. Preface
Paleobotany is a particular branch of science that combines botany and geology, reaching the deep past of Earth’s history. Each trace or remnant of plants preserved in rock can be examined; they contain information on the planet’s vegetation, from the most primitive organisms to the most advanced forms. Based on taxonomy, individual plants are grouped into assemblages and floras corresponding to the paleogeography, topography, and climate of the habitat. Paleobotany examines details of plant anatomy, including ultrastructure and morphology, ecology, global floristic changes, extinction, survival, and evolutionary processes. Exceptional plant remains can be used as index fossils in stratigraphy or, according to their adaptive mechanisms, as climate indicators. In the past 100 years, many changes in paleobotany research have occurred. The new paleobotanical worldview spans modern taxonomy, anatomical studies, floristic and palaeoecological investigations that use statistics and simulative modeling, and studies of plant adaptation mechanisms and plant–climate relationships. Complementing the work on macrofloras, palynology is an increasingly relevant tool employed in stratigraphy and is used to reconstruct climatic conditions during long geological time sequences.
The techniques and methodology of paleobotany have transformed over the course of a century. The development of computers and computer programs has improved the compilation and interpretation of data and the depth of statistical analyses and advanced modeling and the reconstruction of climate and entire ecosystems. The substantial progress in photography and its universal availability has increased the ease of documentation and revealed hidden details. Photographic films and traditional cameras fitted on microscopes provide digital photography and digital cameras connected to computers. Microscopy can take advantage of various techniques, namely, differential interference contrast, epifluorescence, scanning electron microscopy, transmission electron microscopy, and confocal microscopy. Computerized axial tomography allows researchers to observe remains embedded in rock, visualize internal structures, create three-dimensional reconstructions of plant organs or whole plants, and follow their evolutionary development. These tools and many others have vastly improved the quality of paleobotanical research.
The aforementioned aspects are merely a few aspects of the technological progress that have allowed interdisciplinary research and have led to further development. Polish paleobotanists have always endeavored to remain abreast of technical innovations and use all the opportunities thus created. The results of their research are on par with those of Europe’s other leading paleobotany centers. To illustrate progress in Polish paleobotany, we selected some notable and valuable studies.
. Introduction
After the oldest eons, such as Hadean, Archean, and most of the Proterozoic, which were not investigated from a paleobotanical view, the Paleozoic was the first and longest era fully documented by a paleobotanical record. It was divided into five periods and lasted approximately 250 million years (all geological periods and stages with their duration years are included in the International Stratigraphical Chart; Figure 1). Diverse plant microfossil remains have been preserved, since the Cambrian, the oldest Paleozoic period (and the pre-Paleozoic period, Precambrian). The high potential for satisfactory preservation shows that the oldest microfossils, acritarchs, occur in the marine environment and are then used in the stratigraphy of marine deposits. Similarly, spores and pollen grains are usually produced in large amounts by terrestrial cryptogams and gymnosperms, respectively, and are useful in terrestrial stratigraphy and paleoecology. Research on these microfossils has developed extensively during the last century. Palynological investigations have been performed in several directions, and we present those in this paper, showing the variety of methods used. In palynology, the most important methods and studies are routine, similar in type, and performed worldwide. Taxonomy is the basis for all paleobotanical and palynological studies because only well-determined taxa provide a solid output source. Most of the literature combines taxonomical works with biostratigraphic or paleoecological conclusions. However, there are also strict taxonomic studies that are often time-consuming and laborious, and receive less attention than other, spectacular aspects. In addition to the classic morphological descriptions, extended taxonomic revisions are performed in accordance with modern requirements, which provide a more exact basis for biostratigraphy.
Biostratigraphy is based on microfossils such as acritarchs, spores, and pollen grains. Their assemblages may have a limited presence in time and can be used for dating rocks representing geological strata. The advantage of using microfossils is that small fragments of rock samples, such as slabs from drilling cores, can contain thousands of records and preserve them much better than plant macrofossils can. Zonal schemes established for marine or non-marine successions based on palynological analyses often correspond to invertebrate zones. Palynostratigraphy is a type of research in the local range but is of significant importance because knowledge of the stratigraphic structure of rocks is essential for each country.
The other crucial aim of palynological studies is to detect climatic and environmental changes by interpreting the spectra of microfossil occurrences and their changes along stratigraphic profiles. Dynamics in the appearance and disappearance of microfloral taxa are usually caused by environmental events, which allows for the reconstruction of time-related changes in habitat and climate. The next aim is a rough reconstruction of plant cover in a given territory where macro remains are absent or there are complementing data on vegetation based on macro remains (mainly leaves).
. Actitarchs
Taxonomy
Acritarchs are one of the few groups of investigable small organisms with organic walls, forming a polyphyletic group with unknown affinity (Figure 2A–C). They appeared in the Precambrian, 1.4 billion years ago, and occurred in shallow-marine environments. During the Cambrian, acritarchs underwent rapid evolution, leading to an increase in the variety of forms and development of assemblages with different morphologies. Because of this differentiation over time and the rapid change in acritarch associations, they were used to establish detailed zonations within the Cambrian. These microfossils have been considered by the International Commission on Stratigraphy, as the only available stratigraphic tool for the oldest Paleozoic marine deposits, often devoid of other fossils (e.g., trilobites, widely used in dating). Taxonomic studies on acritarchs were initiated by a researcher in the United States, Evitt (1963a, 1963b), and subsequently by others in Poland. Górka (1969) first described 50 species in the Polish Ordovician. Polish studies continued on the Cambrian acritarchs, by Moczydłowska (1981, 1988, 1991), Moczydłowska and Vidal (1986), and Jachowicz-Zdanowska (2011a, 2011b, 2013, 2014); on the uppermost Ordovician/lowermost Silurian acritarchs and prasinophyceans from the southern part of the Holy Cross Mountains, by Kremer (2001); and those on Silurian, by M. Jachowicz (2000). Among the palynologists who have conducted taxonomical studies of acritarchs are Dybová-Jachowiczowa, Jagielska, and Turnau (for details and references see Turnau & Filipiak, 2003). Silurian coccoid cyanobacteria from the Bardo Mountains, Sudetes, were described by Kremer (2006); Lower Devonian eukaryotic algae from the Bukowa Góra (Holy Cross Mountains), by Filipiak et al. (2021); and Devonian acritarchs, by Kondas et al. (2022).
