Introduction
Pronounced intercontinental disjunction is a frequent phytogeographical phenomenon exhibited by many bryophytes at various levels of the taxonomic hierarchy. Given that taxa from different systematic groups of plants, fungi, algae, and animals also show similar disjunct distribution patterns, this phenomenon should not be viewed as a being indicative of a random event, but instead reflects a more widespread occurrence that has deeper historical and/or climatic foundations. Indeed, various hypotheses have been proposed to explain these gaps in the geographical distribution of taxa, including continental drift, long-distance dispersal, and the fragmentation of once continuous ranges.
The historical approach to studying disjunction has tended to focus on comparisons of floras; for example, between continents (Frahm,1982; Schofield & Crum,1972). More recent efforts have been based on molecular phylogenetic and population genetic analyses of focal taxa to determine whether vicariance or dispersal best explain disjunctions within and among species. Throughout the early and middle part of the twentieth century, the prevailing view was that wide disjunctions generally reflect vicariance (Ochi,1980,1981). This, however, contrasts starkly with the current view, based on a large body of studies employing molecular data, which holds that intercontinental disjunctions can typically be explained by dispersal, and that in many cases, populations distributed on separate continents are not completely or weakly isolated from one another genetically (Heinrichs et al.,2009; Shaw et al.,2014; Vanderpoorten et al.,2010).
Most bryophytes have wide geographical ranges, which in many cases are obscured by an inadequate definition of the taxa concerned and limited exploration of many regions of the globe. This is partly a consequence of the type of systematics practiced in nineteenth century, when new species were often described uncritically and in many cases simply represented different phenotypes of the same species. Accordingly, the same species are commonly known in different areas, often on different continents, under local names, and only subsequent taxonomic studies have revealed their true identities, and consequently prompted reclassification. This situation is well illustrated by many intercontinentally disjunct species. For example, although the bryophytes Bryum arachnoideum Müll. Hal. and B. huillense Welw. & Duby have long been considered African tropical endemic species (Ellis, Aranda, et al.,2013; Ellis, Bayliss, et al.,2014; Ochi,1972), they are known also in the Neotropics under the names B. impressotruncatum Herzog and Rhodobryum glaziovianum Hampe, respectively (Ochi,1980,1981). Accordingly, the phytogeographical status of these “African endemics” has been changed to Afro-American disjunct species. Similarly, Philonotis polymorpha (Müll. Hal.) Kindb. has long been known as a narrow endemic of the Antarctic Kerguelen Biogeographical Province (Ellis, Bakalin, et al.,2013; Ellis, Bednarek-Ochyra, et al.,2013); however, several local species described from sub-Antarctic South Georgia, Tierra del Fuego, southern South America, and the northern maritime Antarctic, including Ph. acicularis (Müll. Hal.) Kindb., Ph. varians Cardot, Ph. gourdonii Cardot, and Ph. luteola E. B. Bartram, have been identified as conspecific, and consequently, the Kerguelen species is now established as an amphi-Atlantic south-cool-temperate species (Ochyra et al.,2008). Furthermore, Bucklandiella angustissima Bedn.-Ochyra & Ochyra was only recently described as a new species from the South Island of New Zealand and the surrounding offshore islands, although it is also found at high elevations in the Ecuadorean Andes, where it has been known from a considerably earlier date as Racomitrium crispipilum var. brevifolium Thér (Bednarek-Ochyra & Ochyra,2011). Consequently, it is now described as an amphi-Pacific cool-temperate species.
