economically exploited and therefore have high productivity, make efficient use of solar radiation, have low habitat-agrotechnical requirements, and show high resistance to adverse environmental conditions deserve special attention (Sumi et al., 2022). Furthermore, a greater diversity of cultivated species is a key to ensuring food security and economic sustainability, especially in the face of the ongoing climate change (Dzida et al., 2023; Wongla et al., 2023). Increasing biodiversity prevents agroecological imbalances and reduces the spread of pests that move easily and are difficult to control, especially in large areas of agricultural monocultures (Tyburski & Kostrzewska, 2013). Increasing crop diversity is also important for the conservation of biodiversity in agricultural ecosystems and for the sustainable use of biological resources ensuring sustainable economic development. This indicates the need for research into the possibilities of introducing new or little-known plant species into cultivation (Tylkowski & Grupa, 2008).
Lavatera thuringiaca L. (Thuringian mallow) is a little-known endemic species, although it is commonly found in the wild in central European countries and southern Scandinavia (Celka et al., 2013; Ray, 1995; Szafer et al., 1996). So far, there has been little interest in the cultivation of this species despite the possibility of its multi-directional use in many areas of economy and its capability of growing in adverse environmental conditions. This perennial plant adapts well to different environmental conditions, has low water demands, and is frost-resistant. According to different authors, it can be cultivated as a perennial plant suitable for production of high-performance energy biomass (Romanowska-Duda et al., 2014; Staszewski et al., 2008), a supplement for plant feed for ruminants (Staszewska, 1997), a raw material for the pharmaceutical industry (Matławska et al., 1999; Vadivel et al., 2016; Vysochina et al., 2011), a honey plant for bees to be cultivated on wastelands and uncultivated fields (Staszewski & Staszewski, 2014), an ecological plant to be sown on roadsides and used in recultivation of degraded land (Klimont et al., 2013), a high-value ornamental plant to be grown in gardens and parks on dry soils (Staszewska, 1997), and as a food crop, in the production of pigments, and raw material in the production of strings and ropes (Celka et al., 2013).
The literature on L. thuringiaca consists mainly of natural history publications on the botany and occurrence of the species in different regions and habitats, while reports on the economic use of the species are sporadic, scattered, and fragmentary. The collected material was organized and collated in the article to present the characterization of this species in terms of its biological, biochemical, and genetic properties and potential for economic use. The literature review was based on data collected mainly from Google Scholar, Web of Science, and ScienceDirect databases and older Polish research results. The gathered literature covered original research articles, reviews, book chapters and, in a few cases, conference proceedings.
. Botanical characteristics
A total of 25 different species of Lavatera can be found in the Malvaceae family, with the majority of growing in Mediterranean climates (Zhandabayeva et al., 2021). L. thuringiaca is a species of the Lavatera family (Malvaceae) naturally occurring in the Adriatic Sea zone to central Siberia, through central Europe, and southern Scandinavia (Celka et al., 2013; Ray, 1995; Revenga et al., 2000).
L. thuringiaca plants generally reach a height of 80–150 cm but can grow up to 220 cm in favorable environmental conditions (Figure 1). The stems are thick, stiff, erect, and profusely leafy, protecting the canopy from lodging and guaranteeing a stable yield. After mowing, numerous new well-leaved stems emerge to enable the second crop to be harvested (Figure 2). In the second and subsequent growing years, the plants produce a massive short stem, from which thick, white, fleshy roots covered by a light grey skin emerge. A single crown can produce more than 20 main shoots (Figure 3), on which numerous 1st and 2nd order branches emerge. The intensity of tillering is negatively correlated with the density of plants per unit area (Staszewski & Staszewska, 1994). The taproot system is strongly developed and goes deep. The leaves are palmate and, like the stem, covered by hairs on the underside.
Figure 3
Branching of a single plant of Lavatera thuringiaca in the second year of vegetation (photo: Z. Staszewski).
