. Introduction
Alpine and subalpine ecosystems worldwide sensitively documented global environmental changes that threaten their biota; however, the effects can have a more serious impact on habitats of the mid-latitude mountains with limited alpine area. Mountain regions show steep gradients in abiotic conditions and contain highly specialized vegetation in distinct habitats (Körner et al., 2017; Nagy & Grabherr, 2009), which makes them sensitive indicators of environmental modifications. The impacts of climatic changes are commonly mentioned, but mountainous areas are also subject to anthropogenic impacts, including changes in land use, atmospheric pollution, and recreation pressure.
Changes in the community composition of vascular plants were observed and predicted as an impact of climate change, particularly as a consequence of mean temperature increase and precipitation regime alteration, in mountainous regions (Pauli et al., 2012). Shifts in the altitudinal range margins of plant species, bioclimatic zones, functional plant groups, local extinctions, and declines in diversity together with invasions are mentioned as the main consequences of climate change in the mountain regions (Cannone et al., 2007; Rumpf et al., 2018; Theurillat & Guisan, 2001).
The processes in the area of the forest line ecotone are essential for currently observed changes in the subalpine vegetation (Descombes et al., 2017; Gavazov et al., 2014; Treml et al., 2016). Changes in land use, eutrophication, and climate are considered meaningful drivers of vegetation shifts in the limited narrow alpine area that form a refugia for cold-adapted and endemic species in central Europe (Jeník, 1997, 1998; Messerli & Ives, 1997). Glacial and interglacial stages of the Quaternary and the development of alpine habitats during the Holocene were important implications for plant diversity formation in central Europe (Comes & Kadereit, 2003).
In the High Sudetes Mts., significant human impact was detected during the High Middle Ages (Novák et al., 2010). Since the 17th century, the alpine area of the High Sudetes Mts. has been regularly managed (Hošek, 1964; Jeník, 1998; Klimeš & Klimešová, 1991). Grazing of domestic animals and haymaking were the first human activities in alpine habitats that lasted until World War II. The region of central Europe was heavily impacted by industrial pollution in the 1970s (Fabiszewski & Brej, 2000). Eutrophication and acidification appeared as consequences of coal combustion with catastrophic effects on the chemism of some natural habitats in the Sudetes Mts. (Hájková et al., 2011). Nutrient increase enabled the spread and invasion of nitrophilous species and caused the retreat of poorly competitive species, commonly forbs. Climatic change is connected with further changes in subalpine communities (Zeidler et al., 2014) and habitats close to the forest line ecotone (Treml et al., 2016) in the High Sudetes Mts. The limited-extent islands of forest-free areas are shrinking due to rising forest line that evoke more severe consequences for mid-latitude mountains in terms of global changes than for large high-mountain systems (Krajick, 2004; Treml et al., 2012). However, only a limited number of studies are located in mountains of smaller range with restricted subalpine area.
Following Jurasinski and Kreyling (2007), we consider highly specialized and sensitive subalpine plant species appropriate for long-term observational studies in limited forest-free areas that can reveal directions of historical processes and their future development. We compare long-term vegetation changes in quasi-permanent plots in the same region, as was performed in other mountainous areas (Fischer & Stöcklin, 1997; Ross et al., 2010; Rumpf et al., 2018).
We hypothesized that the vascular plant species composition and functional groups changed during past decades because of environmental influences in the Eastern High Sudetes Mts. In particular, we aimed to address the following questions: (1) Does species abundance differ between two historical periods (1950s and 1970s) and 2019? (2) Does the functional composition of species differ among the studied periods?
