. How it all started?
The monitoring of pollen grains in the air was initiated in England by Charles Blackley, who linked the presence of pollen in the atmosphere to the severity of allergy reactions (Blackley, 1873). Over the following decades, aerobiological research spread rapidly in Europe, gaining importance, especially in allergology (Weryszko-Chmielewska, 2007). In Poland, the first indication that airborne pollen analysis may have practical implications in medicine was provided by Dr. Mieczysław Obtułowicz (Jagiellonian University, Kraków). His pioneering work (Obtułowicz, 1939) presented the first phenological calendars of allergenic plants from Kraków and provided information about pollen morphology and its transport in the atmosphere. In the years 1960–1979, short-term aerobiological studies were set up in several Polish cities, including Kraków, Rabka, Warszawa, Bydgoszcz, and Łódź (Szczepanek, 2006; Śpiewak, 2001). The first analysis of fungal spore content in the air was conducted in Rabka and Kraków in 1960 (Weiss, 1962). Although these analyses were carried out using gravimetric methods and usually did not cover a long period, they provided the first data on the temporal and spatial variation in the content of airborne allergens in Poland. Great contributions to the promotion of this research were made by two Polish allergists, i.e., Dr. Edward Zawisza and Dr. Krzysztof Buczyłko (Buczyłko & Wnuk, 1979; Zawisza, 1974). The use of Hirst-type (Hirst, 1952) volumetric devices for aerobiological monitoring in Poland was initiated in 1987 in Rabka. A year later, volumetric monitoring was started in Kraków and Łódź. In the same year, the Allergen Research Center led by Dr. Piotr Rapiejko was established in Łódź (currently in Warszawa) (Śpiewak, 2003).
Among the pioneers of aerobiological research in Poland, one should mention Prof. Jadwiga Dyakowska (Jagiellonian University, Kraków), who already in the 1930s conducted classic experiments on the pollen settling velocity (Dyakowska, 1937). Prof. Kazimierz Szczepanek (Jagiellonian University, Kraków) and Prof. Małgorzata Latałowa (University of Gdańsk) have contributed immensely to the development of Polish aerobiology by promoting this field of science in the Polish scientific community, publishing articles (Latałowa & Góra, 1996a, 1996b; Obtułowicz et al., 1991; Szczepanek, 1994a), organizing pollen workshops, and supervising and reviewing many doctoral dissertations in the field of aerobiology. Thanks to Prof. Kazimierz Szczepanek, who started continuous aerobiological monitoring in Kraków in 1982 (Szczepanek, 1994b), the city now owns the longest aerobiological database in Poland and one of the longest in Europe. It is also worth mentioning Dr. Alicja Stach (Adam Mickiewicz University in Poznań), who in 1992 initiated aerobiological monitoring in Poznań (Stach, 2000; Stach & Silny, 1996, 1999), firstly with the gravimetric method, and from 1996 with the standard volumetric method of the Hirst design (Hirst, 1952). In 2003, she organized the first European Course on Basic Aerobiology in Poznań, Poland, under the auspices of the International Association for Aerobiology (IAA). In addition, she was the main organizer of several other international aerobiological courses in Poland (Stępalska et al., 2011). The development of aerobiological research in Poznań, and Poland as a whole, benefitted greatly from the European Union’s Marie Curie Actions Development Scheme (i.e., AEROTOP project) in collaboration with the Universidad de Córdoba (Spain) and University of Worcester (UK) (Smith et al., 2007, 2008; Stach et al., 2007a, 2008).
In the following years, new pollen monitoring centers were established in many cities, i.e., Rzeszów (1997), Sosnowiec (1997), Lublin (2001), Szczecin (2003), Łódź (2003), Wrocław (2003) and Piotrków Trybunalski (2005) (Szczepanek, 2006). Some of these research units have been cooperating for many years with the Polish Aerobiological Network (PAN) (http://www.aero.cm-uj.krakow.pl) (Figure 1) and European Aeroallergen Network (EAN) (https://www.polleninfo.org). Both platforms present current forecasts for allergy sufferers and practical preventive tips for people with pollen allergies. Finally, it is worth emphasizing that in 2005, the Aerobiological Section was established as part of the Polish Botanical Society (the first Sections’ Chair was Prof. Elżbieta Weryszko-Chmielewska from the University of Life Sciences in Lublin). Currently, the Section brings together over 20 scientists from all over Poland (Grewling, 2022).