Stratigraphy and Paleoecology
A study of the oldest acritarchs in Poland in the palynostratigraphic aspect was conducted by Moczydłowska (1991), who examined the shallow-marine, Upper Vendian–Lower Cambrian subsurface sequence of the Lublin Slope, East European Platform (EEP). Forty-five form-species were recognized, including descriptions of three new form-genera. They represent six microfossil assemblages, two and four of which were late Edicarians (former Vendian) and early Cambrian, respectively. The assemblages were considered to be evolutionarily controlled because their different compositions were interpreted as independent of paleoenvironments and facies changes. Thus, the Precambrian–Cambrian boundary in the Lublin Slope was established. The Precambrian–Cambrian boundary in Poland was also correlated with acritarch evidence in sequences on the East European, Baltoscandian, Siberian, South China, and Avalon platforms and compared with the earliest trilobite zone on the Baltoscandian Platform and the East European Baltoscandian. This study mainly used taxonomy and referred to, among others, the work of Brück and Vanguestaine (2004) on acritarchs from Ireland.
Because acritarchs showed a cosmopolitan occurrence, they could be used for inter-regional correlations. Additionally, in many Cambrian basins, acritarch biozones are closely correlated with faunal biozones (Jachowicz-Zdanowska, 2013, with references). Very detailed and extended work on acritarchs in biostratigraphic aspects was performed by Jachowicz-Zdanowska (2013). Her material was collected from 22 boreholes in the Upper Silesian Block and from three boreholes in the Moravia region (Czech Republic). Her study was the most comprehensive palynological analysis of the Polish Cambrian, covering almost all lithostratigraphic units, except for a few palynological barren intervals. Nine successive acritarch assemblage zones were determined based on the vertical distribution of the microfossils. The obtained data were used to construct a model of the geological structure of the Precambrian and Paleozoic formations of the Outer Carpathians and the Carpathian Foredeep in south-eastern Poland (Buła & Hadryn, 2008). This type of investigation has been performed worldwide, for example, in Norway (Vidal & Nystuen, 1990), Sweden (Hagenfeldt & Bjerkéus, 1991), and China (Yin et al., 2009).
Another study examined sedimentary successions in three boreholes in Upper Silesia (Sosnowiec IG-1, Goczałkowice IG-1, and Potrójna IG-1). On the basis of the acritarch assemblages, the Lower, Middle, and Upper Cambrian series were recognized, as well as zonation within the Lower and Middle Cambrian (Moczydłowska, 1998). The observed biodiversity trends in these assemblages were interpreted to be caused by regional conditions. Some major bio-events were defined regarding the global phytoplankton diversity during the Cambrian: (i) major radiations occurred in the Early Cambrian and the Late Cambrian; (ii) major turnovers of phytoplankton occurred in the Middle Cambrian and the Late Cambrian; and (iii) one, significant two-stage extinction occurred at the Early-MiddIe Cambrian transition and one unsignificant extinction in the Middle Cambrian. The frequency of geo- and bio-events during the Cambrian, occurring in short intervals of a few million years, indicates the environmental effect on many evolutionary changes. In this work, the taxonomy and zonation aspects were also used, among others by Brück and Vanguestaine (2004).
Acritarch biochronology has provided evidence for the tectonic history of the Upper Silesia terrane (Moczydłowska, 1997, 1998). All Cambrian series and several biozones within a 580 m thick Cambrian succession were recognized in Upper Silesia (southern Poland). The succession also contains rare Lower Cambrian trilobites from the Acado-Baltic faunal province. The distribution of trilobites and acritarchs, with regard to the tectonostratigraphic relationships to adjacent areas, confirmed that the Upper Silesia terrane was originally a distal segment of East Avalonia (part of the Paleozoic microcontinent of volcanic origin on the northern margin of Gondwana, Southern Hemisphere). It rifted off the Avalonian terrane and drifted to its present position as a microcontinent.
The sensitivity of acritarchs to the changes caused by crises was used to analyze the event during the Ordivician/Silurian boundary in the Holy Cross Mountains (Masiak et al., 2003, 2020). The changes in acritarch assemblages during the Ordivician/Silurian confirmed a crisis event. Frequency and diversity of acritarchs temporarily decreased in the Late Ordovician, and then increased at the beginning of the Silurian. A frequency peak coincided with a considerable increase in chitinozoa abundance, which corresponded with the occurrence of bioturbation, brachiopods, and conodonts.
A notable environmental study was conducted by Filipiak (2009), based on a comparison of marine microflora composition in Belgium and Poland (Holy Cross Mountains). A rich phytoplankton assemblage and low-diversity miospore microflora were described from the Upper Devonian deposits of Kowala Quarry. This palynological investigation confirmed the existence of abundant, well-preserved phytoplankton assemblages, consisting mainly of small acritarchs and prasinophytes. Based on the appearance of the acritarch Puteoscortum williereae, this assemblage was restricted to the late triangularis–crepida conodont zones and corresponds to the Puteoscortum williereae zone. This research established the stratigraphic value of P. williereae as a key species for dating the Lower Famennian of Central Europe. After comparing the taxonomical composition of the Holy Cross Mountains and Belgian palynofloras, the detected differences were interpreted as reflecting environmental differences between offshore and more proximal environments, respectively.