It was early recognized that many species of bryophytes have broad geographical ranges, often spanning more than one continent (Irmscher,1929; Schofield & Crum,1972). Indeed, there is a vast literature devoted to moss disjunctions, although there have been relatively few publications that have summarized this phenomenon, and those that have been published tend to be outdated (Irmscher,1929; Schofield,1974; Schofield & Crum,1972; Schuster,1983). In older works, intercontinental disjunctions have typically been explained as a consequence of fragmentation of the supercontinent Gondwana, followed by ancient vicariance (Frey et al.,1999; Schuster,1979; Stotler & Crandall-Stotler,1974). In recent years, however, an alternative explanation, supported by a number of phylogenetic studies, has been proposed, which indicates that long-distance dispersal rather than ancient vicariance appears to be responsible for these wide disjunctions (Heinrichs et al.,2009; Lewis et al.,2014; Medina et al.,2011; Shaw et al.,2011,2014; Vanderpoorten et al.,2008). In the case of bryophytes, however, it is quite probable that disjunct distributions have originated via multiple processes, and thus it is not possible to generalize (Medina et al.,2011).
Among the different types of intercontinental disjunctions characterizing bryophyte distribution, the amphi-Atlantic distribution pattern is by far the best studied. An increasingly large number of species, currently including 78 species of liverworts (Ellis, Bakalin, et al.,2013; Gradstein,2013) and well over 80 species of moss (Ochyra & Ireland,2016), have been shown to have an Afro-American distribution. The largest group of amphi-Atlantic species consists of tropical lowland and montane moss species (e.g., Bednarek-Ochyra et al.,1999; Ellis et al.,2016; Ellis, Bayliss, et al.,2014; Frahm,1982; Müller et al.,2018; Ochyra et al.,1992,2002). The group of Afro-American disjuncts is supplemented by a small group of southern cool-temperate species (e.g., Bednarek-Ochyra & Ochyra,1998,2010,2012,2012,2013; Ellis, Aleffi, et al.,2015, Ellis, Asthana, et al.,2015; Ellis et al.,2012; Li et al.,2009; Ochyra,2010; Ochyra & Bednarek-Ochyra,2013; Ochyra & Lewis Smith,1998; Ochyra & van Rooy,2013; Ochyra et al.,2014,2015; Saługa et al.,2018). Although the Afro-American distribution of bryophyte species has traditionally been attributed to ancient tectonic events (Frahm,2003; Schuster,1983), recent molecular studies have revealed that the present-day distributions of numerous species are relatively recent and are more likely to have originated from long-distance dispersal (Devos & Vanderpoorten,2009; Gradstein,2013; Laenen et al.,2014).
In contrast to the Afro-American distribution pattern, Asian-Australasian disjunction appears to be relatively rare among mosses (Higuchi & Fife,2006). The most typical examples of this distribution pattern include Anacamptodon fortunei Mitt., which occurs in China and Japan in Asia and on Lord Howe Island off the east coast of Australia (Blockeel et al.,2009); Eccremidium minutum (Mitt.) I. G. Stone & G. A. M. Scott, which occurs in southern Australia and New Zealand in the south but has also been discovered at a single location on Honshu Island, Japan (Noguchi,1987); Bryum blandum Hook. f. & Wilson, a southern temperate species occurring in Tasmania and New Zealand and on the sub-Antarctic islands in the Kerguelen Biogeographical Province (Ochi,1972) and extending to China in Asia, where it is represented by a separate subspecies, B. blandum subsp. handelii (Broth.) (Ochi,1968); and Niphotrichum japonicum (Dozy & Molk.) Bedn.-Ochyra & Ochyra, which is widespread in East Asia (Japan, Korea, Russian Far East, continental China, and Taiwan) (Ellis et al.,2019; Frisvoll,1983) and discovered at a single location on Lord Howe Island (Vitt et al.,1993). To this small group of Asian-Australasian bryophyte species, we can now add Lewinskya graphiomitria.
In addition to the aforementioned disjunct distributions, there are several other important disjunctions relating to Asian bryophytes. Among those that have been well documented are Euro-Asian (Fedosov & Ignatova,2008; Ignatov & Ochyra,1994), East Asian-eastern North American (Iwatsuki,1972), East Asian-northern Latin American (Suárez & Ochyra,2011), and East Asian-Hawaiian (Bednarek-Ochyra & Ochyra,2017) disjunctions.
