. Introduction
Pitaya (Hylocereus sp.) is one of the crops with high nutritional and economic value. However, pitaya trees and fruits are often infected by many microorganisms during cultivation, harvesting, and preservation. Many microbial pathogens, such as Neoscytalidium dimidiatum, Colletotrichum gloeosporioides, and Alternaria sp., have caused great losses in yield and fruit quality. Recently, Gilbertella persicaria was discovered as the cause of dragon fruit rot in Thu Thua (Long An Province, Vietnam). Previously, G. persicaria was detected to cause fruit rot of pitaya for the first time in Taiwan in 2009 (Lin et al., 2014) and was found in 2011 in Yunnan, China (Guo et al., 2012).
The disease caused by G. persicaria has been a relatively serious disease of dragon fruit. The disease usually appears on fruit from three to five days after harvesting with such symptoms as small streaks then developing into soft rot. In addition, this fungus also infected parts of pitaya trees in the field with the appearance of black or brown spots on young fruit, flower stalks, and flower buds, leading to abnormal growth and premature fall (Lin et al., 2014). This disease usually occurs in the rainy season or in wet conditions (Lin et al., 2014). Besides, Guo et al. (2012) also reported that G. persicaria sporangia were easily ruptured and released spores when exposed to water. Thereby, it could be seen that the humid tropical climate with high rainfall in Vietnam was favorable for this fungus to grow and cause harm. G. persicaria was also found to cause disease on ex vivo pitaya branches by Taba et al. (2011) and on branches in the pitaya field by Lin et al. (2014), which showed the potential risk of disease outbreaks caused by G. persicaria in pitaya farms.
Up to now, the approach to help control diseases caused by G. persicaria on crops, including pitaya, has been still limited. On pitaya, the main solutions to reduce the damage caused by this pathogenic fungus included farming practices, such as pruning infected branches and flowers (Lin et al., 2014). The application of UV light for pre-storage fruit treatment also reduced the damage caused by G. persicaria (Aborisade et al., 2017). Sipiczki (2006) reported that the yeast strain Metschnikowia pulcherrima could inhibit many fungi, including G. persicaria. Recently, Taba et al. (2021) showed that some strains of Bacillus subtilis could control the disease caused by G. persicaria on ex vivo pitaya stems with a similar efficiency to that of chemical treatments. Through the above studies, it can be seen that microbial antagonists were a potential approach to control G. persicaria.
The objective of this study was to isolate, screen, and evaluate the potential application of rhizobacteria through their ability to inhibit G. persicaria in vitro and ex vivo.
. Material and methods
Microorganism
G. persicaria GTC2.3.1 was supplied by Giatuong Co. Ltd. (Vietnam). This strain was collected from a sample of pitaya fruit from the farm of Truong Minh Tuong (Long An Province). This fungal strain was cultured on slant potato dextrose agar for 7 days in the dark at 33–35 °C for spore production.
Soil samples
Soil samples were taken from the rhizosphere of pitaya plants in Dong Nai, Tien Giang, and Long An Provinces in Vietnam. For each sample, about 500 g of both soil and roots was collected with a sterilized shovel after the surface soil layer was removed. The sample was stored in a sterile nylon bag in a cool container and brought to the laboratory. The soil samples were dried at room temperature for 2–3 days, sieved through a 2–3 mm sieve, and then thoroughly mixed before isolation (Shinkafi & Gobir, 2018).
Ex vivo pitaya tissues
Samples of pitaya tissues were taken from the cultivation farms in Dong Nai, Tien Giang, and Long An Provinces. The selected fruits were healthy, ripe for harvesting, and relatively uniform in size and color. For stem segments, tops of 30-day-old stems were taken from disease-free pitaya plants.
Pathogenicity test for G. persicaria
Pitaya fruit and stem tissues were surface decontaminated as described by Zhang et al. (2019) with some modifications before being used to test the pathogenicity of G. persicaria GTC2.3.1. The fruits and stems were decontaminated by soaking in a 2% (v/v) NaClO solution for three minutes, rinsed with sterile water, and dried at room temperature. Wounds (5 × 5 mm) on fruit peels, bracts, and stems were made with sterile medical blades. The minimum wound depth was two mm (Taba et al., 2021). The suspension of G. persicaria GTC 2.3.1 spores (106 spores ml−1) was made with physiological saline from 7-day-old cultured slant agar. One hundred µl of the fungal spore suspension was inoculated into each wound. Wounds only injected with physiological saline were the control. After that, the pitaya tissues were preserved in sterile containers and kept moist. Each treatment was repeated three times, and each time it consisted of 15 wounds, five wounds per sample of pitaya tissue. After 7 days of infection, the symptoms caused by G. persicaria and the rate of wounds showing the disease were recorded. Infected wounds showing symptoms of the disease were used to check for the presence of the pathogenic fungus by isolation and morphologic observation of fungal colonies on potato dextrose agar plates and under optical microscopy to confirm the results.