. Bioaerosols in the outdoor air
Pollen concentrations – Variation and impacts of climate
Thanks to many years of pollen monitoring and the huge amount of collected data, Polish aerobiologists obtained comprehensive information on the spatiotemporal variation in pollen seasons in Poland as well as the role of both climatic and meteorological factors in shaping the pattern of pollen seasons. In general, it has been shown that pollen seasons in Poland start earlier and last longer than just a few decades ago. Such trends have been observed, e.g., in Tilia (Weryszko-Chmielewska et al., 2019), Quercus (Grewling et al., 2014a), Rumex, Poaceae, and Urticaceae (Bogawski et al., 2014). The study conducted in Lublin revealed that Amaranthaceae pollen seasons began two weeks earlier than 19 years ago (Piotrowska-Weryszko et al., 2021). In addition, in most of the analyzed plants, a significant increase in the seasonal airborne pollen concentration was observed in Poland (Ziello et al., 2012). For instance, the seasonal cumulative load of pollen in Kraków increased on average by 9.0% per year (Ziska et al., 2019). However, in several herbaceous taxa, e.g., Artemisia (Bogawski et al., 2014) and Amaranthaceae (Piotrowska-Weryszko et al., 2021), reduced pollen production has been documented in Poland.
Most of these changes can be explained by recent climate changes. For instance, it has been shown that high air temperature before flowering causes an earlier start of pollen seasons for such plants as hazel (Corylus sp.) (Dąbrowska-Zapart & Niedźwiedź, 2020; Grewling et al., 2014b; Malkiewicz et al., 2016), alder (Alnus sp.) (Dąbrowska-Zapart et al., 2018), birch (Betula sp.) (Grewling et al., 2012a; Piotrowska & Kubik-Komar, 2012), maple (Acer sp.) (Weryszko-Chmielewska et al., 2016), ash (Fraxinus sp.) (Kubik-Komar et al., 2018), oak (Quercus sp.) (Grewling et al., 2014a; Kasprzyk, 2009), lime tree (Tilia sp.) (Weryszko-Chmielewska et al., 2019), Poaceae (Myszkowska, 2014; Stach et al., 2008), and Amaranthaceae (Piotrowska-Weryszko et al., 2021).
In contrast, intensive rainfall recorded before flowering positively affects pollen production in herbaceous species, which results in higher pollen levels during the season. Such effect has been observed, e.g., for Artemisia (Stach et al., 2007a). In turn, periods of drought delay and weaken the intensity of the Artemisia pollen season (Bogawski et al., 2014; Grewling et al., 2012b). Similar relationships between meteorological conditions and pollen season intensity have also been noted for grasses in Poland. It was revealed that hot and dry summers, as well as cold and rainy summers, are not the optimal condition for grass pollen production (Myszkowska, 2014).
The impact of meteorological conditions on daily pollen concentration is very complex as many factors may act differently on airborne pollen level. In general, it has been however shown that an increase in daily air temperature often correlates positively with higher concentrations of airborne pollen grains in most allergenic taxa (Borycka & Kasprzyk, 2014; Dąbrowska-Zapart et al., 2018; Dąbrowska-Zapart & Niedźwiedź, 2020; Grewling et al., 2019b; Kubik-Komar et al., 2018; Weryszko-Chmielewska et al., 2006). On the other hand, rainfall (recorded during the pollen season) often decreases pollen concentrations in the air, potentially due to the “washout” effect. As revealed by Polish researchers, the intensity of precipitation is, however, the dominant control on pollen concentrations. Kluska et al. (2020) showed that pollen concentrations only decreased clearly under rainfall intensities of at least 5 mm h−1.