Lewinskya graphiomitria (Müll. Hal. ex Beckett) F. Lara, Garilleti & Goffinet is an epiphytic moss originally described as Orthotrichum graphiomitrium Müll. Hal. ex Beckett from the South Island of New Zealand (Beckett,1893), and was considered to be endemic to this insular country (Lewinsky,1984). It has a wide, albeit scattered, distribution throughout the South Island and is also found infrequently on the North Island (Fife,2017; Lewinsky,1984), growing mainly epiphytically on the bark of trees and shrubs (e.g., Hoheria glabrata, Aristotelia, Carmichaelia, Discaria, and Nothofagus) in areas of a high precipitation. In addition, it has also been very rarely observed growing also on rocks (Fife,2017; Lewinsky,1984).
Of the 12 species and two varieties of Orthotrichum s. l. that are currently known to occur in New Zealand (Fife,2017), six species and the two varieties are believed to be endemic to this country. Three of these belong to Orthotrichum s. str., namely, O. calvum Hook. f. & Wilson, O. angustifolium Hook. f. & Wilson, and O. aucklandicum (Vitt) Goffinet, although it is worth noting that the latter two are often placed in a separate genus, Muelleriella Dusén (Vitt,1976). The other three species and two varieties of orthotrichalean endemics are representatives of the genus Lewinskya, namely, L. graphiomitria, L. cyathiformis (R. Br. bis) F. Lara, Garilleti & Goffinet, L. sainsburyi (Allison) F. Lara, Garilleti & Goffinet, L. rupestris var. papillosa (Lewinsky) F. Lara, Garilleti & Goffinet, and L. tasmanica var. parvitheca (R. Br. bis) F. Lara, Garilleti & Goffinet.
The endemicity of the orthotrichalean mosses is lower in China where, according to Jia et al. (2011), only 10 species are endemic. Two of them belong to Lewinskya [L. erosa (Lewinsky) F. Lara, Garilleti & Goffinet and L. taiwanensis (Lewinsky) F. Lara, Garilleti & Goffinet] and eight to Orthotrichum [O. jetteae B. H. Allen, O. laxum Lewinsky, O. leiolecythis Müll. Hal., O. notabile Lewinsky, O. pulchrum Lewinsky, O. schofieldii (B. C. Tan & Y. Jia) B. H. Allen, O. subpumilum Lewinsky, and O. vermiferum Lewinsky]. A further two species, Lewinskya dasymitria (Lewinsky) F. Lara, Garilleti & Goffinet and Orthotrichum revolutum Müll. Hal., were also considered as endemic to China, but they have recently been found in Russia (Ignatov & Lewinsky-Haapasaari,1994) and Kyrgyzstan (Ellis, Aleffi, et al.,2014), respectively.
Our main aims in this paper are (i) to describe the intercontinental disjunction of an epiphytic moss species formerly considered to be endemic to New Zealand, and (ii) to establish whether there are existing similarities between the limiting bioclimatic variables in the territories of New Zealand and the remainder of the study area that would enable the species to survive here over the long term.
In this latter regard, we assume that using the concept of fundamental niche and the taking into account species’ restriction by limiting factors, are generally more meaningful approaches than applying “average factors,” and can better serve as identifying boundaries indicating the possibility of surviving or not surviving in a given territory. By extrapolating the conditions characterizing occurrence points from the collection areas in New Zealand, we can document the occurrence of these conditions in other territories, thereby providing a basic understanding of the potential for the species to spread to these areas without necessarily requiring the species to adapt to new bioclimatic conditions. The expansion into such territories is thus theoretically easier or could be directly facilitated by the existence of these conditions.