Isolation of G. persicaria antagonizing bacteria
Isolation was performed according to the method described by Nguyen and Le (2021) with some modifications. The fungal spore suspension (100 µl, 106 spores ml−1) was spread on plates containing potato dextrose agar medium. Then, the prepared rhizosphere soil was sprinkled directly on the surface of the plates inoculated with the fungus. After 2–3 days of incubation at 33–35 °C, streaks of bacterial biomass with the inhibitory zone of the growth of the surrounding fungal pathogens were selected. The selected bacterial biomass was streaked to form individual colonies on Luria Bertani agar. The pure colonies were inoculated on Luria Bertani slant agar for further study.
Evaluation of the antagonistic efficiency of bacterial isolates
The ability of bacterial isolates to antagonize the disease fungus was determined with the dual culture method according to Selim et al. (2016). The fungal spore suspension was spread on potato dextrose agar (100 µl per plate, 106 spores ml−1). Then, each bacterial isolate was inoculated as dots on a fungal plate and incubated at 30–33 °C for three days. Each bacterial isolate was evaluated on separate plates and repeated three times. The antagonistic efficiency was determined based on the diameter of the inhibitory zone and the ratio between the diameter of the inhibitory zone and the diameter of the bacterial growth zone.
Identification of the selected isolates using the MALDI-TOF technique
The bacterial isolates were sent for MALDI-TOF identification at the Center for Science and Biotechnology (University of Science, Vietnam National University, Ho Chi Minh City). The MALDI-TOF identification technique is based on the detection and comparison of the specific protein spectrum of a microorganism with previously published protein spectra database to confirm the similarity of the identified microorganisms with microorganisms in the database.
Evaluation of the ability of selected bacterial isolates for preparation of antagonistic consortia
The selected bacterial strains were evaluated for compatibility according to Kannan et al. (2021) with some modifications. Two-day-old biomass of selected bacterial strains was streaked in lines perpendicular to each other on a plate containing Luria Bertani agar. The plates were then incubated at 30–33 °C. For each pair of the bacterial strains, the experiment was repeated three times, with three plates each time. After 48 h of incubation, the formation of an inhibitory zone at the junctions was observed. Two bacterial strains were considered compatible when there was no inhibitory zone at the intersection.
The ability of combinations of compatible bacteria to antagonize G. persicaria was evaluated with the slightly modified well diffusion method described by Lin et al. (2009). The bacterial strains were cultured in erlens containing 200 ml of Luria Bertani broth under shaking at 200 rpm for 48 h at 30–33 °C. The fungal spore suspension was spread on potato dextrose agar (100 µl per plate, 106 spores ml−1). A well was created at the center of each fungal plate. One hundred µl of a suspension consisting of compatible bacterial broths in equal volumes was inoculated into the well. The antagonistic ability of individual cultures of bacterial strains was also evaluated. The plates were then incubated at 30–33 °C. The experiment was conducted in triplicate, with six plates each time. Free-cell mixture filtrate of the bacterial mixture was used as the control. After three days of incubation, the diameter of the inhibitory zone and the ratio of the inhibitory zone diameter to the diameter of the bacterial growth zone were determined.
Biocontrol efficiency of the bacterial consortia on ex vivo pitaya tissues
The ability of the selected bacterial strains to control the disease caused by G. persicaria in the ex vivo pitaya fruits was determined according to Zhou et al. (2011) with some modifications. The compatible bacterial strains were individually cultured in erlens containing 200 ml of Luria Bertani broth under shaking at 200 rpm for 48 h at 30–33 °C. Pitaya fruits were decontaminated and wounds were created before being inoculated with 100 µl of a mixture of the bacterial broths in equal volumes. After 30 minutes, 100 µl of the G. persicaria GTC2.3.1 spore suspension (106 spores ml−1) was added to the wound. The treatment consisting in replacing bacterial culture with Luria Bertani Broth medium was used to eliminate the effects of the ingredients in Luria Bertani Broth. The controls were injected with the free-cell filtrate of the respective mixtures into the wound to eliminate the effects of the ingredients in Luria Bertani broth and compounds secreted by bacteria in the post-enrichment cultures. The blank was injected only with the spore suspension. Each treatment was repeated three times, each time consisting of three fruits and five wounds per fruit. After seven days, symptom presentation and percentage of symptomatic wounds (if any) were recorded.
