. Introduction
Cabbage (Brassica oleracea var. capitata) is a popular winter/cole vegetable belonging to the Cruciferae family. Cabbage is beneficial for health and nutrition; it is a rich source of vitamins B6, B9, C, and K. Cabbage also contains high amounts of calcium, potassium, magnesium, and small amounts of other micronutrients, including vitamin A, iron, and riboflavin. It is high in fiber and contains powerful antioxidants, including polyphenols and sulfur compounds. Half a cup of cooked cabbage has approximately one-third of the daily vitamin C requirement. In addition to its usual purpose as an edible vegetable, cabbage has historically been used for herbalism. In Bangladesh, this vegetable is grown in about 18,985.8 ha. The total cabbage production is approximately 320,332 metric tons (Bangladesh Bureau of Statistics, 2020). Because of its popularity and high consumer demand, this vegetable is grown worldwide. However, the cultivation of cabbage is highly susceptible to many phytopathogens, resulting in major yield loss and deterioration of leaf quality. Black rot is one of the most destructive seed-borne bacterial diseases of cruciferous plants, including cabbage, and is caused by the necrotrophic plant bacteria Xanthomonas campestris pv. campestris (Vicente & Holub, 2013). The black rot pathogen attacks all crucifers except the cole crops. The polymorphic species Brassica oleracea (including cabbage, cauliflower, broccoli, Brussels sprouts, and kale) are the most economically important hosts (Jensen et al., 2005). This cosmopolitan pathogen is responsible for about 50% of economic losses in cabbage yield and quality (Singh & Kumar, 2013; Vicente et al., 2001). In general, species of the genus Xanthomonas cause serious damage to the leaf tissue of the host plant, resulting in their wilting and necrosis (Velasco et al., 2013). Xanthomonas campestris pv. campestris pathogen invades xylem tissues through hydathodes and wounded tissues and prefers warm and humid conditions to infect the host plants. The characteristic symptoms of the disease are V-shaped chlorotic to necrotic lesions at the borders of the leaves and blackening of the vascular tissues (Alvarez, 2000). Diseased leaves and heads have poor market value and are unsuitable for storage because they quickly rot after harvest (Massomo et al., 2004). Disease progression and aggressiveness ideally depend on the pathogenicity and virulence of the diverse Xanthomonas campestris pv. campestris races. Among these, Races 1 and 4 have been identified as the most virulent species in Brassica oleracea (Jensen et al., 2010). Disease management is usually attempted using pathogen-free seeds in cultivation, eliminating infected plant debris and cruciferous weeds, crop rotation, and using agrochemicals. The control of black rot pathogens in economically important crops remains challenging owing to the emergence of new Xanthomonas campestris pv. campestris races in different agro-climatic regions. Some conventional methods, including the use of different plant extracts, help control black rot (Vicente & Holub, 2013). This disease can be controlled using culture, chemical, biological, and host resistance methods. Various control measures have been adopted both at the farm level and research centers, but no method alone can effectively control the disease (Celetti & Kristen, 2002). Various yeast strains, Paenibacillus isolate (Ghazalibiglar, 2014), Bacillus strains, and plant growth-promoting rhizobacteria (PGPR), such as Bacillus subtilis R14, B. pumilus C116, and B. megaterium pv. cerealis RAB7, and B. cereus C210, are important biocontrol agents exhibiting antimicrobial effects against Xanthomonas campestris pv. campestris bacterium (Ghazalibiglar et al., 2016; Luna et al., 2002; Massomo et al., 2004). However, agrochemicals (pesticides, herbicides, and insecticides) that farmers use create an unfavorable environment for the survival of biocontrol agents. Although antibiotics in the streptomycin group are routinely recommended for the management of this disease, their use over large acreages is not economically feasible. Extracts of several plant species, such as Datura metel (Datura), Allium sativum (Garlic), and Zingiber officinale (Ginger), and weeds, such as Parthenium hysterophorus (Congress grass) and Spiranthus indicus (Gorakh mundi), have been reported to be effective against clinical and plant pathogens (Parveen et al., 2014; Vicente & Holub, 2013). The future of biocontrol agents against plant diseases is bright and promising. With the growing demand for biocontrol products, biological control can emerge as an effective strategy to manage plant diseases, increase yield, protect the environment and biological resources, and approach a sustainable agricultural system. Plants possess various secondary metabolites that inhibit the growth of pathogens (Nascimento et al., 2000). Efforts are underway to search for economically safe phytochemicals that can be utilized for disease control.