Material and Methods
Systematic Part
Lewinskya graphiomitria belongs to a group of rather robust species of New Zealand members of the genus Lewinskya F. Lara, Garilleti & Goffinet, with the height of the plants reaching 2.5−4.0 cm or, occasionally to 6.0 cm. It is easily recognized by its short-ovoid and completely smooth capsules more or less immersed in the perichaetial leaves (Figure 1). The stomata are superficial, numerous, and localized in the central and upper part of the urn. The peristome is double. The exostome contains eight pale yellow or orange teeth, which are reflexed or weakly recurved when dry. The endostome is well developed as eight wide hyaline segments which, when intact, are as tall as the exostome. They are formed by two rows of protruding cells, which are narrowed near the base. The surfaces of the teeth and segments show marked differences in their ornamentation (Figure 2). While the teeth are densely covered on both sides with tall branched papillae, which inside (PPL) form sometimes longitudinal papillose ridges, the segments are rather smooth on both sides, or inside (IPL) they are sometimes slightly ornamented with papillae situated mainly along old cell walls. The calyptra covers only about half of the capsule and it is densely covered with yellow hairs that extend over its apex. The spores are globose, finely papillose and about 20–30 µm in diameter.
Figure 1
Lewinskya graphiomitria with mature capsules (from Guo & Cao s.n., September 15, 2012, OSTR B‒2663).
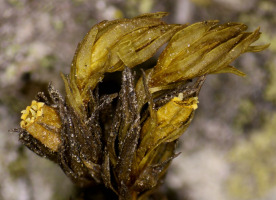
Figure 2
Scanning electron microscopy micrographs of Lewinskya graphiomitria, showing a portion of the double peristome (A), IPL (inner peristome layer) ornamentation of an endostome segment (B), PPL (primary peristome layer) ornamentation of an endostome segment (C), PPL ornamentation of an exostome tooth (D), papillae on the OPL (outer peristome layer) side of exostome tooth (E), and a spore (F). Scale bars: (A) 100 µm; (B‒C) 50 µm; (D) 20 μm; (E) 5 μm; (F) 10 μm. All from Guo & Cao s.n., September 15, 2012, OSTR B‒2663.
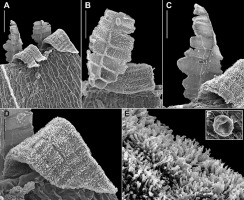
The broadly conceived genus Orthotrichum Hedw. has recently been split into four segregates, namely, Nyholmiella Holmen & E. Warncke, Pulvigera Plášek, Sawicki & Ochyra, Lewinskya, and Orthotrichum s. str. (Lara et al.,2016; Plášek et al.,2015,2016; Sawicki et al.,2017). In its traditional circumscription, Orthotrichum has recently been studied by Fife for the purposes of an online moss flora of New Zealand (Fife,2017), following the concept presented in a detailed study published by Lewinsky (1984). Currently, there are 12 species and two varieties known from this country, among which, six species belong to the genus Orthotrichum s. str. and six species and two varieties have been placed in the genus Lewinskya. In contrast, the traditionally interpreted genus Orthotrichum is almost four times richer in China, and as a consequence of a revision of herbarium collections and the collection made during several expeditions to twelve provinces, some 47 species have been identified in this large Asian country, of which 30 belong to Orthotrichum s. str., 16 to Lewinskya, and one to Nyholmiella (Skoupá et al.,2017,2018).
The specimens were studied in the herbaria of Guizhou University, Shanghai Normal University; Shanghai Museum (SM); and in the Ostrava University herbarium (OSTR). They are arranged according to the individual provinces of China. Locality data were translated from the original labels written in Chinese. The GPS coordinates follow the WGS84 system. Nomenclature fully follows currently published data presenting the polyphyly of the genus Orthotrichum s. l., which required splitting the broadly recognized genus Orthotrichum into four segregates (Plášek et al.,2015,2016; Sawicki et al.,2017). The monoicous taxa with superficial stomata were originally placed in the genus Dorcadion Lindb. (Plášek et al.,2015); however, as this name proved to be a homotypic synonym of Orthotrichum, it was subsequently replaced by Dorcadionella Plášek, Sawicki & Ochyra (Plášek et al.,2015,2016; Sawicki et al.,2017). Notably, Lewinskya antedates Dorcadionella by 7 days (Lara et al.,2016).