Extraordinary Studies on Acritarchs
In addition to routine taxonomical or palynostratigraphical works, sometimes an exceptionally well preserved material allows for extraordinary studies. A series of important, original, and notable observations were made on acritarchs, showing various methodologies and problems within this group of organisms. Contrary to the usual palynological studies, the majority of which are routine and performed worldwide, some studies are unique in their conception and preserved material. Kaźmierczak and Kremer (2002) suggested that not every find of microscopic carbonaceous structures in ancient rocks is a crucial track of the earliest life on Earth (Kaźmierczak & Kremer, 2002). They revealed that changes in temperature of microbial remains embedded in a mineral matrix may influence their original morphology, resulting in structures believed to be Earth’s oldest fossils.
The starting point of this research was an investigation of structures present in silicified (chertified) cyanobacterial mats from the Bardo Mountains (Żdanów locality, southwestern Poland) of the Early Silurian age (approximately 440 my BP). Observation of post-mortem degradation processes in modern coccoid cyanobacteria mats, showed that after burial, and biodegradation the remaining polysaccharide envelopes of cells and cell clusters were often preserved as a cobweb-like carbonaceous material (Figure 2D–F). Other aspects of microfossils have been also studied. In 2009, authors (Kaźmierczak & Kremer, 2009 with references) shared their discovery of internal bodies in problematic Silurian and Devonian organic-walled microfossils classified traditionally as polygonomorph, acanthomorph, sphaeromorph, and herkomorph acritarchs (Figure 3A,B). The authors concluded that these bodies are comparable to the reproductive structures of modern unicellular green algae (Chlorococcales). This finding suggests that some acritarchs may be remains of asexually reproducing (sporulating) vegetative cells of chlorococcalean algae, and confirms earlier suggestions, based on ultrastructural and biomarker studies, regarding the relationship between some acritarchs and green algae. These results contradict the common hypothesis that they could be cysts of dinoflagellates or Prasinophyta phycomas. Discovery of the asexual reproduction mode of representatives of the main acritarch divisions opens new perspectives in biological studies of these microfossils and is of high evolutionary importance. Consequently, the taxonomy of acritarchs may be simplified if different forms (shapes, sculptures) are not considered different taxa. The authors also highlighted the disproportions between the abundance and diversity of “acritarch” green microalgae in the early Paleozoic seas and the scarcity of unicellular chlorophytes in modern marine environments. This phenomenon may be explained by changes in marine phytoplankton composition due to the changing biogeochemical components of the environment. However, this question remains partly unanswered and is a direction for further research.
Another observation was provided on uniquely preserved Late Devonian calcispheres from the core of the deep borehole Sosnowiec IG-1 (Upper Silesia), which were interpreted as acritarchs that underwent early post-mortem calcification (Kaźmierczak & Kremer, 2005). This statement is based on remnants of organic walls, characteristic of various acanthomorphic acritarchs, preserved in calcispheres (Figure 3C,D). They play a role as palaeoenvironmental indicators. The abundance of Devonian early post-mortem calcified acritarchs co-occurring with similarly calcified volvocacean green algae showing variously developed calcareous coatings and linings indicates that their life and early diagenetic (shallow burial) environments were highly oversaturated with calcium carbonate.
Figure 2
(A,B) Early Silurian (Llandoverian) herkomorph acritarchs. (A) Cymatiosphaera sp. and (B) Dictyotidium sp., Łupianka Hill near Żdanów scanning electron microscopy (SEM) images (by B. Kremer). (C) Gorgonisphaerdium ohioense, characteristic Late Devonian acritarch, Kowala quarry, Holy Cross Mountains. SEM image (by P. Filipiak). (D) Light micrographs of modern mat of benthic coccoid cyanobacteria (Sulejów Dam, central Poland). The mat is composed of globular colonies enclosed in thick envelopes (arrow) (photo J. Kaźmierczak). (E) Thermally altered colony of Silurian coccoid cyanobacteria (Żdanów, the Bardo Mountains), originally composed of minute cells (photo J. Kaźmierczak). (F) The quasi-regular, segmented, blackish structures of thermal origin are strikingly similar to the purported Archaean Apex Chert microfossils (photo J. Kaźmierczak).