Rapid identification and application of different botanical extracts before the onset of black rot disease symptoms in cruciferous crops are necessary. Therefore, accurately identifying different plant extracts and their most efficacious concentrations is a prerequisite for effective control measures. This study aimed to identify appropriate botanical plant extracts that could control black rot pathogen Xanthomonas campestris pv. campestris. To test the biocontrol alternatives of chemicals, extracts of 10 medicinal plants: royal poinciana (Delonix regia), ivy gourd (Coccinia grandis), sisso (Dalbergia sissoo), bougainvillea (Bougainvillea spectabilis), mint (Mentha spicata), devil’s cotton (Abroma augusta), drumstick (Moringa oleifera), false daisy (Eclipta alba), asthma plant (Euphorbia hirta), and mimosa (Mimosa pudica) were tested for their antibacterial effects against Xanthomonas campestris pv. campestris. So far, little information is available regarding the efficacy of selected plant extracts in controlling Xanthomonas campestris pv. campestris pathogen to minimize production losses.
. Material and Methods
Bacterial Strain
The bacteria Xanthomonas campestris pv. campestris (HRI-W-1279A) were used in this study. The strain was obtained from the culture collection at the School of Life Sciences, Wellesbourne Campus, University of Warwick, UK. The culture was maintained at 28 ± 2 °C on King’s medium B base with periodic subculturing.
Medicinal Plants
The 10 medicinal plants: royal poinciana, ivy gourd, sisso, bougainvillea, mint, devil’s cotton, drumstick, false daisy, asthma plant, and mimosa were collected from Noakhali Science and Technology University, Bangladesh (Table 1). Disease-free, clean, fresh, and healthy leaves were collected from these plants.
Table 1
Description of the medicinal plant materials.
Processing the Collected Leaves
We collected 1 kg of fresh, healthy, and disease-free raw leaves from each medicinal plant. The leaves were then surface-sterilized with sodium hypochlorite solution and washed thoroughly using distilled water. The clean leaves were air-dried at room temperature in the laboratory. After drying, the leaves were ground with a mortar and pastels to make leaf powder.
Preparation of Plant Extracts
To prepare aqueous plant extracts, 10 g of leaf powder was placed in a beaker, and 100 mL of distilled water was added. The leaf powder was mixed thoroughly to prepare the solution and left for 6 hr to soak properly at room temperature. The solution of each leaf powder was transferred to two 50 mL Falcon tubes and centrifuged at 14,000 rpm for 10 min using a centrifuge machine (Esco Micro; Changi, Singapore). The supernatant was collected using a micropipette. The supernatant was filtered using filter paper (Whatman International; Maidstone, England). The filtrates were considered stock solutions and preserved at 4 °C. From this stock, 25% and 50% water solutions were prepared before application. To obtain the ethanol extract, 10 g of leaf powder from each sample was homogenized in 100 mL of ethanol (solvent). The solution was kept at room temperature (25 ± 2 °C) for 4 hr. Then, the solution of each leaf powder was transferred to two 50 mL falcon tubes and centrifuged at 14,000 rpm for 10 min, after which the supernatant was allowed to evaporate completely. The residue was dissolved in sterile distilled water to obtain the extract (1 g/mL). The extract was stored as a stock solution at 4 °C for further use. From this stock, 25% and 50% ethanol extracts were prepared before application.
Antibacterial Assay
The antibacterial activity of the aqueous and ethanol extracts of 10 medicinal plants was tested using the disc diffusion method following Bauer’s procedure (Bauer et al., 1966). Muller Hinton agar medium was seeded with 100 µL of bacterial inoculum (1 × 108 CFU/mL). The impregnated disks (6 mm) containing the test sample (100 µg/mL) were placed on an agar medium seeded with the test bacteria. Standard antibiotic disk streptomycin (10 µg/disk, active ingredient: streptomycin sulfate) (Flinn Scientific; Batavia, Illinois, USA) and blank disks (impregnated with distilled water) were used as positive and negative controls, respectively. The plates were then incubated at 37 °C for 48 hr to allow maximum growth of the test bacteria. The antibacterial activity of the 10 medicinal plant leaf extracts was determined by measuring the diameter of the zone of inhibition (ZOI) using a scale ruler (cm/mm) and was expressed in millimeters (mm). ZOI is an area with no bacterial growth around the disk. The zone size indicates the antibacterial activity of the given treatment because the bacterium cannot grow in this area due to the presence of an antibacterial substance. Therefore, a larger inhibition zone indicated a good antibacterial effect of the given treatment. The assay was repeated twice, and the mean of three experiments was recorded.