. Results
Pathogenicity test for G. persicaria GTC2.3.1
After seven days of infection with G. persicaria GTC2.3.1, the wounds on the fruit peels formed unusual symptoms, compared with the control. The wounds appeared wet, soft, and black or brown at the edges (Figure 1a). In turn, the wounds in the control were still fresh and naturally pink and the wound mouth was hard and succulent. All of the wounds on the fruit peels showed signs of rot (Table 1). The lesions on the stem segments were similar to those on fruit peels. However, the wounds on the stem segments had different symptoms and were less obvious than the wounds on the fruit peels. The wounds on the stems were yellow to brownish-black with withered edges, and some wounds appeared spreading yellow around (Figure 1b). In contrast, the wounds on the bracts did not show any abnormal signs, compared to the control. The wounds on the bracts were still fresh, dry, without discoloration, and no appearance of mycelia (Figure 1c).
Isolation, screening, and identification of bacterial antagonists against G. persicaria GTC2.3.1
In this study, eleven soil samples collected on the pitaya cultivation farms in Dong Nai, Tien Giang, and Long An Provinces were used to isolate antagonizing bacteria (Table 2). All of the soil samples showed streaks of bacterial biomass inhibiting the growth of G. persicaria GTC2.3.1 on the isolation plates (Figure 2). Eighty-nine isolates were collected after streaking and purification. In general, all the soil samples showed the presence of potentially antagonistic bacteria; however, the number of bacterial isolates obtained from each soil sample was not the same (Table 3).
Table 2
Coordinates of collected soil samples.
Figure 2
Presence of bacterial growth zones inhibiting the growth of G. persicaria GTC2.3.1 on isolating dishes from some soil samples (red arrows). Bar = 1 cm.
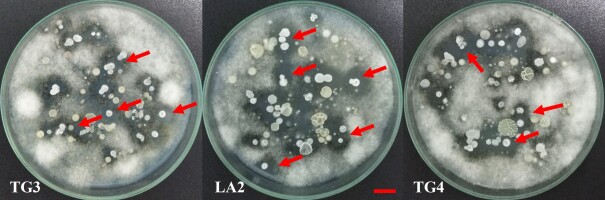
Table 3
Collected bacterial isolates.
The isolates were evaluated for their ability to antagonize G. persicaria GTC2.3.1 on potato dextrose agar with the dual culture technique (Figure 3). All of the eighty-nine isolates were capable of antagonizing G. persicaria GTC2.3.1. The results of the top fifty isolates ranged from 1.81 to 2.47 and showed statistically significant differences between the bacterial isolates (Table 4). The ratio between the diameter of the inhibitory zone and the diameter of the bacterial growth zone also differed statistically significantly. However, the order of ranking according to the diameter of the inhibitory zone was different from that assessed according to the ratio between the diameter of the inhibitory zone and the diameter of the bacterial growth zone.
Table 4
Top 50 G. persicaria GTC2.3.1 isolates antagonized with high inhibition.
The selected bacterial isolates, including LA2.9, LA3.2, LA4.5, and LA6.1, were sent for identification with the MALDI-TOF technique at the Center for Bioscience and Biotechnology (University of Sciences, Vietnam National University - Ho Chi Minh City). The identification results showed that all of the four isolates had high similarity with the same genus Bacillus (Table 5).
Evaluation of the compatibility of selected bacterial strains
All of the four bacterial strains selected in this study were compatible with each other after two days of testing according to the cross-streak method described by Kannan et al. (2021). There were no signs of an inhibitory zone at the intersections (Figure 4).