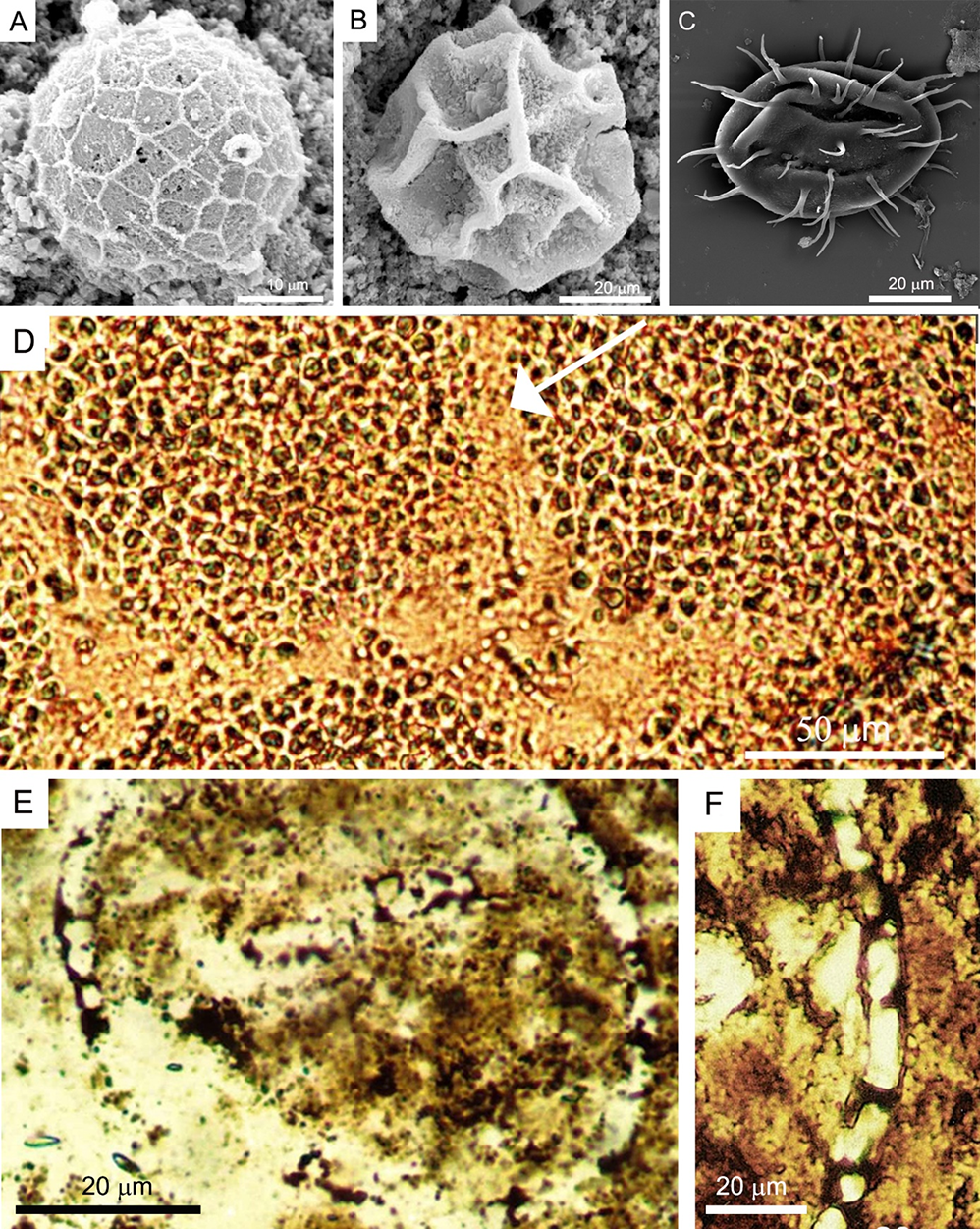
. Other Palynomorphs
Palynostratigraphy, Heterospory, and Seed-Megaspores
In the past 100 years, studies on megaspores and miospores have provided relevant tools for determining and correlating stratigraphic zonation (also with some stratotype profiles) in Poland and Europe (Figure 4A–C). Notably, data on climatic and floristic events during the period boundaries are from Devonian/Carboniferous, Carboniferous/Permian, and Permian/Triassic (e.g., Karczewska & Dybova-Jachowicz, 2001; Kmiecik, 2001; Turnau et al., 2003).
During the first half of the twentieth century, intensive taxonomic studies on Carboniferous megaspores originating from the Upper Silesian Coal Basin were initiated by Zerndt, who first created a megaspore collection in Poland, described 50 taxa (32 new), and classified them into five groups, namely, Laevigati, Apiculati, Zonales, Lagenicula, and Pollen (Zerndt, 1930, 1931, 1932, 1934, 1937, 1940). Studies on megaspores and miospores, and using them for dating and correlations of coal bearing strata, were followed by those by Bocheński, Brzozowska, Dybová, Jachowicz, Karczewska, Kmiecik, Knafel, Krawczyńska-Grocholska, and Żołdani (for details and references see Florjan, 2012; Karczewska & Dybová-Jachowicz, 2001). Microspores were investigated, among others, by Dybová and Jachowicz (e.g., Dybová & Jachowicz, 1957a), also in the context of palynostratigraphy (e.g., Dybová & Jachowicz, 1957b, 1958). For a detailed review, see Dybová-Jachowicz and Jachowicz (1987) and Pajchlowa and Wagner (2001).
Using megaspores in stratigraphic investigations concerning the Polish Devonian was initiated in the second half of the twentieth century by Fuglewicz and Prejbisz (1981), who first described and illustrated this type of fossil in Devonian strata (for details and references see Karczewska & Dybová-Jachowicz, 2001).
The evolution of the heterospory phenomenon is one of the crucial achievements in early land plant evolution, which improves their reproductive strategies. Polish materials from the Miastko-1 borehole provide relevant data documenting this evolutionary event. Turnau and Karczewska (1987) studied some Middle Devonian dispersed spore species whose size range was close to 200 µm (a diameter generally accepted for megaspore identification). Spores of similar morphology have been demonstrated to occur in the Middle Devonian, in two distinct size classes (documented in Biharisporites capillatus, Coronispora variabilis, Grandispora ciliata, and Apiculiretusispora gulata). Although they are of dispersed character, Turnau and Karczewska (1987) suggested that these spores represent micro- and megaspores of the same plants in the process of differentiation from homospory to heterospory, a phenomenon discovered and studied by Petitt (1965) and Chaloner (1967). Notably, these data imply that the small spores were relatively large at the beginning of the heterospory phenomenon evolution. The literature has suggested that in some Devonian plants, all spores increased in size, presumably before spore size differentiation and, probably, sex determination. Only in the later stages of heterospory development did different selective pressures acting on the microspores decrease their size. According to the authors, the reason for the increase in size of the isospores was to improve the nutritional conditions for the developing gametophyte. Thus, they hypothesized that in the course of heterospory development, endospory preceded size differentiation and, presumably, sex determination. They proposed the following pattern for heterospory development in progymnosperms: from exosporous-homospory to endosporous-heterospory. They also suggested that megaspores were produced by progymnosperms and were probably in the same sporangia as microspores, however, could not prove by adequate records (Turnau & Karczewska, 1987).