Statistical Analysis
All data are expressed as mean ± standard error (SE). Statistical analyses for medicinal plant extracts were evaluated using one-way ANOVA followed by Tukey’s HSD test using STATISTICS 10 (version 10.0.0.9) software. Differences were considered statistically significant at p < 0.05. The relationship between different aqueous and ethanol extract levels was analyzed, and graphs were generated using the ggplot2 package in R (https://www.r-project.org/). Medicinal plants were categorized into three types: trees, herbs, and shrubs, and distilled water and streptomycin were denoted as c and c1, respectively. Variance explained (VE) was calculated for every level of aqueous and ethanol extracts with medicinal plants and their types individually and with their mutual interaction through a linear model followed by ANOVA with R. Multivariate analysis of variance (MANOVA) was performed for plant types, and a boxplot was constructed with R.
. Results
Antibacterial Activity of Medicinal Plants
The antimicrobial effects of all 10 medicinal plant extracts, streptomycin (positive control), and distilled water (negative control) against X. campestris pv. campestris are shown in Figure 1–Figure 4. Antibacterial activity was measured as the radius of the zone of inhibition (ZOI, mm). The size of the zone indicates the antibacterial activity of each plant extract. The results obtained in the present study showed that the 10 medicinal plant extracts possess potential antibacterial activity against X. campestris pv. campestris. Among them, seven plants showed significant inhibitory effects (p < 0.05) at both 25% and 50% aqueous and ethanol extract concentrations when compared with streptomycin (Table 2, Table 3). Royal poinciana, ivy gourd, sisso, bougainvillea, mint, devil’s cotton, drumstick, and false daisy showed potential inhibition of X. campestris pv. campestris at all concentrations. Royal poinciana showed the best result with inhibition zones of 21.67 ± 0.17 mm and 22.67 ± 0.33 mm with 25% and 50% aqueous extracts, respectively, and 23.50 ± 0.29 mm and 24.67 ± 0.17 mm with 25% and 50% ethanol extracts, respectively, followed by ivy gourd, sisso, bougainvillea, mint, and devil’s cotton. Mimosa and asthmatic plants gave small inhibition zones. The smallest inhibition zone was found in mimosa (6.17 ± 0.17 mm) and asthma plants (6.33 ± 0.17 mm) with 25% aqueous extracts. For all 10 medicinal plants, inhibition zones were larger with ethanol extracts than with aqueous extracts, and inhibition zones increased with increasing concentration. The streptomycin inhibition zone was 16.33 ± 0.17 mm, larger than the inhibition zones of false daisy, mimosa, and asthma plant at both concentrations of aqueous and ethanol extracts and 25% aqueous extracts of devil’s cotton and drumstick. As expected, no inhibition zones were observed in distilled water (Table 2, Table 3).
Figure 1
Antibacterial effect of 25% aqueous extracts of medicinal plants.
The letters represent the significant effects of plants on each other. The whiskers above the bars represent standard error (SE).
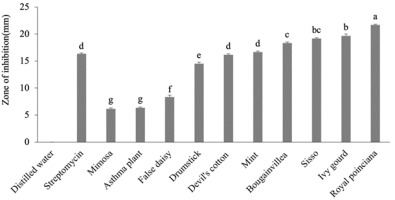
Figure 2
Antibacterial effect of 50% aqueous extracts of medicinal plants.
The letters represent the significant effects of plants on each other. The whiskers above the bars represent standard error (SE).
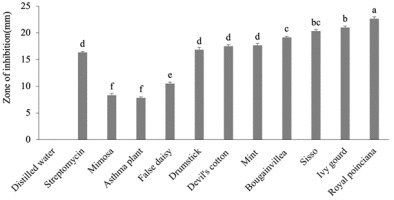
Figure 3
Antibacterial effect of 25% ethanol extracts of medicinal plants.
The letters represent the significant effects of plants on each other. The whiskers above the bars represent standard error (SE).
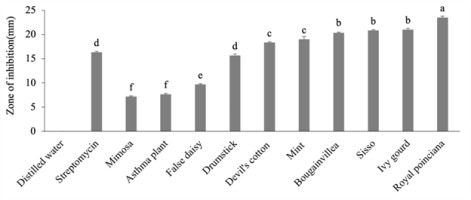
Figure 4
Antibacterial effect of 50% ethanol extracts of medicinal plants.
The letters represent the significant effects of plants on each other. The whiskers above the bars represent standard error (SE).
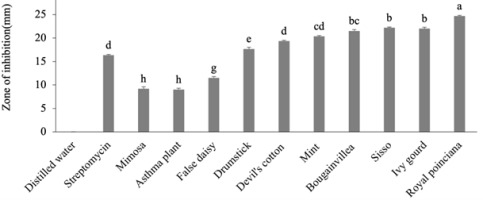
Table 2
Antibacterial activity of aqueous extracts of 10 medicinal plants (zone of inhibition in mm).
Table 3
Antibacterial activity of ethanol extracts of 10 medicinal plants (zone of inhibition in mm).