. Material and methods
Study site
The Králický Sněžník Mts. (Śnieżnik Kłodzki, Glatzer Schneeberg, Spieglitzer Schneeberg) is one of three ridges in the High Sudetes Mts. that rise above the forest line. On the border between Poland and the Czech Republic, it reaches a maximum height of 1,424 m a.s.l. (50.207 N, 16.847 E), the mean forest line is at 1305 m a.s.l., and the forest-free area is approximately 65 ha (Treml & Banaš, 2000). It is formed by one of the oldest European pre-Cambrian rocks, and the area belongs to the Lugikum boundary zone of the western Sudetes Mts.; hence, metamorphic rocks dominate there (Jahn et al., 1996). Most of the area was affected by periglacial conditions during the last Pleistocene glaciation; thus, glacial and periglacial landforms persist to the present day (Křížek et al., 2019). The average temperature of the topmost parts is 1.7 °C (Czech Hydrometeorological Institute), and the average annual rainfall is 1,312 mm (Jahn et al., 1996).
In the study area, the first studies of the subalpine flora came in the 19th century, and the first phytosociological records (Chytrý & Rafajová, 2003) were obtained in the 1950s (Vicherek, unpublished material), followed by an inventory in 1974 (Krahulec, 1990). The plant communities possess a similar pattern to those described in other parts of the High Sudetes Mts. (the Krkonoše Mts. and Hrubý Jeseník Mts.). The forest-free subalpine area is dominated by graminoids (e.g., Festuca supina, Nardus stricta, Calamagrostis villosa, Calamagrostis arundinacea, and Avenella flexuosa) and Vaccinium shrubs (V. myrtillus and V. vitis-idaea). Non-indigenous dwarf pine (Pinus mugo) was planted there during the nineteenth and twentieth centuries (Hošek, 1964; Novák et al., 2010).
Data collection
In 2019, we carried out a resurvey of plots in the following habitats: alpine heathlands Loiseleurio-Vaccinietea (AAA), alpine grasslands on base-poor soils of alliance Juncion trifidi (ABA) and of alliance Nardo strictae-Caricion bigelowii (ABB), the subalpine tall-forb vegetation of the alliance Calamagrostion villosae (ADA ass. Crepido conyzifoliae-Calamagrostietum villosae (ADA02), tall-fern vegetation of alliance Dryopterido-Athyrion (ADE), and alpine heathlands with Calluna vulgaris (TEF). Reference historical data, the original surveys from the 1950s and 1970s originate from The Czech National Phytosociological Database (Chytrý & Rafajová, 2003), and the vegetation units and abbreviations follow Chytrý (2010).
Plots were re-located using the original description of the plot in terms of slope, aspect, and topography. We used the original 15 vegetation relevés collected by J. Vicherek in 1958 (henceforth the 1950s) and 23 vegetation relevés from 1974 and 1973 collected by F. Krahulec in 1973 (henceforth the 1970s). The relevés were restricted to Czech territory. The locations of the relevés of both periods do not overlap, and relevés differ in their area; hence, they are considered as two separate datasets and were analyzed separately. The dimensions of relevés from the 1950s were 5 × 5 m (25 m2), and those from the 1970s were 4 × 4 m (16 m2). As the exact location of the original plots is unknown, three replicate plots were surveyed at the approximate location of each original relevé in 2019 to account for vegetation heterogeneity following Kapfer et al. (2017). The approach resulted in 45 new relevés in the area originally resurveyed in the 1950s, and 69 new relevés in the area resurveyed in the 1970s (114 in total).
Species cover was estimated using Braun-Blanquet’s seven-grade abundance-dominance scale (van der Maarel, 1979) and the nomenclature of plant species followed Danihelka et al. (2012). Species cover expressed on Braun-Blanquet’s scale was converted to percentages by Tüxen transformation and square root transformation to reduce differences among values (van der Maarel, 1979). We used Turboveg (Hennekens & Schaminée, 2001) and Juice (Tichý, 2002) software for processing and editing data.
To discriminate plant functional groups, species strategies, and growth forms were compiled from the database Pladias (Chytrý et al., 2021). The degree of specialization of a species based on its realized niche along multiple environmental gradients – the ecological specialization index (ESI) – followed Zelený and Chytrý (2019). A species list with attributes is shown in Appendix S1.