Specimens Examined
CHINA. Jiangxi Province. Jinggangshan City, on the border of Jinagxi and Hunan provinces, Luoxiao Mountain System, Jinggang Mt, ca. 1,000 m a.s.l., GPS: 26°37′25″ N, 114°11′10″ E, on bark of tree, April 8, 1984, leg. Dengke Li s.n. (OSTR B‒2763, herb. of Shanghai Museum SM 16651). Guizhou Province. Dejiang County, Diaokan town, Nangan Nature Reserve, ca. 1,040 m a.s.l., GPS: 28°13′48″ N, 107°51′36″ E, on bark of tree, May 2015, leg. Wei Cao s.n. (herb. of Guizhou University DJ20160523159, OSTR B‒2780); Dejiang County, Yaopenshui town, Nangan Nature Reserve, ca. 1,132 m a.s.l., GPS: 28°36′36″ N, 108°04′48″ E, on bark of tree, June 2015, leg. Wei Cao s.n. (herb. of Guizhou University DJ20160602019, OSTR B‒2779). Taiwan Province. Nantou County, Hehuan Mt, ca. 2,210 m a.s.l., GPS: 24°01′936″ N, 121°10′945″ E, on bark of tree, September 15, 2012, leg. Shuiliang Guo & Tong Cao s.n. (herb. of Shanghai Normal University 20120915462, 20120915469 & 20120915471, KRAM, OSTR B‒2663, B‒2666 & B‒2667).
NEW ZEALAND. Canterbury. Arthur’s Pass, May 1889, leg. Beckett, No. 176 (CHR, OSTR); Arthur’s Pass, ca. 790 m a.s.l., GPS: 42°56′54″ S, 171°33′40″ E, on bark of tree, February 19, 2013, leg. V. Plášek, s.n. (KRAM, OSTR); Ben More, November 1893 – Iso-syntype (CHR). Nelson. Hira Forest, ca. 115 m a.s.l., GPS: 41°16′15″ S, 173°21′15″ E, on bark of tree, March 6, 2013, leg. V. Plášek, s.n. (KRAM, OSTR). Otago. Mt Cargill, ca. 650 m a.s.l., GPS: 45°48′48″ S, 170°33′17″ E, on bark of tree, February 27, 2013, leg. V. Plášek s.n. (KRAM, OSTR). Southland. Homer Tunnel, on Hoheria in open grass field, February 14, 1980, leg. Lewinsky No. 2104 (CHR, OSTR).
Quantification of Extrapolated Projection
For projection into unrecorded areas between New Zealand and the remainder of the study area (Australia, Brunei, Burma, Cambodia, China, Darussalam, the Democratic People’s Republic of Korea, Fiji, Indonesia, Japan, the Lao People’s Democratic Republic, Malaysia, New Caledonia, Papua New Guinea, the Philippines, the Republic of Korea, Solomon Islands, Taiwan, Thailand, Timor-Leste, Vanuatu, and Vietnam), we used 25 presence points [nine GBIF data, 16 data from Lewinsky (1984)] from New Zealand to identify environmental conditions theoretically suitable for the studied species. For analysis purposes, we then used 50-km buffers around presence points to characterize the specific bioclimatic conditions suitable for L. graphiomitria. Buffer zone size was determined on the basis of previous assessments of buffers of 25, 50, 75, and 100 km. Among these, from the perspective of the effort required to specify a broader fundamental niche of the species based on selected limiting factors, the 50-km buffer appeared to be optimal for capturing the environmental conditions characterizing presence points from neither too large nor too small an area.