The appearance of seed-megaspores in fossil records indicates that seed plants began to evolve. Seed-megaspore Granditetraspora zharkovae was proposed by Arkhangelskaya and Turnau (2003) based on dispersed megaspore tetrads consisting of one large (presumably fertile) and three small (presumably aborted) spores from Middle Devonian deposits in European Russia. These are probably the oldest recorded seed-megaspores and show progymnosperm affinity. Arkhangelskaya and Turnau (2003) also implied that the parent plants of these seed-megaspores arose from ancestors that displayed only low-grade heterospory. Dispersed megaspore tetrads, G. zharkovae Arkhangelskaya, and Turnau, were also found in the Middle Devonian deposits in Western Pomerania, Poland (Turnau & Prejbisz, 2006). Based on well-preserved Polish material, diagnoses of the fossil genus Granditetraspora and the species G. zharkovae were emended. Turnau and Prejbisz (2006) discussed some biological and paleoecological aspects connected to seed-megaspore origin because these types of megaspores are relatively large microfossils; thus, they have low fossilization potential outside their areas of origin. That they have been recorded from several localities separated by hundreds of kilometers suggests, according to Turnau and Prejbisz (2006), that the seed plant precursors must have been a common component of the Middle Devonian vegetation and that the earliest stages of seed plant evolution occurred prior to this period. They also concluded that the occurrence of relatively large, nutrient-rich reproductive structures, which might have been dispersed while still enclosed in a sporangium, is an advantage for plants that grew in a hot, periodically arid, Middle Devonian climate.
The subjects of another detailed investigation from the same locality and age were three dispersed megaspore taxa (Turnau et al., 2009). Using light microscopy, scanning electron microscopy (SEM), and transmission electron microscopy for anatomical details of megaspores, the researchers concluded that the Middle Devonian anisodiametric tetrads G. zharkovae might be produced by a progymnosperm. The unknown parent plant of G. zharkovae developed monomegaspory, a distinctive feature of seed plants, and probably confirmed the presence of a gymnosperm ancestor in the Middle Devonian. Such studies are of significant importance for the taxonomy and tracking of evolutionary trends in the development of early land vascular plants.
Studies on Ultrastructure of Megaspore Membranes
The development of techniques over the last 100 years has enabled research on the anatomy of some Middle Devonian dispersed megaspore walls (e.g., Turnau et al., 2009; Zavialova & Turnau, 2012). Specimens originated from the same core samples from the Miastko 1 borehole in Western Pomerania. The samples were first photographed using SEM (after the chemical treatment usually used for SEM preparation), embedded in resin, and cut perpendicular to the megaspore equatorial plane.
Comprehensive investigations using light, scanning, and transmission electron microscopy supplemented the information on the morphology and structure of megaspores and provided data on spore wall ultrastructure of four megaspore species, Corystisporites acutispinosus, Coronispora variabilis, Grandispora ciliata (Figure 4D,E), and Pomeranisporites subtriangularis. Details of their wall structure enabled the formulation of the emended specific diagnoses, as well as suggestions for the systematic affiliation of studied megaspores with lycopsids, putative lycopsids, and some enigmatic Devonian plants.
Spores and Pollen Grains, Palaeofloristic, and Palaeoenvironmental Studies
The composition of spore assemblages in sediments depends on many factors, for example, the distance between the sedimentary basin and the spore-producing mother plant and transport, which is different in the case of large megaspores (shorter distances) and much smaller miospores (found only in some types of environments, mostly terrestrial and near-shore marine). Taxonomic determination (resulting in the diversity of assemblages) depends on spore preservation, but studies of spore successions based on numerous samples from continuous sections may provide crucial information on vegetation, its evolution, paleoecology, and dynamics. Dispersed spores can offer a more complete representation of contemporaneous vegetation than rarer plant macrofossils; however, the botanical affinities of spores are often undeterminable. Observations of changes in vegetation caused by environmental changes are, apart from stratigraphy, one of the most commonly examined subjects of palynological studies and help to understand plant–environment interactions.
The Thermal Alteration Index was used by Stempień-Sałek (2011) in a paleoenvironmental study of Upper Ordovician sedimentary basins of three ancient units: the Avalonia, Baltica, and Lesser Poland blocks. The basis of this method is that transformations in the coalification of organic matter and the degree of its thermal maturity indicate the paleotemperature to which sediment was heated. This method leads to a comparison of the color of fossil palynomorphs ranging from bright yellow to dark yellow, brown, and dark gray to black, with a standardized color scale. The palynomorph colors were related to the temperature gained by the sediment, which allowed the reconstruction of the thermal history of the basins. All the examined units participate in present-day structures of the geological units of northern and central Poland (see Stempień-Sałek, 2011, for references).
Middle Devonian spore successions from northwestern and southeastern Poland, studied by Turnau (2014), have resulted in the reconstruction of floristic dynamics caused by environmental changes. A rapid reduction in spore taxonomic diversity was observed close to the Middle/Late Givetian boundary. A similar decline in diversity was reported in other localities, such as European Russia, Belarus, northern France, and Scotland (see Turnau, 2014, for references). According to megaspore data obtained by Turnau (2014) from Western Pomerania, the Middle Devonian heterosporous plant assemblages were represented by lycopsids, trimerophyte-progymnosperm intermediates, and possible archaeopteridaleans. Homosporous plants (or those with incipient heterospory) include: lycopsids, aneurophytes, zygopterid ferns, cladoxylopsids or trimerophyte-cladoxylalean intermediates, and rhyniophytes, zosterophylls, or trimerophytes. A notable event in the Middle Devonian was the appearance of archaeopterids accompanied by a high diversity of lycopsids and filicopsids. During the Middle Devonian, changes in the frequency of some aneurophytes were observed, and at that time, some advanced progymnosperm taxa appeared and became an important element of the vegetation. These changes were caused by the initial sea-level rise of the Taghanic Crisis, which caused a substantial loss of habitats for some aneurophytes. Repeated shoreline shifts have strongly modified the ecosystem and accelerated the spread of highly competitive archaeopterids. These shifts were especially extensive in cratonic, low-relief areas in eastern Laurussia. Climate aridity caused the disappearance of many plants and was unfavorable for aneurophytes (Turnau, 2014, with references).