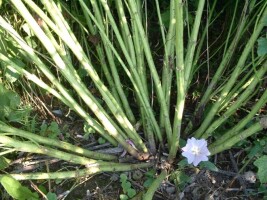
L. thuringiaca is a self-pollinating plant producing a large number of hermaphroditic pale pink flowers 5–8 cm in diameter with darker veins. They are borne singly at the angles of the leaves on the main stem and numerous branches, and each produces a fruit (schizocarp) containing about 20 seeds. The phenomenon of precociousness in flower development is characteristic here: the anthers, gathered in a whorl, are the first to mature, while the pistil grows among them a little later and is fertilized with its own pollen before the arrival of insects (Staszewska & Jabłoński, 1998). This phenomenon does not reduce insect abundance. The bud setting and flowering period in L. thuringiaca is relatively long, lasting from mid-June to early September, with most flowers produced in July. The seeds are kidney-shaped, smooth, grey to dark brown with an TSW of 3.6 g (Staszewski & Staszewska, 1994). Thanks to the self-pollination of the plants, the seed production of L. thuringiaca is very efficient. A single plant may produce up to 41,000 seeds per year (the individuals in question produced on average 3,200 seeds per year) (Celka et al., 2013). Depending on the planting density, 300 to even 600 kg of seeds can be obtained from 1 ha of plantation (Staszewski & Staszewska, 1994). The seeds contain about 18% of oil with dominant linolenic acid, which can be used in the production of medicines and cosmetics.
In the first year, the plants grow half the size but flower and set seed. In later years, shoot buds emerge at the beginning of spring; their vigorous growth begins in May and continues until the end of flowering. In the second and third growing years, L. thuringiaca can yield 22.7–27.1 tonnes of dry matter per hectare. It yields better than e.g., Helianthus annuus L. (15.6–16.1 tonnes of dry matter per 1 ha) (Staszewski & Staszewska, 1994).
An advantage of this plant is the efficient production of seeds, which unfortunately have a low germination capacity in natural growing conditions caused by the high proportion of hard seeds that do not swell, because the seed coat does not allow water to pass through. The proportion of hard seeds in the total seed pool of L. thuringiaca harvested in different years can be up to 80–90% (Grzesik et al., 2015; Pawlat et al., 2017). A common treatment to facilitate hard seed germination is scarification, which involves damaging the seed coat and causing them to swell when water is available (Figure 4). A study conducted by Grzesik et al. (2015) indicated the possibility of increasing the germination of L. thuringiaca seeds up to over 40% with mechanical scarification (rubbing the seeds between sandpaper) and up to 85% after exposure to sulfuric acid. In order to improve the seed quality, physical factors such as electric, laser, and magnetic fields or plasma are also used. Studies found that the exposure of L. thuringiaca seeds to He–Ne laser light had a beneficial effect on field emergence capacity and biometric characteristics (Budzeń et al., 2015), seed germination (Budzeń et al., 2018), and the chemical composition of biomass ash (Budzeń et al., 2023). Pawlat et al. (2017) proved that pre-sowing plasma stimulation of seeds improved germination parameters, such as germination capacity and germination energy. In turn, Kornarzyński et al. (2015) showed a positive effect of an alternating electric field and magnetically treated water on the germination of parental and second-generation Lavatera seeds.
L. thuringiaca is adapted to grow on poor sites. It is characterized by high durability, frost hardiness, and competitiveness with other plant species. It tolerates drought conditions but does not tolerate excessive soil moisture and flooding (Vazquez-Thello et al., 1995). In the wild, it is often found on dry hillsides, scrub, and roadsides. According to the breeder (Staszewski & Staszewska, 1994), the cultivation technology of L. thuringiaca is easy and low-cost. Establishing a plantation requires careful cultivation because L. thuringiaca has small seeds and is exposed to competition from weeds during the initial growth period. It grows well on various types of soil. The best forecrops are fodder and root or industrial plants, but they can also be cereals. The seeds are sown with a grain seeder to a depth of 1–2 cm, and the sowing density and row spacing depend on the direction of use of the plantation. When growing for seeds and bee nectar, a population density of approx. 20 plants per 1 m2 is used, maintaining a row spacing of 30–60 cm, for the production of energy biomass the recommended density is 30–40 plants per 1 m2 with a row spacing of approx. 30 cm, and for the production of green fodder or for sowing fallow lands, it is recommended to have approximately 40 plants per 1 m2 in rows every 20–40 cm. Sowing should be done in early spring, because the plant requires a lot of moisture for good germination. L. thuringiaca requires protection against weeds in the initial period after sowing, but when the plants grow and develop well, their competitiveness in the field increases very quickly. It is quite resistant to diseases and pests and generally does not require protection. Its fertilizer needs are similar to those of Althaea rosea Cav. (kg ha−1): N – 60–80, P – 60–90, K – 100–120. Harvesting should begin when the seeds grow to their maximum size and turn yellow and even slightly brown. Harvesting is carried out in one phase, using a combine harvester. Delaying the harvest too much causes large losses when the seeds scatter. The Lavatera plantation is used for approximately 5 years or longer (Staszewski & Staszewska, 1994).