For the purposes of the analysis, we selected bioclimatic layers of WorldClim version 2 (http://worldclim.org/version2) (Fick et al.,2017) at a resolution of 30 arcsec (approx. 1 km). For the initial comparison (Figure 4), we used maximal and minimal temperature and precipitation data (BIO5 ‒ maximal temperature of the warmest month, BIO6 ‒ minimal temperature of the coldest month, BIO13 ‒ precipitation of the wettest month, and BIO14 ‒ precipitation of the driest month). These layers were selected as “annual average” factors, given that average temperatures or average rainfalls are of little biological relevance to the occurrence of the species. In contrast, maximal and minimal factors could serve as limiting factors influencing survival in new areas.
Subsequently, we selected layers based on the previous research of Číhal et al. (2017), who focused on the family Orthotrichaceae (Bryophyta) in Tajikistan and Kyrgyzstan. We selected three variables, previously evaluated as the most important for the studied family, namely, BIO2 ‒ mean diurnal range [mean of monthly (maximal temperature – minimal temperature)], BIO6 ‒ minimal temperature of the coldest month, and BIO13 ‒ precipitation of the wettest month. The second set of variables is in good agreement with the layers selected to serve as limiting factors. Nevertheless, even though the two sets of layers differ by only one bioclimatic layer, the results obtained can be significantly different (Figure 5).
To assess the structure of the data, we also used the dudi.pca function from the ade4 package (Dray et al.,2007), which enabled us to perform a PCA analysis and to evaluate the data for potential outliers (isolated points or points distant from others) that could negatively influence further analysis. After inspection, it became apparent that there were no “outlier” points in the data; however, PCA analysis revealed that the BIO14 layer was correlated with the BIO13 layer in the first set of layers, and therefore we elected to discontinue using BIO14 in the analysis. Our selection of layer BIO13 for further analysis is consistent with the approach used by Číhal et al. (2017). In an effort to detect any to any hidden correlation, we also used the vifcor function from the usdm package (Naimi et al.,2014), with the correlation factor set to r = 0.7. However, this function failed to identify any hidden correlation. The R program (R Development Core Team,2018) was used for these analyses.
Our own analysis comparing environmental layers between the study areas was carried out using the ExDet tool (Mesgaran et al.,2014) algorithm based on the Mahalanobis distance, which shows novel combinations between covariates and reveals types of novelties between environmental layers and studied areas. In this regard, there are two types of novelties that can be obtained, namely, type NT1, which indicates areas in the projection space with at least one climate covariate outside the univariate range of the reference data, and type NT2, which indicates areas that are within the univariate coverage of the reference data, but represent nonanalogous covariate combinations. Areas that do not show either NT1 or NT2 novelties are similar to the reference data, as they both fall within the range of reference covariates and capture the same covariate combinations (Mesgaran et al.,2014).
Results
During a revision of the specimens maintained in the aforementioned herbaria in 2018, we identified certain interesting undetermined specimens of an orthotrichalean moss that did not correspond to any species known in China at that time. On the basis of a detailed study of comparative material from around the world, these specimens were identified as Lewinskya graphiomitria, a species that has hitherto been considered endemic to New Zealand. This is not only the first record of this species in China and the entire Asian region but also its first discovery from any locality outside New Zealand. The discovery of L. graphiomitria in Asia thus fundamentally transforms the phytogeographic status of this species, which is now considered representative of species showing a rare type of Asian-Australasian distribution pattern (Figure 3).
On the basis of analysis performed using the ExDet tool (Mesgaran et al.,2014), we were able to map areas that do not show NT1 (climatic covariates outside the univariate range) or NT2 (nonanalogous covariate combinations) novelties (Mbatudde et al.,2012; Pradhan et al.,2012), which indicates that the bioclimatic variables used as limiting factors do not show any extrapolation from the territory in New Zealand to the remainder of the study area in the zones highlighted on the map shown in Figure 4 as green ranges. These green zones indicate areas characterized by conditions that would be suitable for the existence of L. graphiomitria, and in which the species could survive and spread. In contrast, the red and blue zones shown in Figure 4 indicate those areas that are outside the appropriate ecological amplitude of this moss.