Anti-fungal ability of bacterial consortia against G. persicaria GTC2.3.1
Nowadays, many microbial product formulations contain simultaneously multiple strains of microorganisms. Combining multiple microbial strains will exploit the interactions between the microbial strains to enhance the target activity or make the formulation more diverse. In addition, different microbial strains are genetically diverse, which makes the formulation applicable to a variety of field conditions. In this study, different combinations from four selected bacterial strains were applied to evaluate the antagonistic efficiency against G. persicaria GTC2.3.1 on potato dextrose agar. In general, the efficiency against G. persicaria GTC 2.3.1 of the combinations tended to increase (Figure 5). The combinations of Bacillus sp. Bacillus sp. LA3.2 – Bacillus sp. LA6.1, Bacillus sp. LA2.9 – Bacillus sp. LA4.5 – Bacillus sp. LA6.1, and Bacillus sp. LA2.9 – Bacillus sp. LA3.2 – Bacillus sp. LA6.1 exhibited a higher antagonistic efficiency with a diameter of the inhibitory zone significantly different from that in the treatments using a single strain.
. Discussion
The symptoms expressed in the wounds on the fruit peels infected with G. persicaria GTC2.3.1 were similar to those on G. persicaria-infected pitaya fruits on the field described by Lin et al. (2014). In the case of the stem, Taba et al. (2011) reported similar symptoms caused by the G. persicaria (Eddy) Hesseltine strain. In this study, although the rate of diseased wounds was similar, the severity was higher on the fruit peels than on the stem segments. This may be because stems are less succulent and contain lower amounts of nutrients than the fruit peels. In addition, the structure of the stem is stiff with more fiber. Similar to the stem, bracts are organs with a hard structure and little nutrition, which was probably one of the reasons why it was difficult to be infected by the fungus. Moreover, bracts are often treated with such compounds as auxin and cytokinin during cultivation to keep them green and increase the aesthetic value of the fruits. Some previous studies demonstrated that auxins and cytokinins inhibited the growth of some fungal pathogens, as discussed by Gupta et al. (2020) and Sankar et al. (2012). Therefore, perhaps this was also the factor that helped the bracts resist the attack of G. persicaria GTC2.3.1. However, to date, there have been no reports evaluating the ability of auxins and cytokinins to inhibit G. persicaria.
In this study, four isolates, including LA2.9, LA3.2, LA4.5, and LA6.1, ranked highest in both evaluation criteria. The diameter of the inhibitory zone of these four isolates ranged from 2.33 to 2.47 cm. Previously, studies using microorganisms antagonizing G. persicaria were very limited. Sipiczki (2006) reported that the yeast strain Metschnikowia pulcherrima was able to inhibit G. persicaria with the diameter of the inhibitory zone of 3.5 to 4.5 mm, four to five times lower than these four isolates. In turn, studies on the application of bacteria in the control of other phytopathogenic fungi are very popular. However, the diameter of the inhibitory zone of the selected bacterial isolates in this study was also higher than that of many selected isolates in previously reported studies. For instance, the isolates selected by Selim et al. (2016) had the inhibitory zone of 0.41, 0.43, and 0.49 cm against Fusarium solani, Rhizoctonia solani, and Erwinia carotovora, respectively. The selected bacterial isolate in the study conducted by Nguyen and Le was able to antagonize Phyllosticta cavendishii with the highest diameter of the inhibitory zone of only 1.95 cm.
On the other hand, when comparing the ratio between the diameter of the inhibitory zone and the diameter of the bacterial growth zone, the bacterial isolates selected in this study had this ratio ranging from 4.13 to 4.39, equivalent to the selected bacterial isolate in the study reported by Nguyen and Le (with this ratio reaching 4.29). Through the above analysis, it could be seen that G. persicaria could be easily inhibited by many bacteria, thereby showing great potential in using bacteria to control this fungal disease.
Many previous reports also isolated Bacillus capable of antagonizing various phytopathogenic fungi. Isolate P10 could antagonize fungal phytopathogens, including Verticillium dahliae, Botryosphaeria dothidea, and Fusarium spp., on tomato and was identified as belonging to Bacillus subtilis, as reported by Pei et al. (2021). Oh et al. (2021) also isolated and identified strain B. wiedmannii DDP257 with multi-resistance to ten different phytopathogenic fungi. Through the above examples, the common presence as well as the potential of Bacillus in controlling phytopathogenic fungi could be seen. This may be related to the common presence of Bacillus spp. in a wide variety of natural environments (Radhakrishnan et al., 2017). In addition, most species of the genus Bacillus have various mechanisms to inhibit fungal growth, such as biosynthesis of antifungal peptides, secretion of fungal cell wall hydrolytic enzymes, and competition for nutrients and living space (Kang et al., 2015). Furthermore, some previous studies showed that Bacillus was more effective against phytopathogenic fungi than other bacteria, as discussed by Karim et al. (2018), Murtado et al. (2020), and Yadav et al. (2021).