. Raw material for energy purposes
All EU member states are committed to reducing greenhouse gas emissions mainly by replacing traditional transport fuels with biofuels and coal with biomass (Hoogwijk et al., 2009). Every tonne of biomass used in energy production reduces air pollution by 1.5 t of carbon dioxide that was taken up in photosynthesis to produce it. This is because biomass is a renewable fuel, and this means that the CO2 released during the biomass combustion process is taken up by other plants and converted into new biomass (Yemshanov & McKenney, 2008). Growing energy crops on agricultural land is seen as one of the most important potential sources of biomass (Rosenqvist & Dawson, 2005). In addition, compared to conventional agricultural crops, energy crop plantations have a better energy and carbon balance, higher soil carbon sequestration, less nitrogen leaching, better phytoremediation properties, and similar or higher plant, invertebrate, and bird biodiversity.
Plants used for energy crops should be characterized by efficient conversion of solar radiation into biomass and large annual growth under minimal habitat requirements and good adaptation to the climate conditions. As a perennial crop, L. thuringiaca can be cultivated for energy purposes, as it produces a relatively high biomass yield in different environmental conditions and affects the structure and fertility of the soil (Romanowska-Duda et al., 2014). Depending on the habitat conditions and the level of fertilization, L. thuringiaca harvested once a year yields 15–25 t ha−1, and when harvested twice, the yield is higher at 23.0–27.0 t ha−1 (Staszewski et al., 2008; Staszewski & Staszewski, 2014), which is a good result, compared to other popular energy crops such as (t ha−1) Salix spp. (13.0–18.0 in an annual cycle, 19.0–22.8 in a 3-year cycle) (Matyka, 2013; Szczukowski, 2012), Sida hermaphrodita (L.) Rusby 8.2–18.5 (Majtkowski et al., 2010; Matyka, 2013), or Miscanthus ssp. 21.0–29.9 (Kotecki, 2010; Matyka, 2013). The biomass has comparable energy parameters (calorific value, C, H, N, S, fixed carbon, volatile matter and ash content) to typical energy crops, e.g., S. hermaphrodita, Miscanthus, Helianthus tuberosus L., or Salix viminalis L. (Budzeń et al., 2021). As shown by Budzeń et al. (2016, 2021), the application of pre-sowing He–Ne laser light stimulation influences the energy parameters of L. thuringiaca biomass, namely it increased the heat of combustion and the calorific value of the grown shoots. Besides, an innovative method for improvement of the chemical composition of L. thuringiaca biomass ash was presented by these authors (Budzeń et al., 2023). They determined the effect of pre-sowing seed stimulation with He–Ne laser light on the chemical composition (P, S, K, Ca, Mn, Fe, Ni, Cu, and Zn content) of ash. It was shown that the pre-sowing application of He–Ne laser light, depending on the stimulation time, intensified the content of preferred macro- and micronutrient groups in L. thuringiaca ash, allowing it to be used more effectively in agriculture as a substitute for commercial fertilizers.
The wood of L. thuringiaca is chemically similar to that of deciduous trees and the ash content (6.5–7.0%) is similar to that in cereal straw. The calorific value is 14,218 kj/kg at moisture content of 8.33%, while the ash and sulfur contents are 3.90 and 0.28%, respectively, which qualifies this species as a valuable energy crop (Staszewski et al., 2008). The advantage of L. thuringiaca is the low cost of establishing a plantation, compared to other common crops, and the plantation establishment requirement is a few kg of seed per ha. For the cultivation of this species, machinery designed for cereals and oilseed rape is sufficient, so that the farmer does not have to incur costs associated with investing in additional equipment. A plantation of L. thuringiaca can be maintained for five years or even longer, given the easy seed dispersal and self-sowing of this plant, which renews the plantation. The L. thuringiaca biomass crop can be burned directly in a domestic furnace.