Fungal spore concentrations – Variation and impacts of climate
Similarly to allergenic pollen grains, the presence of fungal spores in the air is a threat to public health (Horner et al., 1995). Moreover, fungal spores are also essential pathogens of crops, fruits, and vegetables, causing significant losses in the agricultural, vineyard, and gardening industries (Dean et al., 2012; Jędryczka, 2014; Martínez-Bracero et al., 2020). In Poland, the most important aeromycological research centers are the Institute of Plant Genetics, the Polish Academy of Science in Poznań, the University of Szczecin, and Jagiellonian University in Kraków. In addition, important indoor fungal research is conducted at the Central Institute for Labour Protection - National Research Institute (CIOP-PIB) in Warszawa. The concentration of fungal spores in the air results from the interaction between biological and environmental factors. In Poland, the length and intensity of the spore season of many fungal taxa, such as Alternaria, Cladosporium, Drechslera, Torula, Botrytis, Erysiphe, Epicoccum and Didymella, depends primarily on the daily air temperature and relative humidity (Grewling et al., 2019b; Grinn-Gofroń, 2014; Grinn-Gofroń & Bosiacka, 2015; Grinn-Gofroń et al., 2019; Grinn-Gofroń & Rapiejko, 2009; Martínez-Bracero et al., 2020; Stępalska et al., 2012; Stępalska & Wołek, 2009). The highest concentration of these spores is often recorded in summer (June–August) (Kasprzyk et al., 2015). In contrast, the highest concentrations of Aspergillus and Penicillium were noted in September, October, and November (Grinn-Gofroń, 2011), while spores of pathogenic fungi of grapevine, e.g., Plasmopara viticola and Erysiphe necator, dominate in the air during May and June (Martínez-Bracero et al., 2020). An increase in the concentration of Alternaria and Cladosporium spores was also observed before a thunderstorm when air temperature and ozone concentration increased (Grinn-Gofroń & Strzelczak, 2013). It was also revealed that warm and dry weather during the sporulation season accelerates and elongates Cladosporium spore seasons in Poland (Kasprzyk et al., 2016). Finally, based on long-term aeromycological studies in Poznań (1998–2014), it was shown that an increase in average air temperature and precipitation between July–November resulted in an acceleration of the appearance of spores of Leptosphaeria maculans–L. biglobosa species complex in the air. This, in turn, greatly influences the severity of stem canker of oilseed rape caused by these fungal species (Dawidziuk et al., 2011; Kaczmarek et al., 2016).
Bioaerosols modeling and forecasting
The results of aerobiological studies are important not only from a purely biological point of view but can find applications in spatio-temporal modeling of pollen and spore concentrations, which is intensively developed in Poland (Bilińska et al., 2017; Nowosad et al., 2015, 2016, 2018; Werner et al., 2018, 2021). Such models can predict future changes in the course of pollen and spore seasons, which is particularly important in allergic prophylaxis. In addition, from an agricultural point of view, it is also important to predict the severity of fungal spore seasons accurately. Polish scientists made a significant contribution to the development of such forecasting models using diverse modeling techniques (e.g., multivariate regression trees, classification models, growing degree methods, and artificial neural networks) (Grinn-Gofroń et al., 2019; Grinn-Gofroń & Strzelczak, 2009; Kasprzyk, 2009; Kasprzyk et al., 2011a). For instance, long-term and short-term forecast models for grass pollen have been constructed in Poznań (Stach et al., 2008). The forecast models predicted the start of the grass pollen season within two days and achieved 61% and 70% accuracy. Satisfactory results were also achieved in the case of forecasting the start day of the oak pollen season in Rzeszów, especially using the modeling technique based on the indicator taxa (Kasprzyk, 2009). Some of the constructed models predict not only daily but also hourly variation in bioaerosol concentrations, e.g., as was shown in Szczecin for Alternaria and Cladosporium spores (Grinn-Gofroń & Strzelczak, 2009). Worth to add that Poland runs the System for Forecasting Disease Epidemics SPEC - one of the most extensive systems based on aerobiological methods - aiming to forecast the risk of stem canker of oilseed rape. The system is based on the estimation of Leptosphaeria maculans and L. biglobosa ascospores concentration in the air. Airborne spores that form inoculum responsible for disease epidemics allow for predicting the risk of yield losses and their quality (Jędryczka, 2014).