Environmental conditions can also be determined from the structure of miospores. Filipiak and Racki (2010) observed enrichment in abnormal spore morphotypes during a terrestrial flora turnover close to the Devonian/Carboniferous boundary in Kowala Quarry (the Holy Cross Mountains). Most Vallatisporites spp. showed an anomalous overall shape and ornamentation (Figure 4F,G). Similarly, altered miospores have recently been found in Sudetes at similar time intervals (Dzikowiec Quarry; Filipiak in Matyja et al., 2021). Such anomalies usually result from environmental perturbations, such as intense volcanism. Other major events that occurred in the Late Devonian of the Holy Cross Mountains were also proven by Filipiak based on palynological research and supported by geochemical data (Kellwasser event; Filipiak, 2002).
Figure 3
(A) Cymatiosphaera, early Silurian (Llandoverian) herkomorph acritarch, scanning electron microscopy (SEM) image (by B. Kremer). (B) Cross section of Cymatiosphaera-like specimen from same sample, enclosing spherical aplanospore-like structures comparable with spores of modern unicellular green algae, light microscope image (by J. Kaźmierczak). (C) Acritarch Dorsennidium patulum (SEM image), Famennian Chagrin Shale, Ohio (from Wicander, 1974, courtesy of R. Wicander) as forerunner for calcispheric structure shown in (D). Thin-section photomicrographs of Late Devonian calcispheres from Sosnowiec IG-1 borehole (photo J. Kaźmierczak).
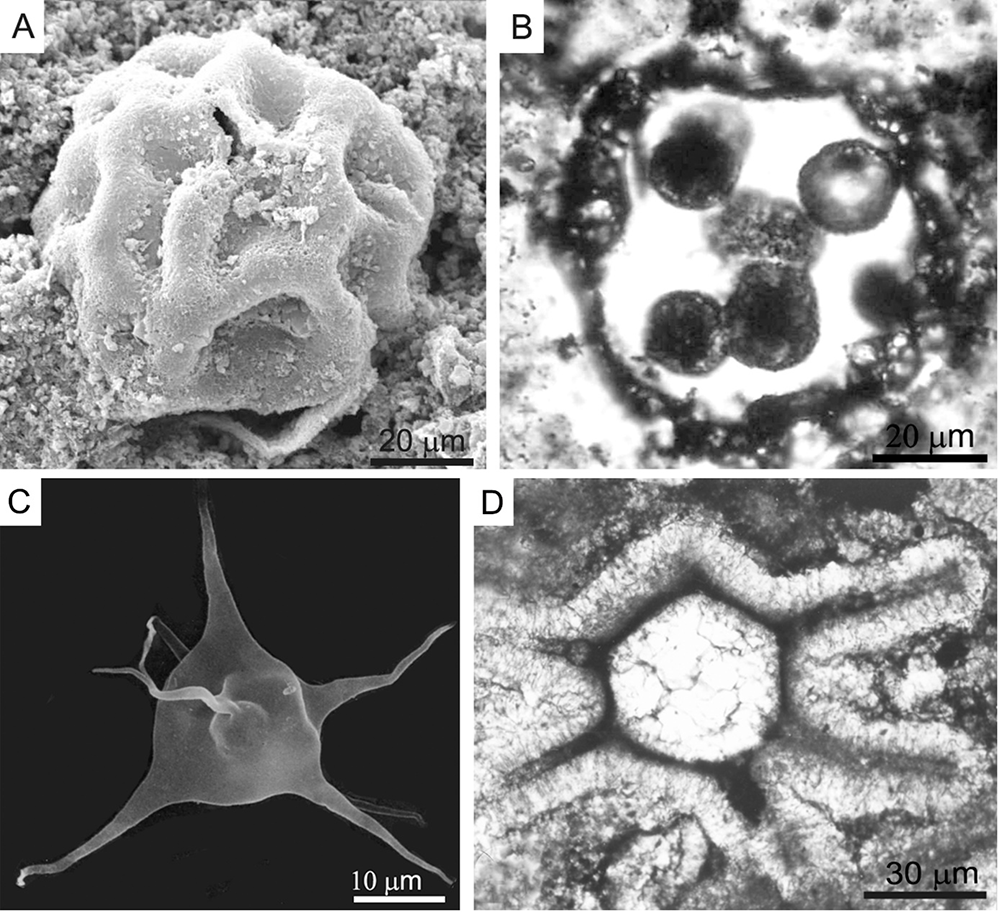
Figure 4
(A) Emphanisporites annulatus; index miospore for the annulatu-sextanti Zone (Lower Devonian), Bukow Góra quarry, Holy Cross Mountains (photo P. Filipiak). (B) Diducites versabilis, important miospore for the late Famenian palynostratigraphy, Kowala quarry, Holy Cross Mountains (photo P. Filipiak). (C) Retispora lepidophyta, the most important cosmopolitan species for dating the D/C boundary, late Famennian, Kowala quarry, Holy Cross Mountains (photo P. Filipiak). (D) Grandispora ciliata, Devonian (photo E. Turnau). (E) The same species, fragment of proximal wall showing the inner body and inner part of the outer envelope (photo N. Zavialova). (F,G) Two mutated miospores Vallatisporites? from the Devonian/Carboniferous boundary in the Kowala quarry, Holy Cross Mountains (photo P. Filipiak).