Data analysis
The dissimilarity matrix of Bray–Curtis distances was calculated after the square-root transformation of species data. Following the methods of Ross et al. (2010), we used ‘vegdist’ from the R (ver. 3.6.3; R Core Team 2020) package Vegan (Oksanen et al., 2019) to perform pairwise comparisons of the original and replicate plots and between all iterative comparisons of the replicate plots.
To identify species that are responsible for compositional shifts in vegetation, the permutation p value of the association between a species vector and a vector of memberships to a site group was computed. We used the function signassoc (package indicspecies; De Cáceres & Legendre, 2009) with correction for multiple testing using the Sidak method. Time shifts in vegetation composition were assessed using permutational multivariate analysis of variance (PERMANOVA), which was preferred as a robust method for heterogeneity (Anderson & Walsh, 2013). The matrix of Bray-Curtis distances (vegdist function, package vegan) was used in PERMANOVA. The restricted Monte Carlo permutation test with 999 permutations was used in each analysis.
To evaluate a dissimilarity matrix, the analysis of similarity (ANOSIM) was applied (package ‘vegan’) as it used ranked dissimilarities and it is a complement to an NMDS plot (Clarke, 1993). Species richness was expressed as the Shannon–Wiener index, and Pielou’s evenness was determined. To analyze differences in species richness between years, a two-sample permutation test (package coin) was used. To assess species heterogeneity and whether species heterogeneity changed among the periods of time, we calculated β-diversity by multivariate homogeneity of group dispersion variances using PERMDISP2 (function betadisper of package Vegan 2.4.2; Anderson et al., 2006) and Bray-Curtis distances. To visualize changes in vegetation composition between years, we applied the nonmetric multidimensional scaling (NMDS) ordination method to the Bray-Curtis dissimilarity matrix (Anderson, 2001). The abundances of each species were square-root transformed, and the analysis was adjusted for localities as covariable data. The significance of the canonical axis was tested by a Monte Carlo permutation test (999 permutations).
The cover of a particular functional group was computed by the function functcomp (package FD). The differences in the mean cover of functional groups were tested using a two-sample t-test, and the function oneway_test (package coin) was used. The functional groups with significant changes were visualized by NMDS, and Bray-Curtis distance was used.
. Results
Based on the PERMANOVA (F = 9.48, p< 0.01) and ANOSIM (R = 0.48, p < 0.01) results, the compositional heterogeneity of relevés of the 1950s differs significantly from that of 2019. Significant differences were also confirmed (PERMANOVA F = 4.85, p < 0.001; ANOSIM R = 0.28, p < 0.01) between the relevés of the 1970s and 2019.
Diversity
A total number of 69 vascular plant taxa were identified across the three observed periods. The two-sample permutation test revealed that the species richness in plots in 2019 was lower than in the 1950s (z value = 2.993, p < 0.01). The result also corresponded with the Shannon–Wiener index (1.63; 1.18) and evenness (0.50; 0.35) in the 1950s and 2019, respectively. A significant difference was not observed among plots in the 1970s and 2019. The results of PERMDISP2 showed significant overall differences in β-diversity between the phytosociological dataset from the 1950s and the dataset from 2019 (F = 9.43, p = 0.006). Based on a comparison between the phytosociological dataset from the 1950s and the set of repeated relevés from 2019, β-diversity in the 1950s was higher than that in 2019 (F = 6.43, p = 0.016) – Appendix S2. The PERMDISP2 β-diversity of the phytosociological dataset from the 1970s was higher than that of the dataset from 2019 (F = 7.85, p = 0.003).
Composition
The results of NMDS showed the overall difference in compositional heterogeneity between the periods 1950s and 2019 (Figure 1). The plant species composition showed a significant change towards homogenization, and species richness was lower in 2019 than in the 1950s (r2 = 0.57, p < 0.01). Several dominant plants (e.g., Vaccinium myrtillus, Picea abies, Luzula luzuloides ssp. rubella, and Calamagrostis villosa) with positive temporal responses to 2019 were recorded.