. Fodder crop for livestock
Research conducted by Staszewska (1997) showed that L. thuringiaca can be used for fodder purposes. Biomass harvested several times (3–4) per year (except for the year of sowing) yielded 10.0–15.0 t of dry matter per hectare, providing forage with high content of protein and water-soluble sugars. The dry matter content of the crop was around 21–25%. The crude protein content in L. thuringiaca green forage and silage was 20.7 and 23.7%, respectively, and the NDF (neutral detergent fiber) and ADF (acid detergent fiber) fractions were 19.3, 19.2% and 13.0, 14.7%, respectively (Table 1). The silage was characterized by good indices of biological value and protein utilization by animals, and the nutrient digestibility coefficients (except for crude fiber) were similar to those of other forages of this type. This species can also be ensiled with other forage crops. The study showed that the addition of Dactylis glomerata L. slightly increased the protein value of the silage (by about 4%) and significantly increased the fiber fraction of NDF (by 41%). Ensiling with Medicago sativa L. increased the protein content (by 17%) and only slightly the NDF content (by 6%). In contrast, silage with H. tuberosus had significantly lower protein content (by 52%) and slightly higher NDF content (by 6%). All silages had adequate color, texture, odor, and biological value (Staszewska, 1997).
Table 1
Chemical content of green fodder and silage (Staszewska, 1997).
To date, there has been little interest in L. thuringiaca as a forage crop, but there is now more interest in introducing new crop species, in particular those with low soil and water requirements, especially on extensive farms with a high proportion of light soils.
. Forage plant for honey bees
L. thuringiaca is a good melliferous plant, as it provides nectar to bees over a period of 4–6 weeks. Abundant flowering lasts for about 30 days and flowers persist for about 6 weeks on the tops of the stems (Staszewski & Staszewska, 1994). The flowers are visited mainly by honey bees and, to a lesser extent, by bumblebees. Pollinators only collect nectar, because they cannot form pollen baskets from very large pollen grains visible to the naked eye (Kołtowski, 2015). The frequency of honey bees visiting the flowers is very high because the nectar of L. thuringiaca is of good quality and contains a lot of sugar. During the day, it secretes up to 4.8 g of nectar per flower (Iriondo & Pérez, 1996). In terms of honey yield, it can be compared to buckwheat (150–200 kg of honey per hectare) but is more reliable than buckwheat because it produces nectar even in dry weather due to the fact that its nectaries are sheltered (Staszewska & Jabłoński, 1998). L. thuringiaca honey is characterized by its light color, pleasant aroma, and exquisite taste. It contains 82.0% of reducing sugars, 2.2% of sucrose, and 17.7% of water (Table 2). Besides biomass, honey harvesting is an additional benefit that can increase the value of L. thuringiaca production.
Table 2
Parameters of Lavatera thuringiaca honey (Staszewska, 1997).
Indicator | Unit | Content |
---|---|---|
Water | % | 17.70 |
Carbohydrates | % | 81.92 |
Saccharose | % | 2.18 |
Acidity | ml 1 N NaOH 100 g−1 | 2.70 |
Conductivity | 10−4 S cm−1 | 3.40 |
Diastase number | 21.13 | |
HMF* | 0.05 |
Jabłoński (2000) reports on the advisability of establishing the so-called bee pastures by sowing perennial species of melliferous plants that provide “bee nectars” on fallow and devastated lands. According to Klimont (2007), the following species are suitable for perennial bee pastures: Solidago gigantea Aiton, Echinops exaltatus Schrad., L. thuringiaca, Agastache anethiodora (Nutt.) Britton, and Trifolium repens L. The results of research conducted by Klimont et al. (2013) indicate that L. thuringiaca belongs to melliferous species characterized by high abundance and are additionally useful for biological reclamation of areas after sulfur exploitation. In addition to the utilization of post-industrial and domestic waste, thanks to such species it is possible to recreate the plant cover on devastated sites, obtain bee nectar (nectar, pollen), and additionally achieve an aesthetic effect for the surroundings.