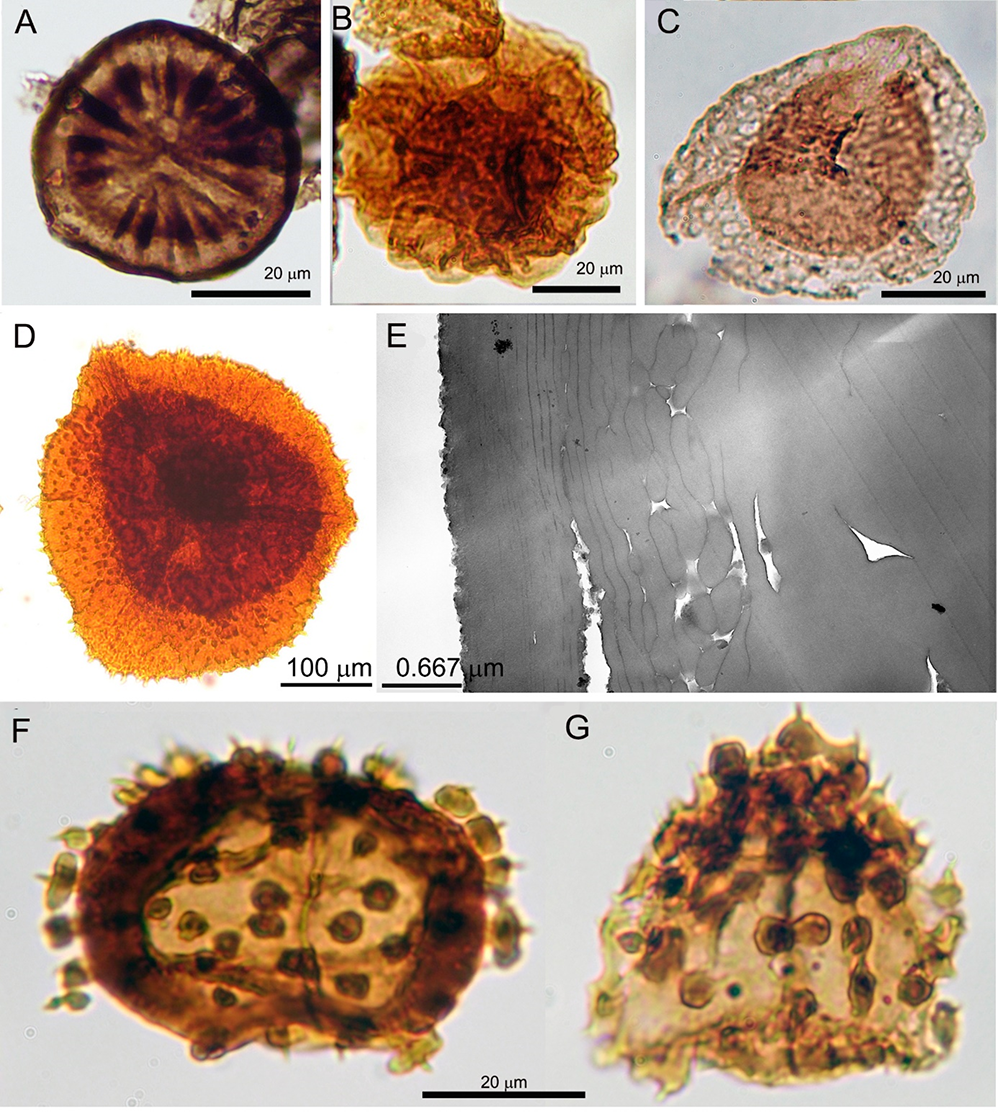
The Lower Devonian strata from the Upper Silesian Block, Małopolska Block, and the Łysogóry Block were investigated by Filipiak (2011). The samples originated from 11 boreholes, a trench, and a quarry representing the Upper Pragian to Eifelian interval. In many cases, the investigated samples were obtained from transitional facies where faunal information was insufficient and only palynostratigraphical data were reliable. Palynofacies observations suggest terrestrial to shallow-marine conditions during the transitional period between the Early and Mid-Devonian periods. The upper Pragian to Emsian sedimentary rocks from the Upper Silesian Block and Lesser Poland Block were deposited under marginal marine or alluvial or both conditions. Full marine conditions prevailed only in the upper Emsian in the Łysogóry block. During the Early Eifelian, similar marine conditions were established across the investigated region.
Lower Devonian rocks have also been investigated in Trojanowice 2 boreholes (Filipiak, 2014). Based on the palynostratigraphy, two standard miospore zones were recognized from the two stages of the latest Early Devonian and the earliest Middle Devonian. Both zones showed differences that were interpreted to be caused by the paleoenvironment. Palynofacies from the lower part of the section indicated marginal marine paleoenvironmental conditions, and those from the upper part indicated deeper marine conditions further from the shoreline.
A mixture of plant palynomorphs and small fragments of arthropod cuticles has been reported from the Lower Devonian clastic deposits of the Upper Silesian and Lesser Poland blocks in southern Poland (Filipiak & Zatoń, 2011). The plant material comprises higher plant cuticles and enigmatic remains (nematophytes), classified as Nematothallus, Cosmochlaina, and tubular banded tubes. The presence of such mixed assemblages indicates that marginal-marine and/or alluvial environments are spreading in the southern Poland margin of the Old Red Continent.
Analysis of the palynofacies of the Frasnian/Famennian boundary (Upper Devonian) (Kowala Quarry and Jagów-Płucki trench) provided an opportunity to reconstruct sedimentary conditions and palynofloral history over the extinction boundary interval (Filipiak, 2002). Based on the taxonomic impoverishment of acritarch and miospore assemblages and a low proportion of land-derived material, offshore sedimentation in a mid-shelf environment was confirmed, and increasing miospores and land-plant tissues suggested eustatic sea-level fall during the earliest Famennian. The increased productivity of phytoplankton (leiospheres, the most commonly observed palynomorphs) and amorphous organic matter near the Frasnian/Famennian boundary might be caused by eutrophication and/or disturbances in a marine trophic web, which could stimulate a rapid increase in primary production and subsequent expansion of hypoxic conditions. Based on data from the literature, including palynological research in Poland, there was no basic change in marine palynofloras and a long-lasting decline in primary production during the end-Frasnian biotic crisis, in contrast with events during Wenlockian (Silurian) or Viséan (Devonian) biotic events.