Sources and pathways of bioaerosols dispersal
Airborne pollen grains can be transported in the atmosphere at various distances depending on factors such as the strength and direction of the wind, regional and local topography, vegetation type, air turbulence, convection, and sea-land breezes (Gassmann et al., 2002; Grewling et al., 2022; Pauling et al., 2020; Szczepanek, 2003). Polish aerobiologists contributed significantly to understanding the dispersion of pollen grains in the atmosphere, often using advanced modelling techniques, e.g., air mass back trajectory and particle dispersion modelling (Werner et al., 2021). Atmospheric dispersion of pollen grains over Poland has been studied for such taxa as birch (Betula sp.) (Bogawski et al., 2019a; Myszkowska et al., 2021; Skjøth et al., 2007), alder (Alnus sp.) (Skjøth et al., 2015), and Pinaceae (Szczepanek et al., 2017) (Figure 2). Bogawski et al. (2019a) revealed that birch pollen from north-eastern Europe could be transported to Poland after the flowering period of local birch populations, while Myszkowska et al. (2021) showed that, under favorable synoptic conditions, birch pollen could arrive from southern Europe concomitantly with Saharan dust. Analyses by Szczepanek et al. (2017) confirmed that atmospheric transport of pine pollen could be expected before the local pollen season in southern Poland.
Figure 2
Atmospheric long-distance transport of pollen grains in Poland. Data based on: Betula (birch) (Bogawski et al., 2019a, Skjøth et al., 2007, 2015), Ambrosia (ragweed) (Grewling et al., 2016, 2019b, Šikoparija et al., 2013, Kasprzyk et al., 2011b, Stach et al., 2007b), Alnus (alder) (Skjøth et al., 2015), and Pinus (pine) (Szczepanek et al., 2017).
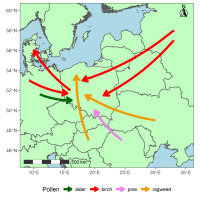
Significant findings concern the long-distance transport of highly allergenic pollen of ragweed (Ambrosia). It is an invasive plant that spreads throughout Europe and produces vast amounts of small and light pollen (Essl et al., 2015). Polish studies conducted in close cooperation with researchers from European centers confirmed that ragweed pollen: (i) reaches Poland most often from southern (Pannonian Plain) and eastern (Ukraine) Europe (Bilińska et al., 2017; Kasprzyk et al., 2011b; Šikoparija et al., 2013; Smith et al., 2008; Stach et al., 2007b; Stępalska et al., 2020), (ii) after traveling several hundred km, it is still immunoreactive (Grewling et al., 2016), and (iii) is often transported with other hazardous airborne particles, e.g., particulate matters from Silesia, fungal spores from Pannonian Plain and dust from Sahara desert (Grewling et al., 2019a). Cross-sectional studies on the spread of Ambrosia artemisiifolia pollen in Europe also include the experience of Polish scientists (Šikoparija et al., 2017).
The atmospheric transport of fungal spores was investigated less frequently by Polish aerobiologists than pollen transport. However, an occurrence of atypically high concentrations of spores has been linked to remote sources for such taxa as Cladosporium, Alternaria (Grewling et al., 2019a, 2022), Ganoderma (Sadyś et al., 2014), and Leptoshaeria (Grinn-Gofroń et al., 2016).
Relationship between phenology and bioaerosols concentration
The presence of bioaerosols in a certain location is not only the result of the flowering/sporulation of local populations but can also be modified by other phenomena, such as long-distance transport and re-suspension of bioaerosols. Therefore, there is often a mismatch between the timing of bioaerosols release and their concentration in the air. To better understand this phenomenon, joint phenological and aerobiological studies are required. Although aeromycological research is rare, there are plenty of Polish studies that examine the relationship between flowering and the course of pollen seasons, e.g., for tree species such as Alnus glutinosa, Alnus incana (Dąbrowska & Kaszewski, 2012; Kasprzyk, 2003; Pidek, 2007; Stępalska et al., 2016), Corylus avellana (Puc & Kasprzyk, 2013; Stępalska et al., 2016), Betula pendula (Kasprzyk, 2003), Populus wilsonii, Ulmus laevis, Salix caprea, Aesculus hippocastanum and Tilia cordata (Stach, 2006). In addition, observations of the flowering of several species of herbaceous plants in relation to airborne pollen were conducted in Szczecin (Kruczek & Puc, 2012).