Figure 1
Diagram of nonmetric multidimensional scaling (NMDS) of a Bray-Curtis distance matrix describing the distribution of individual samples based on the variability of the species composition of vascular plants in the 1950s and 2019. The most significant species are represented by red arrows. The color of points and ellipses indicates the particular period of time; ellipses show the overall shift in species composition and represent 95% confidence areas based on the standard errors of the average of the axis scores. Dashed ellipses show changes in compositional heterogeneity and represent where 95% of the data are located.
Vegetation types: alpine heathlands Loiseleurio-Vaccinietea (AAA), alpine grasslands on base-poor soils of alliance Juncion trifidi (ABA), and alliance Nardo strictae-Caricion bigelowii (ABB), subalpine tall-forb vegetation of order Calamagrostis villosae (ADA), and heathlands with Calluna vulgaris (TEF).
The stress value is a measure of the disagreement between the rank order in the original dataset and that in the NMDS (lower numbers indicate better agreement).
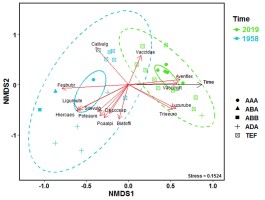
The results of NMDS did not show a significant difference in compositional heterogeneity in the phytosociological dataset between the 1970s and 2019, but the different diameters of 95% confidence ellipses indicated homogenization (Figure 2).
Figure 2
Diagram of nonmetric multidimensional scaling (NMDS) of a Bray-Curtis distance matrix describing the distribution of individual samples based on the variability of the species composition of vascular plants in the 1970s (yellow color) and 2019 (green color). Points and ellipse colors indicate the period of time; dashed ellipses show changes in compositional heterogeneity and represent where 95% of the data are located. Solid ellipses show the overall shift in species composition and represent the 95% confidence ellipses based on the standard errors of the average of the axis scores.
Vegetation types: alpine grasslands on base-poor soils of alliance Juncion trifidi (ABA), subalpine tall-forb vegetation of order Calamagrostis villosae (ADA), alliance Crepido conyzifoliae-Calamagrostietum villosae (ADA02), tall-fern vegetation of alliance Dryopterido-Athyrion (ADE), and heathlands with Calluna vulgaris (TEF).
Arrows represent vectors of 14 important species.
The stress value is a measure of the disagreement between the rank order in the original dataset and that in the NMDS (lower numbers indicate better agreement).
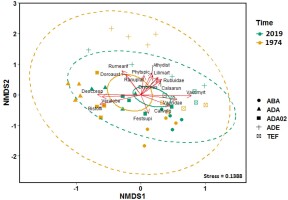
Functional groups
The distribution of functional groups differed significantly in most cases between the two phytosociological datasets from 1950s–2019 and 1970s–2019 (Table 1). Differences were proven in species strategies, growth forms, and ESI. Since the 1950s, a general trend of cover reduction of both forbs and Pteridophytes and an increase of graminoids and, to some extent, dwarf shrubs can be observed.
. Discussion
In the eastern part of the Sudetes Mts, we focused on vegetation changes in a mountain range with a narrow forest-free area, where management and climate changes can have far-reaching consequences for vegetation. We documented distinctions in subalpine vegetation of the study site among three periods over a period of 60 years that confirmed qualitative and quantitative changes. Decreases in alpha and beta diversity were detected, confirming the homogenization of the vegetation over time. There were also changes in the cover of particular plant species that belong to specific functional groups.