Relation Between Aqueous and Ethanol Extracts
The relationships between aqueous and ethanol extracts were highly linear, indicating that they mimic each other in the pathogen ZOI of cabbage, and 25% and 50% aqueous extracts were highly correlated with 25% and 50% ethanol extracts, respectively. In Figure 5, the data points are very close to the linear line, indicating a similar mechanism of action of the aqueous and ethanol extracts of the plants. This finding points to the importance of the aqueous extracts of selected medicinal plants capable of combating the black rot pathogen of cabbage.
Plant Types and Different Levels of Aqueous Extracts and Ethanol Extracts
Medicinal plants were categorized as trees, herbs, and shrubs. Distilled water (negative control) and streptomycin (positive control) were denoted as c and c1, respectively. In Figure 6, the x-axis is labeled with plant types and the y-axis with the ZOI of aqueous and ethanol extracts. The interquartile range (IQR) of herbs was higher but the median was lower than that of the other plant types. The highest median ZOI was found for trees. The median of shrubs was higher than that of herbs but lower than that of trees.
. Discussion
According to Bauer et al. (1966), bacteria are resistant to streptomycin at 10 µg/disk potency if ZOI is ≤11 mm, moderately sensitive if ZOI is 12–14 mm, and sensitive if ZOI is ≥15 mm. Therefore, compared with streptomycin, 25% and 50% of both aqueous and ethanol extracts of royal poinciana, ivy gourd, sisso, bougainvillea, mint, devil’s cotton, and drumstick showed significant antibacterial activity against X. campestris pv. campestris (Xcc), indicating that the pathogen is sensitive to the extracts mentioned above. Xcc was resistant to the false daisy, mimosa, and asthma plant extracts. The antibacterial activities of seven plant extracts were greater than those of streptomycin. In addition, the results obtained in this study indicated that the increase in the ZOI was invariably proportional to the increase in the concentration of both aqueous and ethanol extracts of the medicinal plants. The bark extracts of Delonix regia and Erythrina humeana exhibited potent antioxidant activities and moderate antibacterial activity against Dickeya dianthicola, Pectobacterium carotovorum, and D. chrysanthemi that cause wilting in potato and dianthus crops (Modi et al., 2016). The aqueous bark extract of M. oleifera had significant antibacterial activities against four X. campestris pathovars (Goss, 2018). Different extracts of M. oleifera have shown variable antibacterial activity against Escherichia coli, Staphylococcus aureus, Salmonella gallinarum, and Pseudomonas aeruginosa (Goss, 2018). The methanol extracts of E. alba demonstrated antibacterial activity against X. oryzae, X. campestris, and Erwinia carotovora. Extracts of plants, such as turmeric, garlic, guava, bael, eucalyptus, neem, gudhal, tulsi, lantana, and castor, were significantly effective against Xanthomonas axonopodis pv. citri (Negi & Kumar, 2015). Euphorbia hirta inhibits the growth of E. coli, Proteus mirabilis, Pseudomonas aeruginosa, and S. aureus (Singh & Kumar, 2013).
Xanthomonas campestris causes severe losses in agricultural yield worldwide by causing cruciferous black rot disease. Black rot is an intensively destructive disease in all crucifers, including cabbage, cauliflower, turnip, kale, and broccoli. Some synthetic antibiotics like bacterimycin, streptocycline, streptomycin, and tetracyline (the active ingredients are di-bromo, di-nitro, propane-1,3-diol; streptomycin sulfate 90% + oxytetracycline 10%; streptomycin sulfate; and tetracycline hydrochloride, respectively) are used for the management of bacterial diseases. However, frequent use of pesticides may promote the development of new strains of pests resistant to these chemicals. Pesticide accumulation in the soil may kill decomposers. In summary, pesticide use is harmful to ecosystems and the environment. Therefore, the development of eco-friendly methods for the management of plant diseases to protect the environment is of great concern.
. Conclusion
In the present study, medicinal plant extracts exhibited antibacterial properties. Among the medicinal plants tested, royal poinciana, ivy gourd, sisso, bougainvillea, mint, devil’s cotton, and drumstick showed strong antibacterial activities against Xanthomonas campestris pv. campestris (Xcc), whereas the activities of false daisy, mimosa, and asthma plants were comparatively weak. The present study indicates that medicinal plants have antimicrobial activity and can be used to manage black rot disease caused by Xcc. In vitro evaluation of the antimicrobial activities of plants is the first step toward developing eco-friendly management strategies for infectious diseases. However, these findings must be corroborated under field conditions in host plants, such as cabbage or cauliflower. Further studies are required to better understand the antibacterial properties of the selected medicinal plants.