The comparative analysis of flowering phenology and pollen seasons allows the identification of species that have the most significant impact on pollen abundance. For instance, in Lublin, it has been shown that among four species of Acer (maple tree), A. negundo and A. platanoides are responsible for the majority of Acer pollen in the air (Weryszko-Chmielewska et al., 2016). In contrast, among 10 lime tree species, Tilia cordata was the most important pollen contributor (Dąbrowska et al., 2016). The pollen production and flowering phenology of three Artemisia species have been compared to airborne pollen season in Poznań (Bogawski et al., 2016). The results of this study showed that A. vulgaris is the most important pollen source in the first part of the season, whereas A. campestris is the major pollen contributor during late summer (especially in the outskirts of the city). The linkage between airborne pollen data and the phenological observations of silver birch (Betula pendula) resulted in the construction of an accurate model for predicting the timing of flowering in a heterogeneous city area of Poznań (Bogawski et al., 2019b). Phenological observations of Alnus glutinosa, Corylus avellana, and Betula pendula conducted in Poland were also included in project No. NN305 321936, financed by the Ministry of Science and Higher Education, to develop forecasting models for predicting spatiotemporal variation in tree pollination (Nowosad et al., 2015, 2016, 2018).
. Bioaerosols in the indoor air
In contrast to pollen grains, the presence of fungal spores indoor may often be high enough to cause health problems. Indoor fungal spores are also extremely important in the context of the protection of cultural heritage (biodeterioration). For instance, the indoor monitoring in the Regional Museum in Rzeszów revealed high microorganism biodiversity (both fungal spores and bacteria) in the historical depots of the museum. The most common fungus was Penicillium expansum, while the most common bacteria were Micrococcus luteus/lylae and Staphylococcus warneri (Grabek-Lejko et al., 2017). Aerobiological studies were also carried out in salt mines such as Bochnia (Frączek et al., 2013) and Wieliczka (Myszkowska et al., 2019), emphasizing that microorganisms in the air of salt chambers seem to be related to the biological material carried in by patients and staff. The microbial quality of the working environment was assessed in office buildings with different ventilation systems, showing that effectively working and regularly maintained mechanical ventilation or air-conditioning systems ensure better hygienic quality in the office buildings than natural/gravitational ventilation (Gołofit-Szymczak & Górny, 2010, 2018).
. New approaches in bioaerosols analysis
Traditionally, monitoring of pollen and fungal spores in the air was carried out using both gravimetric and volumetric methods. The first of them was widely used in Poland at the turn of the 20th and 21st centuries (Kasprzyk, 1999; Puc, 2006; Puc & Puc, 2004), especially in remote areas where access to power supply was limited, e.g., in forests and mountains (Kaszewski et al., 2008; Pidek et al., 2006; Pidek, 2007; Stępalska et al., 2002). These studies provided valuable data on the modern deposition of pollen, which can be used in the reconstruction of the structure and composition of vegetation and climate in the past (Pidek et al., 2009). However, the gravimetric method does not allow measuring the number of particles in a given volume of air. Therefore it is now much less used than the volumetric method. Standard aerobiological monitoring is predominantly based on a 7-day volumetric trap of the Hirst design (Hirst, 1952) and light microscopic analysis of bioaerosols. Unfortunately, such a setup also has some limitations. For instance, due to the high morphological similarity, many pollen grains and fungal spores cannot be linked accurately to species or genus levels. Therefore, modern molecular research methods are increasingly used to analyze aerobiological samples. In Poland, the real-time PCR technique was successfully applied to identify two important fungal pathogens of oilseed rape (Brassica napus L.), i.e., L. maculans and L. biglobosa, which have identical spore shape and size (Grinn-Gofroń et al., 2016; Kaczmarek et al., 2009). The fungi were identified using dual-labeled fluorescent probes that were targeted to a β-tubulin gene fragment of either Leptosphaeria species (Jędryczka, 2014). Identification of pollen grains has been performed using different analytic methods, i.e., Fourier transforms infrared (FTIR) spectroscopy (Depciuch et al., 2016; Kasprzyk et al., 2018). These results indicated that the pollen grains of Betula pubescens and B. pendula have similar chemical compositions, but the pollen of B. humilis is outlaying. It has been emphasized that FTIR spectroscopy is sufficient for accurate, fast, and cost-effective identification of pollen. Recently, the imaging flow cytometry technique was also applied for the identification of pollen grains from Betulaceae, Oleaceae, Urticaceae, and Asteraceae families (Gierlicka et al., 2022).