Diversity
The low overall species number formed in the subalpine belt on base-poor soils is a characteristic feature of the habitats in the Sudetes Mts. (Chytrý, 2010). The decline in diversity has been documented since the 1950s, even though species richness of vascular taxa is low across the three observed periods. The decline is congruent with observations across European mountain ridges (Britton et al., 2009; Czortek et al., 2018; Jurasinski & Kreyling, 2007; Ross et al., 2012), including the Eastern Sudetes Mts. (Zeidler et al., 2014). However, the study of Steinbauer et al. (2018) documented the opposite diversity trend in non-isolated mountain ranges in Europe. The meta-analysis repeated plant surveys from 302 mountain summits across Europe, spanning 145 years of observation. Global warming and climate-induced biotic change accelerate the documented increase in plant species richness. A decrease in beta diversity (homogenization) is often accompanied by an increase in the value of alpha diversity, as was documented in the Norwegian mountains (Odland et al., 2010) and the Alps (Jurasinski & Kreyling, 2007; Vittoz et al., 2009). The apparent increase in homogeneity is evoked by the reduction in the spatial turnover of species composition, a reduction in β-diversity (Baselga et al., 2018). Biotic or taxonomic homogenization, defined by Petsch (2016) as the increase in taxonomic similarity among communities, usually occurs when a widespread environmental change promotes the expansion of a few widespread general species. The variability in vegetation composition between plots was reduced over the last decades as a consequence. In contrast, the increase in alpha diversity is considered to be a consequence of an altitudinal shift in alpine vegetation under global warming. The increase in the number of species is attributed to immigration from the lowlands but also to the filling process by plant species that occurs in surrounding habitats (Pauli et al., 2007).
Table 1
Differences in the functional groups using a two-sample t-value test or Wilcoxon test as a non-parametric alternative for distinctions in functional groups of plants in the 1950s–2019 (a), and 1970s–2019 (b).
At our study site, we documented a decrease in both beta diversity and alpha diversity. Similar trends were described (Ross et al., 2012) for heath and grassland communities of the Scottish Highlands. The authors pointed to other drivers of the changes that interact with global change. In particular, shifts in traditional land use, soil chemistry, and pH related to atmospheric deposition of nitrogen and climatic changes have the potential to alter species composition, species richness and, hence, plant attributes in subalpine belts.
In a particular mountain region, the increase in alpha diversity depends on the immigration of other species from other mountain systems or from lowlands that also depend on specific barriers and restrictions (Jurasinski & Kreyling, 2007). Humans facilitated the onset of many species to alpine belts through land use, building roads, tourism infrastructure, and an increasing number of visitors. This is particularly true in the western part of the High Sudetes Mts. and in the Krkonoše Mts. (Vítková et al., 2012). In contrast, the limited alpine belt of the study area is characterized by less human impact, a greater distance from other mountain systems, and forest belts that prevent upward migration and species enrichment (Jeník, 1998). The trend of increasing homogenization is similar to that in other mountainous territories, while an increase in alpha diversity cannot be expected in the study area. Increasing homogenization was also observed in understorey vegetation of sub-montane forests in the Czech Republic (Prach & Kopecký, 2018), and the process was driven by extinctions of species specialist under the impact of environmental and land-use changes. Following Holzinger et al. (2008), we attributed the loss of species to competition pressure caused by the expansion of particular species as a consequence of environmental changes.
Species composition
Species that increased their presence (e.g., Avenella flexuosa, Vaccinium myrtillus, Picea abies, Calamagrostis villosa, Luzula luzuloides, and Ligusticum mutellina) are a common part of the subalpine communities and possess generalist traits. In contrast, the retreat of specialists (e.g., Potentilla aurea, Hieracium alpinum, Viola lutea subsp. sudetica, Homogyne alpina, and Hieracium caesium) took place at the same time in the subalpine belt. Major changes in community composition among periods were observed in a limited number of species, especially dominants. The maximum values of shifts are reached at the transition between the wooded andunwooded areas in many mountainous regions (Descombes et al., 2017; Gavazov et al., 2014; Kikvidze et al., 2005). Shifts and species decreases were also documented in Scottish alpine vegetation that was re-surveyed in 1963–1987 and 2004–2006 (Britton et al., 2009). There was an overall increase in cover of generalists such as Vaccinium myrtillus, which became more widespread. Nevertheless, they found random directions of the changes with no overall trends in Scottish alpine vegetation. In our case, the visible long-term pauperization of the area above the forest line at the Králický Sněžník Mts. is evidenced by historical comparisons. Following the floristic data from the nineteenth century summarized by Fiek (1881), several alpine species disappeared during the time: Coeloglossum viride, Conioselinum tataricum, Dianthus speciosus, Epilobium nutans, Hieracium prenanthoides, and Pseudorchis albida (Szeląg, 2002, personal field experience). In comparison with the situation in the 1970s, Hypochoeris uniflora and Campanula barbata decreased their numbers substantially (Krahulec, personal observation). Taking into account other European studies (Britton et al., 2009; Gavazov et al., 2014; Kikvidze et al., 2005) reduction in the total number of vascular plant species is anticipated in the subalpine area. We underline the process of colonization and spread that could lead to greater changes in the future, as the species’ responses depend on changes in their environment and functional traits, especially growth forms.