. Management of marginal soils and reclamation
Land devastated by human management requires reclamation, which helps to restart soil-forming processes, initiate biological life in the subsoil, and restore the vegetation cover. Plant species suitable for reclamation should be characterized by low sensitivity to soil compactness, pH, nutrient abundance, and content of harmful substances. According to Klimont et al. (2013), of the 45 perennial crop species studied, L. thuringiaca proved to be one of the most suitable plants for biological reclamation of land after sulfur mining due to its high plant vigor assessed by the density, uniformity, and abundance of the canopy overgrowing the substrate. L. thuringiaca spreads well, creates a dense, high canopy, and suppresses plants of other species (weeds). It has a deep pile root system strengthening the soil. In addition, the authors showed that the species had a beneficial effect on the chemical properties of the substrate by increasing the content of organic matter and available nutrients, serving as food for bees, and improving the aesthetics of the environment.
L. thuringiaca was also useful for the reclamation of nutrient-poor post-flood lime fertilized with sewage sludge (Klimont, 2007). The study showed that, of the 25 species of melliferous plants, L. thuringiaca had the highest exuberance on this substrate, along with Solidago gigantea Aiton, Solidago canadensis L., Eryngium planum L., Anchusa officinalis L., and Agastache foeniculum Kuntze. Cultivation of these plants had a beneficial effect on the chemical properties of the sludge-enriched lime, increasing the organic matter content in the forming humus layer from 0.86% in the control to 4.04%. In turn, the nutrient content in the sorption complex increased from 1.2 P2O5, 2.1 K2O, and 1.2 Mg mg 100 g−1 of soil in the control site to 7.2, 4.3, and 4.2 mg 100 g−1 of soil, respectively (Klimont, 2007).
An additional possibility for the use of L. thuringiaca can be the management of wasteland, fallow land, and soils excluded from cultivation (Staszewski & Staszewski, 2014). The species is persistent, easily bushy, and has a dense canopy that successfully competes with weeds. In unmanaged plantations, mature seeds are easily self-sowing seeds providing regrowth, which can take decades. Thanks to this, the plantation reproduces itself. Its deep taproot system also makes the species suitable for strengthening slopes and embankments.
L. thuringiaca can act as a cover crop to help stop soil erosion in poor quality areas. In addition, its roots can improve soil structure by creating a root network, which is important especially in heavy soils. Its low water and soil requirements and good resistance to cold help Lavatera adapt to diverse environmental conditions and contribute to a lower irrigation and fertilization effort (Vazquez-Thello et al., 1995).
The plant can be also used to create hedges, which can not only improve the aesthetics of the area, but can also serve a protective function. It is also an ornamental plant, often used on farms. Thanks to its beautiful flowers and green leaves, it can be an aesthetic element in areas that require visual improvement.
. Phytochemical constituents
L. thuringiaca has pronounced antioxidant, antimicrobial (Kalayou et al., 2012), anti-inflammatory (Silva et al., 2014), antibacterial (Mašković et al., 2013), cytotoxic (Mašković et al., 2018), and antitussive (Tuzlaci et al., 2010) effects and other types of pharmaceutical activity (Mašković et al., 2018). Due to the high favorable content of phenolic compounds, it is applied in the pharmaceutical industry as a medicinal plant (Matławska et al., 1999). Zhandabayeva et al. (2021) present the composition of L. thuringiaca components that have a certain antibacterial effect. In the composition of thick CO2 L. thuringiaca extract, the content of 31 components was proven: α-bisabolol oxide B (9.65%), linolenic acid (9.38%), bisabolol oxide A (8.26%), cis-β-farnesene (7.63%), spathulenol (6.97%), linoleic acid (6.95%), herniarin (5.61%), pulegone (5.08%), ethyl ether (3.15%), elaidic acid (2.57%), phytol (2.49%), myristic acid (2.33%), verbenone (1.93%), and α-bisabolol (1.36%). Antimicrobial activity studies have shown that the CO2 extract of L. thuringiaca has a pronounced effect against clinically significant microorganisms: Escherichia coli, Staphylococcus aureus, Candida albicans, Pseudomonas aeruginosa, Klebsiella pneumoniae, Streptococcus pneumonia, Staphylococcus haemolyticus, and Staphylococcus saprophyticus.