In recent years, increasingly more attention has been paid to analyzing sub-pollen (and sub-spore) particles in ambient air (Buters et al., 2012, 2015; Galán et al., 2013; Górny, 2012; Górny et al., 2002; Grewling et al., 2019c). These tiny particles may also contain allergenic proteins but are not recognizable under a light microscope. Therefore, more advanced analytical and monitoring methods are required. Aerobiological monitoring coupled with immunoenzymatic analysis (ELISA) focused on detecting allergens in different air fractions has been run for almost two decades in Poznań within two European projects (MONALISA-ENV/F/000068 and HIALINE-20081107) and four national projects (NN404015439, 2011/03/D/NZ7/06224, 2013/09/D/NZ7/00358, and 2020/39/B/ST10/01554). The results of these projects have shown that the content of the main pollen grains allergens is subject to strong spatio-temporal variation. For instance, across Europe, the same amount of grass pollen can release variable amounts of allergen ranging from less than one to nine pg of Phl p 5 per pollen, and this difference varies per location in Europe and during the pollen season (Buters et al., 2015). The species-specific differences in pollen allergenicity were also observed in Poznań with respect to Artemisia pollen grains (Grewling et al., 2020a). It has been shown that the pollen of A. campestris contains 60% more Art v 1 (the major Artemisia pollen allergen) than A. vulgaris pollen. In Kraków, high differences in the content of the birch pollen allergens (Bet v 1, Bet v 2, Bet v 6, and Bet v 7) have been observed in pollen collected from differently polluted areas, and a significant increase in Bet v 1 amount was noticed in the sites with the highest pollution level (Ziemianin et al., 2021). The seasonal variation in the level of Alternaria spores allergen (Alt a 1) has also been documented (Grewling et al., 2019c). Alt a 1 has been found in different air fractions (PM > 10, PM2.5-10, and PM0.12-2.5), suggesting that apart from intact Alternaria spores, other airborne particles could also be a source of Alt a 1 (Grewling et al., 2020b).
Besides ELISA, the FTIR spectroscopy technique has been used to investigate changes in pollen proteins (Depciuch et al., 2017). For instance, it was found that in south-eastern Poland, in areas exhibiting low pollution, hazel pollen contains a lower amount of proteins. Significant differences in the spectra regions corresponding to proteins have been observed in mugwort pollen grains collected from sites with different pollution levels (Depciuch et al., 2016). Moreover, air pollution induced structural changes in macromolecules of Artemisia pollen by increasing the content of sporopollenin and decreasing the polysaccharide level.
In Poland, traditional volumetric monitoring of bioaerosols is increasingly often supplemented with state-of-the-art techniques, such as remote sensing and geospatial technology (e.g., satellite data) (Bogawski et al., 2019a, 2019b), sophisticated statistical modelling (Werner et al., 2018, 2021) or investment in automatic monitoring samplers (e.g., in University of Rzeszów). The latter could be a breakthrough in aerobiological research by providing real-time information on bioaerosols, which can be immediately used, e.g., in allergy prophylaxis or crop protection.
. What is the future of Polish aerobiology?