In this study, we performed a comparison of bioclimatic variables based on (i) general rules, in which limiting factors play a more important role in determining the occurrence of species (Figure 4), and (ii) based on previous research on the family Orthotrichaceae in Asia (Figure 5).
Discussion
Many bryophyte species, even those that are sterile, are characterized by wide transcontinental ranges (Devos & Vanderpoorten,2009; Gradstein,2013; Laenen et al.,2014). Conversely, there are numerous examples of species that have very limited distributions, despite producing prolific quantities of diaspores (Lewinsky,1984). For Lewinskya graphiomitria, long distance dispersal is the most likely means of transfer that would account for its disjunct distribution, even though it could partly be attributable to stepping-stone migration through Australia and/or New Guinea. To examine this issue, we initiated a study based on a comparison of projections into novel covariate space between localities in New Zealand and the remainder of the study area.
The sporadic occurrence of New Zealand endemic species in China would certainly be considered as interesting example of accidental intercontinental bryophyte spread. However, the fact that L. graphiomitria has been recorded repeatedly in four localities in China tends to suggest that the distribution of this species is an example of a confirmed areal disjunction. New Zealand and China include locally similar landscapes, with comparable climatic conditions, high precipitation, and heterogeneous topography. Moreover, our analysis indicates that the occurrence of L. graphiomitria may not only be limited to territories in China and New Zealand. At a number of localities between these two remote areas, and also in Japan (in the case of second set of variables), there are further areas with similar climatic conditions and comparable geomorphology, where occurrence of the species can be predicted (Figure 4 and Figure 5).
On the basis of our analyses, we detected no extrapolations between environmental layers for certain regions of the studied area, particularly in South Australia, South China, Thailand, and parts of Papua New Guinea and Japan. In China, this corresponds to the newly discovered area of knowledge regarding the distribution of L. graphiomitria. At present, we can assume that there are no limiting factors that would prohibit population spread in these areas. This contrasts with those areas that show extrapolations, which could be indicative of suppressive environmental or physiological barriers that would prevent populations survival in these localities. Given that at a regional scale, approximately 1% of the ambient spore rain has a trans- or intercontinental origin (Sundberg et al.,2006), such barriers can also play a significant role as a strong homogenizing force that prevents the differentiation and adaptation of local populations (Vanderpoorten et al.,2010). This “anti-adaptation” force could also provide evidence for the important limiting role of environmental factors as barriers to population spread over regions receiving spore rain but lacking satisfactory environmental conditions.
In the present study, we refrained from predicting the actual distribution of L. graphiomitria, owing to a lack of relevant data. Instead, we simply wished to highlight that there are no extrapolations with respect to limiting conditions between New Zealand and China for the studied species, which could explain the distribution of the newly discovered populations and their long distance dispersal between these areas.
We believe that our research can serve as a guide for more detailed analyses of the distribution of L. graphiomitria and collection of data in the field. This in turn could contribute to gaining a better understanding of the occurrence of this species and thereby enable the use of some of the available “environmental niche modeling” methods, which as yet is unfeasible owing to insufficient presence data.
Handling Editor
Attila Molnár V.; University of Debrecen, Hungary; https://orcid.org/0000-0001-7096-9579
Authors’ Contributions
This research was completed by the collaboration of authors in roles of conceptualization, VP and ZK; methodology: VP, ZK, LČ, and SG; software: LČ and LF; validation: VP; formal analysis: VP; investigation: ZK and VP; resources: SG, LF, and VP; data curation: VP; writing – original draft preparation: VP, ZK, LČ, and LF; writing – review and editing: VP; visualization: LČ and LF; micro- and macrophotography: VP; supervision: VP and SG; project administration: VP and LF; funding acquisition: VP.