The presence of kaempferol 3-O-β-glucoside, kaempferol and quercetin 3-O-rutinosides, cis- and trans-tiliroside, and p-coumaric acid was identified in the flowers of L. thuringiaca (Matławska et al., 1999). Besides, the species contains 5 glycosides in flowers and 4 glycosides in leaves. The most common aglycones are quercetin, kaempferol, and apigenin (Matławska, 1990). This result fits well with the view that p-coumaric acid and kaempferol glucoside may occur regularly in the flowers of Lavatera. Fedoseeva and Myznikova (2017) identified derivatives of caffeic and chlorogenic acids and umbelliferone in the roots of L. thuringiaca, chlorogenic acid, flavonoids of the flavone and catechin groups, and coumarin compounds in the grass and stems, chlorogenic and ferulic acids and derivatives of quercetin (quercitrin, etc.), kaempferol, and flavone in the leaves, and phenologlycosides and kaempferol and flavone derivatives in the flowers.
L. thuringiaca plants have been analyzed by Vysochina et al. (2011) for the content of low-molecular biologically active compounds, such as flavonoids, phenolic acids, tannins, and coumarins. Their results showed that L. thuringiaca contains high amounts of coumarins and 1.3% of flavonoids. Coumarins are valuable natural products. The increased interest in these chemical compounds is related to the high physiological activity of many derivatives of coumarin and furocoumarins, which have antitumor activity. Natural coumarins and furocoumarins also have antibiotic, antifungal, antispasmodic, and insecticidal properties and exhibit anticoagulant, sedative, anticonvulsant, and vasodilating effects (Raters & Matissek, 2008). Lavatera species have been studied for their flavonoid content also by Skalicka-Wozniaka et al. (2007) and Razavi et al. (2011). Flavonoid compounds exhibit strong antioxidant activity (Rice-Evans et al., 1997). Many factors such as genotypes, organ, physiological stage, and growing conditions (soil, agronomy, and climate) have been reported to have a significant effect on phenolic acids and antioxidant properties of plants (Carbone et al., 2011). The phytochemical constituents of L. thuringiaca and its activities are presented in Table 3.
Table 3
Phytochemical constituents of Lavatera thuringiaca and their activities.
Phytochemical constituents | Class | Activities | References |
---|---|---|---|
Isopulegone, Piperitone, E-Nerolidol, Caryophyllene oxides and E-Nerolidol | Monoterpenoid | Anticonvulsant, anti-inflammatory, antioxidant, gastroprotective, anti-stress, antimicrobial, antiviral, and perfume compositions | (Shahverdi et al., 2004; Silva et al., 2007, 2009a, 2009b) |
D-Menthol, Humulene, Caryophyllene oxide, α-Bisabolol, Phytol | Terpene | Pain-reliever, appetite suppressant, anti-irritant, anticancer, analgesic, antifungal, anti-inflammatory, sedative, anti-irritant, antimicrobial, and analgesic | (de Cássia Da Silveira e Sá et al., 2015; de Chavan et al., 2010; Kim et al., 2011; Laude et al., 1994; Nguyen et al., 2017; Santos et al., 2013; Willis et al., 2011; Zhandabayeva et al., 2021) |
Alloaromadendrene, α-Bisabolol oxide A & B, Cis-β-farnesene, c-Muurolene, Isocaryophyllene, α-Curcumene | Sesquiterpene | Antifungal, anti-irritant, anti-inflammatory, antimicrobial, and antioxidant | (Darwish et al., 2020; Forrer et al., 2013; Kim et al., 2011; Nogueira et al., 2021; Yu et al., 2014) |
Verbenone, Pulegone, Thymol | Monoterpene | Antinociceptive, antimicrobial, anticonvulsive, antiseptic, antibacterial, and antifungal | (de Melo et al., 2017; de Sousa et al., 2011; Hu et al., 2017; Santoyo et al., 2014) |
Spathulenol | Sesquiterpenoid | Antimycobacterial, antiproliferative, anti-inflammatory, and antioxidant | (do Nascimento et al., 2018) |
Palmitic acid, Ethyl ester, Linolenic acid, and Isovaleric acid | Fatty acid | Anti-inflammatory, promitogeinc and, stimulating effects on HSC (hepatic stellate cells), anti-cancer, antibacterial and antifungal properties. Used to make validol, valocordins, and others | (Jalalvand et al., 2019; Kim et al., 2014; Kusumah et al., 2020; Li et al., 2003; Saeed et al., 2012; Tu et al., 2019; Zhandabayeva et al., 2021) |
Myristic acid, Stearic acid, Elaidic acid | Saturated fatty acid | Antimicrobial, anti-cancer, and against herpes viruses | (Andrei et al., 2000; Chen et al., 2019; Habib et al., 1987) |
Herniarin or 7-methoxycoumarin | Coumarin | Antigenotoxic, anti-inflammatory, and antinociceptive | (Andrei et al., 2000; Cheriyan et al., 2017; Nayeli et al., 2020) |
Linoleic acid | Polyunsaturated fatty acid | Analgesic and bactericidal properties | (Kusumah et al., 2020; Tu et al., 2019; Zhandabayeva et al., 2021) |
Cannabidiol | Phytocannabinoid | Anticonvulsant | (Frías-Soria et al., 2021) |
Perhydrofarnesyl acetone | Diterpenoids | Perfume composition | (Zhandabayeva et al., 2021) |
Geranyl linalpol | Diterpene | Antimicrobial and anti-inflammatory | (Zhandabayeva et al., 2021) |
. Molecular research on Lavatera
Genetic studies uncover the structure and biological function of the plant genome (i.e., all genes) and its regulatory parts. The molecular basis of genes is deoxyribonucleic acid (DNA). The function of particular genes or DNA segments can be inferred by making associations between varieties in the appearance of different plants (for example, disease resistance) with their genetic differences. Genetic research also helps to analyze plant properties that are not based on individual genes but on gene networks. In this way, complex traits, such as yield and drought tolerance, can be tailored more precisely.