Growth forms
Changes in the specific responses of plant species were reflected in shifts of specialization and growth forms during the second half of the 20th century. Based on functional traits (Wild et al., 2019), we revealed the trend of an increase in generalists at the expense of specialists in both periods. Specialists are considered to be more sensitive to habitat conditions than generalists and hence more susceptible to population reduction or even extinction under environmental changes (Zelený & Chytrý, 2019). Following Chytrý et al. (2018), we interpreted the retreat of specialists as site conditions changes in habitat quality and the ability of habitats to support these groups of species.
The group of specialists also included the functional group of forbs (e.g., Senecio hercinicus, Solidago virgaurea, Bistorta officialis, and Veratrum album subsp. lobelianum) that is particularly associated with the 1950s. Forbs receded at the expense of graminoids, which is in congruence with the findings of studies performed in both the northern tundra (Ross et al., 2012; Walker et al., 2006) and alpine communities (Klanderud & Totland, 2005; Theodose & Bowman, 1997), including the Sudetes Mts. (Zeidler et al., 2014).
Graminoids can withstand harsh conditions better than forbs (Körner, 2003). The functional group consists mostly of long-lived individuals that often reproduce clonally and thus quickly colonize open niches. Hence, graminoid species can close gaps in alpine habitats through encroachment rather than recruitment from seeds. The success of graminoids is also pronounced at sites with low remaining living biomass and high necromass (De Boeck et al., 2018).
Alpine vegetation of the European Alps also experienced changes in the area of shrubs in the second half of the 20th century, as documented by Cannone et al. (2007). Shrubs showed rapid expansion rates of 5.6% per decade there. Expansion of bilberry as a dominant dwarf shrub is also commonly documented in alpine and Arctic environments that can influence species composition and ecosystem functions (Boscutti et al., 2018). Its cover may become the driver of plant diversity and turnover and even overrule the effect of elevation in alpine ecosystems. Such development is particularly anticipated under environmental changes connected with the cessation of management and climatic changes.
The changes in diversity, species composition, and growth forms were also associated with the shifts in the occurrence of observed vegetation types. The increase occurrence of alpine heathlands Loiseleurio-Vaccinietea (AAA) and heathlands with Calluna vulgaris (TEF) was documented since the 50s. On the contrary, subalpine tall-grassland vegetation of order Calamagrostis villosae (ADA) and tall-fern vegetation of alliance Dryopterido-Athyrion (ADE) belong to the vegetation types reducing their occurrence during the monitored 60-year period. These changes underline vegetation shifts observed in the Eastern Sudetes Mts. (Klinkovská et al., 2023).
Environmental changes
We attributed the documented changes in subalpine vegetation to the cessation of traditional management, eutrophication, and climate change.
Seasonal farming, pasturing, and haymaking gradually declined in high mountains (Dirnböck et al., 2003) and abruptly ceased after World War II in the Sudetes Mts. (Klimeš & Klimešová, 1991; Krahulec et al., 2001; Treml et al., 2016). The pasture affected particular species by removing biomass, disturbing aboveground organs, and/or via zoochory (Klimeš & Klimešová, 1991; Krahulec et al., 2001). Management cessation is recognized as a trigger of plant biomass and litter accumulation that lowers seedling establishment and species richness, as documented in mountain grasslands (Galvánek & Lepš, 2012). Especially, regular pasturing and haymaking, as the main driving factors that removed biomass and caused disturbances, suddenly ceased. With the abrupt shift in land use, we consider the first analyzed step of environmental and plant composition shifts in alpine areas in the 1950s of the 20th century.