Polish aerobiologists are an important part of the European aerobiological family. They published more than 260 papers (based on the Scopus database), significantly increasing the knowledge about pollen grains and fungal spore production and dispersion in the atmosphere (Figure 3). During the past years, published papers have increased, especially in fields such as molecular aerobiology, aeromycology, and statistical modeling of aerobiological data. All analyzed articles (n = 260) were cited more than 6,300 times (based on the Scopus database), and approximately 30% of these papers were prepared in cooperation with European scientists. Among the closest collaborators are researchers from the UK (21.7%), Spain (9.6), and Serbia (6.0%) (Figure 4).
Figure 3
Research topics raised by Polish aerobiologists in scientific articles (n = 260, based on Scopus database).
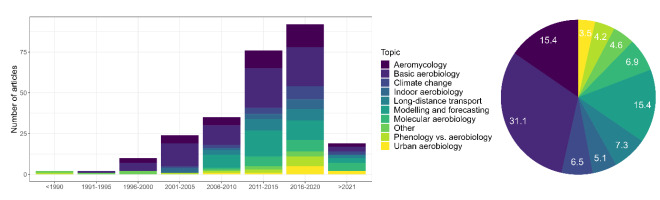
Figure 4
Percentage of papers published by Polish aerobiologists in cooperation with European scientists (n = 260, based on Scopus database).
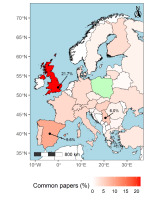
The contribution of Polish scientists to the development of aerobiology is recognized at both national and international levels. Representatives of Polish aerobiological centers have participated in several European projects, e.g., MONALISA (Monitoring Network of Allergens by Immuno-Sampling), HIALINE (Health Impacts of Airborne Allergen Information Network), and actions of European Cooperation in Science & Technology (COST ES0603, COST FA 1230, and COST CA18226). Within these projects, the novel techniques for examining airborne allergens were tested, e.g., using Coriolis and Chemvol samplers (Buters et al., 2012, 2015). The research groups in Kraków, Łódź and Lublin have actively participated in the Copernicus Atmosphere Monitoring Service CAMS_23 (https://www.regional.atmosphere.copernicus.eu), of which one of the main goals is to develop advanced models to predict the risk of pollen allergy in Europe.
Polish scientists have taken part in numerous conferences, workshops (Basic and Advanced Aerobiology Course), and international programs. They organize important aerobiological congresses (e.g., the 5th European Symposium on Aerobiology was held in Kraków in 2012) and co-organize European workshops and courses. Most importantly, they also participate in the decision-making structures of aerobiological associations. Dr. Dorota Myszkowska (Jagiellonian University, Kraków), who coordinates the Polish Aerobiology Network, is the President of the IAA (2018–2022), while Dr. Łukasz Grewling (AMU, Poznań) is the IAA Newsletter Editor (2018–2022). The board of the European Aerobiology Society (EAS) includes Dr Agnieszka Grinn-Gofroń (University of Szczecin), who is also a member of two EAS Working Groups. Polish scientists also cooperate intensively with allergy centers and medical societies, primarily the European Academy of Allergy and Clinical Immunology – within the “Aerobiology and Pollution” Working Group (https://www.eaaci.org), which combines pollen exposure and air pollution. So far, two aerobiological monographs (Weryszko-Chmielewska, 2006, 2014) and one comprehensive textbook “Aerobiologia” (Weryszko-Chmielewska, 2007), have been published in Poland.
We can confidently say that the achievements and numerous current activities are certainly promising in terms of further development of aerobiology in Poland. Indeed, some areas of research require further intensive efforts. For example, greater attention should be paid to incorporating molecular methods in aerobiology and developing a nationwide system for predicting bioaerosols concentration, similar to COSMO-ART in Switzerland (Pauling et al., 2020). Investment in state-of-art real-time automatic monitoring systems is also necessary. There is still a lot of work ahead of us, but that makes research interesting, and aerobiological research, with its vast applicability in medicine, agriculture, and cultural heritage protection, is certainly worth it.