L. thuringiaca is able to survive at freezing temperatures. Its frost tolerant character may have potential use in the genetic improvement of other related plants for increased frost tolerance (Vazquez-Thello et al., 1995). The results reported by Vazquez-Thello et al. (1996) showed that the formation of somatic hybrids (fusion callus) between transgenic Hibiscus rosa-sinensis and transgenic L. thuringiaca (a cold-tolerant species) resulted in a significant improvement in cold tolerance over H. rosa-sinensis. The introduction by genetic transformation of chimeric marker genes encoding e.g., antibiotic or herbicide resistance enables the verification of hybrids at the earliest stage. Selection based on resistance to two antibiotics has been used to verify somatic hybrids of H. rosa-sinensis + L. thuringiaca (Vazquez-Thello et al., 1996). Vazquez-Thello et al. (1996) produaed transgenic L. thuringiaca plants by transformation of Agrobacterium tumefaciens LBA 4404 cell suspension carrying the binary vector pMM454 (Nos-Hpt). After 2 h co-culture, the cells were placed in solid media and the transformed loci were selected by somatic embryogenesis under selection control. A protoplast from a transgenic L. thuringiaca line was combined with protoplasts from a transgenic hibiscus in an attempt to transfer Lavatera cold tolerance to hibiscus. In addition, by selecting for resistance to kanamycin (from transgenic hibiscus protoplasts) and hygromycin (from transgenic Lavatera), somatic hybrids were isolated. In their study to identify intergeneric hybrids of H. rosa-sinensis and L. thuringiaca, Vazquez-Thello et al. (1996) used isoelectric focusing to distinguish allelic forms of the enzyme. Verification of the hybrids was performed based on the presence of marker genes introduced by genetic transformation. Inter- and intra-specific hybridization among different species and genera is one of the most important breeding methods for creating new and improved plants (Kuligowska et al., 2016).
Grant et al. (2001) and Grant and Culham (2001) carried out genetic analysis of perennial Lavatera cultivars, i.e., L. thuringiaca and Lavatera olbia (L.) Alef. The research involved investigating the parental origin of the cultivars and the role of hybridization in generation of Lavatera cultivars using artificial hybridization and randomly amplified polymorphic DNA (RAPD) markers. In addition, the ability of RAPD markers to distinguish cultivars with a narrow genetic base was also evaluated. Previous studies showed that RAPD were used widely to evaluate distinguishability and relatedness of genotypes in plants (Culham & Grant, 1999). The DNA extraction and RAPD polymerase chain reaction showed that species-specific molecular markers were identified from the RAPD profiles. Nine RAPD markers for L. thuringiaca were identified and showed that two bands (UBC 721 700 bp, UBC 792 900 bp) were unique only for L. thuringiaca. The identified molecular markers were used to determine the origin of the varieties. It was shown that ‘Ice Cool’ and ‘Candy Floss’ cultivars are derived from L. thuringiaca (Grant & Culham, 2001).