Previously, two species B. mojavensis and B. subtilis were also collected by many researchers. It was noteworthy that these two species could antagonize various fungal species, as discussed by Haiyan et al. (2016), Nair et al. (2002), and Prajakta et al. (2019). In addition, B. mojavensis and B. subtilis have been shown to have activities that help promote plant growth, such as the production of hydrolytic enzymes, biosynthesis of phytohormones, etc. (Anguiano et al., 2019; Hashem et al., 2019; Maheshwari et al., 2020; Makhlouf et al., 2023; Prajakta et al., 2019; Sumi et al., 2022). The above analysis confirmed the potential application of the four selected antagonizing Bacillus strains in this study. In addition, these results again suggested that B. mojavensis and B. subtilis were common antagonistic microorganisms in soil. However, additional identification analyses have to be performed to confirm the species for appropriate research and application.
Previously, there have been many studies evaluating the compatibility of antagonizing bacterial strains to establish bacterial consortia that could control phytopathogenic fungi. Irabor and Mmbaga (2017) evaluated the compatibility of seven bacterial strains with the ability to antagonize Phytophthora capsici and showed that only two of them were compatible. Three out of six pairs of bacteria capable of antagonizing Fusarium oxysporum were also shown to be compatible (Widyantoro et al., 2019). Recently, Hadi et al. (2021) also evaluated the compatibility of seven bacterial strains with the ability to antagonize F. oxysporum and noted that none were compatible with all six remaining strains. Through the above evidence, it could be seen that the compatibility varies with each bacterial strain. The compatibility of antagonizing bacterial strains might enhance the ability to biocontrol phytopathogenic fungi, as reported by Palmieri et al. (2016) and Tsalgatidou et al. (2023). All of the four bacterial strains selected in this study were compatible, so they could form ideal combinations to be applied to control G. persicaria GTC2.3.1.
Many previous reports showed that the application of a combination of microbial strains could enhance the target activity, compared with the use of single strains, for instance, bacterial consortia capable of biosynthesizing indole acetic acid (Widhiya & Suharjono Ardiyati, 2016), solubilizing ZnO and ZnCO3 (Roshani et al., 2020), solubilizing P (Baas et al., 2020), etc. The anti-fungal activity was also one of the reported activities that could be enhanced by applying combinations of bacterial strains instead of using single strains, such as the antagonistic ability of Fusarium oxysporum (Fujiwara et al., 2016 ), F. oxysporum and Fusarium sp. (Devi et al., 2018), Rhizoctonia solani (Yin et al., 2022) etc. However, combined bacterial strains could also reduce or eliminate the target activities of single bacterial strains, as reported by Mülner et al. (2019) for Sclerotinia sclerotiorum antagonistic activity, Roshani et al. (2020) for P solubilizing activity and indole acetic acid biosynthesis, or Alfiyah et al. (2021) for nitrogen fixation ability, etc. In this study, some combinations had lower antagonistic activity against G. persicaria GTC 2.3.1, while some combinations showed enhanced antagonistic ability compared with the single strains. This might be related to the competition for growth conditions among strains in the combination or secondary compounds produced during the life process in certain conditions that inhibited each other among bacterial strains (Wu et al., 2020). In this study, the presence of G. persicaria GTC 2.3.1 on the agar plate could affect the interactions between the strains in the combination, inhibiting or competing instead of being compatible, as shown in Figure 4.
. Conclusions
Gilbertella persicaria GTC2.3.1 was used as the target pathogenic fungus because this fungus caused disease on the branches and fruits of pitaya with similar disease symptoms in the field. Four isolates, including LA2.9, LA3.2, LA4.5, and LA6.1, were screened from 89 bacterial isolates collected from 11 rhizosphere soil samples, based on inhibitory zone diameters (from 2.33 to 2.47 cm) and the ratio between the diameter of the inhibitory zone and the diameter of the bacterial growth zone (from 4.13 to 4.39). All the four bacterial isolates were compatible with each other and were found to belong to the Bacillus genus. Some combinations of the bacterial strains when applied together could help enhance the control of Gilbertella persicaria GTC2.3.1 on agar plates, showing the potential to develop consortium formulas from these strains. However, the field efficiency and the safety of these consortia need to be assessed before their practical application.