Environmental changes, especially increases in the trophy, are considered the important drivers of generalist species, graminoids, and dwarf shrub spread (Britton et al., 2009). The subalpine habitats show high sensitivity to nitrogen, as a dose of 5–10 kg/ha/year evokes plant production and increases species composition changes (Bobbink et al., 2015). At the study site, Fabiszewski and Brej (2000) documented approximately 50 kg/ha/year in the 1970–1980s, mainly as a consequence of brown coal combustion. Shortages of magnesium, calcium, and potassium and the toxic impact of aluminum and sulphuric dioxide were recognized as accompanying factors that were followed by changes in alpha diversity, including disappearance and shrinkage of the range of oligotrophic species at the expense of graminoids and ubiquitous plant species (Fabiszewski & Brej, 2000). The dose of atmospheric deposition decreased since then, yet it was approximately 15 kg/ha/year in subalpine areas of the Eastern High Sudetes Mts. in the 2010s (Czech Hydrometeorological Institute). Atmospheric nitrogen deposition is the most important indirect anthropogenic impact in central Europe, and we considered it the important driver of species and functional group changes at the end of the 20th century.
Dramatic changes in alpine plant communities are expected in the context of global climate changes (Pauli et al., 2012; Steinbauer et al., 2018). The trend of increasing air temperatures has also been demonstrated in the central European mountains (Urban & Tomczyński, 2017), which is also applicable to the Eastern Sutetes Mts. (Czech Hydrometeorological Institute).
The migration of trees upward into formerly treeless areas and forest line gradual increases in tree cover were documented in the Eastern High Sudetes Mts. during the 20th Century (Treml et al., 2016). This is congruent with our findings, as Norway spruce (Picea abies) showed an increase in its current presence compared to that in the 1950s and 1970s. Although the directions and speed of vegetation shifts under climate change are ambiguous at the local level (Alexander et al., 2018; Elmendorf et al., 2012; Hülber et al., 2016), we considered climate the driver of the turnover rate in communities (Descombes et al., 2017) and important determinants for future development.
Following previous studies (Chytrý et al., 2019), we considered changes in land use together with the disturbance regime, atmospheric nitrogen input connected with the loss of calcium and magnesium, and climatic changes to be the main drivers of biocenotic evolution, succession, and species expansions in subalpine habitats in the High Sudetes Mts. The current threatening factor for sensitive subalpine plant habitats is tourism. As a result of building tourism infrastructure, the number of visitors is increasing in the study area (Banaš et al., 2020). At present, there are big construction activities on the Polish side of the summit, just on the state border.
. Conclusions
The documented changes since 1950 underline the trends in vegetation structure that occurred under the complex influence of several environmental shifts in the High Sudetes Mts.
The vegetation shifts consisted of decreased diversity and homogenization, which were reflected by changes in spectra in plant functional groups.
The vegetation shifts are reflected by the receding of grasslands and encroachment of shrubs that underline common trends in the subalpine belt of central Europe.
The development of vegetation was probably linked to the cessation of historical land use, atmospheric deposition, and climate changes. Without active management, the negative trend will continue under the complex effect of the factors, particularly under intensifying trends in climate change and tourism.
Following experiments performed in the High Sudetes Mts. (Krahulec et al., 2001; Mrazkova-Stybnarova et al., 2020), we recommend implementing a suitable combination of mowing and grazing to maintain diversity, and to prevent homogenization of vegetation.
Further long-term monitoring is required to discover the effect and advance the understanding of the complex interaction of factors on subalpine vegetation in the future.
. Supplementary material
Appendix S1. List of species occurring in the plots, their abbreviations, and plant traits.
Appendix S2. Supplementary figures regarding the comparison of species heterogeneity in the studied periods and 2019 and regarding the overall trend of changes in species composition and plant functional groups among the studied periods 1950s, 1970s, and 2019.