Tchórzewska et al. (2008) carried out analyses of karyokinesis, cytokinesis, and chondriokinesis occurring during meiosis in the process of microsporogenesis in dicotyledonous angiosperms, including L. thuringiaca. They demonstrated that, in L. thuringiaca, simultaneous cytokinesis occurs during microsporogenesis and organelles move in a characteristic manner, classified as envelope chondriokinesis (Tchórzewska, 2017; Tchórzewska et al., 2013). In this type of chondriokinesis, the autonomous oragnelles at the end of prophase I cluster around the nucleus and karyokinesis takes place in this confined space. Then, in telophase I, the envelopes of the organelles form around two nuclei, followed by four nuclei in telophase II. Such a position of the cell organelles is maintained not only until the end of meiosis, but also after this phase when the mononuclear pollen grains have already formed a sporoderm (Furness et al., 2002; Koç & De Storme, 2022). Subsequent analyses were focused on the configuration of the tubulin cytoskeleton in L. thuringiaca meiocytes. It turned out that the tubulin cytoskeleton in this species forms configurations differing not only from those observed in Psilotum nudum but also from those described in seed plants. The performed studies demonstrated new configurations of microtubules (MTs), which surrounded aggregates composed of organelles around the nucleus in the form of networks (in late prophase I and II). Moreover, an unusually strong perinuclear radial microtubule system occurring in telophase I and II in the species studied and the presence of a phragmoplast in a small space devoid of cell organelles between aggregates composed of cell organelles surrounding the progeny nuclei were observed. It was found that meiosis in L. thuringiaca microsporogenesis is similar to that occurring in most plants with simultaneous cytokinesis, but the movement of organelles during meiosis is very unique and specific (Brownfield et al., 2015; Marciniec et al., 2019). Microtubules were found to be closely associated with karyokinesis and chondriokinesis during microsporogenesis in L. thuringiaca, and thus determined the formation of functional pollen grains (Tchórzewska et al., 2008). Tchórzewska et al. (2008) described the role of both chondriokinesis and the tubulin cytoskeleton in meiotically dividing cells during microsporogenesis in species of the family Malvaceae. The research showed that the characteristic envelopes of autonomous organelles in the species studied divide the space within which karyokinesis occurs, replacing the cell wall that is still absent. On the other hand, the analyses of the tubulin cytoskeleton showed that microtubules were responsible not only for segregation of chromosomes but also for the characteristic rearrangements of cell organelles during meiosis (chondriokinesis). In this study, colchicine was used, which is an alkaloid that binds specifically to microtubules causing inhibition of their polymerization. The destruction of the tubulin cytoskeleton resulted not only in the absence of normal chromosome segregation but also in the absence of the movement of cell autonomous organelles typical of envelope chondriokinesis (Tchórzewska et al., 2008). For L. thuringiaca, Markova and Goranova (1993) reported chromosome counts of 2n = 44.
. Conclusions
The review highlights the biological and biochemical properties of L. thuringiaca and the potential for multipurpose use of this species. Its high production potential and favorable chemical composition allow it to be used for fodder purposes, and its abundant and long flowering makes it an excellent forage for bees and other pollinators. The biomass can also be used for energy purposes, as it has similar energy parameters to those of typical energy crops (e.g., Salix, Sida, Miscanthus). In turn, its high resistance to unfavorable environmental conditions (drought, frost) and low habitat requirements (especially soil requirements) mean that it can be used for the development of marginal soils and for the reclamation of land contaminated by industry. At the same time, the cultivation of this plant is economically advantageous due to the low cost of establishing the crop and the easy dispersal of seeds that renew plantations despite the high proportion of hard seeds. L. thuringiaca contains numerous biologically active compounds (including phenolic compounds); hence, the plant exhibits antioxidant, antimicrobial, anti-inflammatory, and cytotoxic activities, which facilitate its use for medical and pharmaceutical purposes. Research has also been conducted on the use of L. thuringiaca genes in enhancing the frost resistance of crop plants.
The advantages of L. thuringiaca related to its potential use in various production directions and its resistance to unfavorable habitat conditions prompt wider interest in this species, especially in view of the observed climate change and increasing water problems in the world. Diversification of cultivated plant species will enhance food security and economic sustainability. However, the literature on this species is scarce and requires further research, especially in terms of the chemical composition and low-cost methods of seed extraction and scarification. A very important aspect of further research should also be the assessment of the threat of uncontrolled spread of a new alien species in the agroecological space of Poland.