Based on a palynological study of the uppermost Famennian section (the latest Devonian) from the Kowala Quarry, two miospore zones were identified: Retispora lepidophyta–Apiculiretusispora verrucosa and Retispora lepidophyta–Verrucosisporites nitidus (Marynowski & Filipiak, 2007). The black shale within the upper part of the section was identified as equivalent to the globally known Hangenberg Black Shale, which resulted from global sea-level rise. The Kowala Black Shale, dated as the Retispora lepidophyta–Apiculiretusispora verrucosa zone, might represent the transgressive phase of the Upper Devonian Sea. Because this phase is not reflected in the global transgressive/regressive curve, it might be the result of regional tectonic activity during the Famennian period. Sea-level fluctuations were common in the uppermost Fammenian, suggesting that the transgressive–regressive cycle during the Hangenberg event was rapid. Increased phytoplankton productivity in the upper part of the water column was recorded in black shales. The increased concentration of marine particles from sample K4 (from Kowala) of the tuffite layer is explained by the “sea snow effect” of volcanic ash, in which phytoplankton rapidly accumulate due to dense mineral sedimentation. Rapid water column transport and burial facilitated the accumulation of large amounts of organic matter, even under oxic conditions.
Another significant result of this study was obtained from the analysis of the first documented fossil charcoal from the Upper Famennian deposits of the Holy Cross Mountains. Such organic particles provide evidence of wildfires. The rounded edges, rather small in size, and general no internal cellular structures suggest an allochthonous origin and transport over a relatively long distance.
Palynological investigations, combined with geochemistry, of the Lower to Middle Frasnian (Middle Devonian) reef-to-basin succession at the Kowala Quarry (Marynowski et al., 2008) have demonstrated the variable character of organic matter deposited under different oxidative–reductive conditions. The palynostratigraphical study allowed for the recognition of two miospore zones: Geminospora semilucensa–Perotrilites donensis and Archaeoperisaccus ovalis–Verrucosisporites grumosus. Relatively small quantitative and qualitative changes in organic particles (miospores, tracheids, prasinophytes, and acritarchs) are interpreted primarily in terms of sea-level fluctuations and enhanced productivity.
The boundaries of geological periods are usually associated with significant environmental changes and, consequently, changes in the composition of floras. Such an event was detected in shale layers from the Intrasudetic Basin (northeastern periphery of the Bohemian Massif, southwestern Poland) containing the latest Carboniferous to Early Permian palynological assemblage (Jerzykiewicz, 1987). A decrease in the relative abundance of spores was observed, especially in the monolete group, and the relative abundance of trilete spores remained consistently low throughout the sequence. Additionally, the relative abundance of pollen grains increased (bisaccate pollen increased from 2% to 41%). The pollen assemblage changed from monolete spores and monosaccate pollen dominated through an intermediate stage with the domination of monosaccate pollen to mixed monosaccate and disaccate pollen flora. These gradual changes in palynological assemblages at the Carboniferous/Permian boundary were interpreted in terms of gradual climatic changes. The latest Carboniferous assemblage was relatively rich in spores, indicating lowland swamp flora. The gradual drying of Permian conditions is indicated by an increased abundance of wind-disseminated pollen grains in the assemblages of the earliest Permian. The palynomorph-based floral succession of the Intrasudetic Basin corresponds well with a generally accepted statement on the progression of climate aridity from the Late Carboniferous to Early Permian.
The Late Permian (Zechstein) microflora from southern Poland provided data on the paleoclimate and paleogeography of the Polish Basin (Fijałkowska-Mader, 1997). The author recognized seven spore-pollen assemblages from four regions (the North-Sudetic Through, Fore-Sudetic Monocline, Holy Cross Mountains, and Nida Trough), which are correlated with other European (German and Alpine) basins. The microflora was dominated by xerophytic plants, mainly conifer pollen grains, namely, near rare hygrophytic forms such as lycopsids, ferns, or cycads, indicating a dry and warm climate during Late Permian. The depositional environment was interpreted as shallow water (because of the presence of acritarchs). Arid conditions were interrupted locally by humid periods caused by transgressions by the end of the Zechstein sedimentation. The microflora precisely indicated all changes, which led to the conclusion that during the Late Permian, the sebkha environment was dominated by periods of deposition in the deeper conditions of a shallow lagoon.
The Late Permian crisis caused by strong environmental stress was detected by Fijałkowska-Mader (2012, 2020) based on abnormal morphological forms of bisaccate pollen grains Lueckisporites virkkiae and Jugasporites delasaucei, which are typical elements of Permian microfloristic assemblages. The mutation was visible in the number and shape of sacci and the thickness and structure of the exine of the central body. The aberrated pollen grains became trisaccate or sackless, the sack could be fully developed or reduced, the exine of sacs could be abnormally thick or thin, and the exine of the body thickened. The percentage of abnormal pollen grains increased toward the end of the Permian. Mutations were probably affected by increased UV radiation caused by volcanic activity in the territory of today’s Siberia and China.
Palynomorphs in Biostratigraphy
Many palynostratigraphic studies have been conducted in Poland. The oldest strata studied in Poland based on palynomorphs were Upper Ordovician in age. The precursor of this research on a European scale was conducted by Jagielska (1962), who provided a taxonomical background for further analyses. The series of analyses in five large geological units of northern and central Poland (epi-Caledonian Platform, EEP, Małopolska Block and Holy Cross Mts–Łysogóry Fold Zone, and Kielce Fold Zone) were made by Stempień-Sałek (2011). She determined the Ordovician/Silurian boundary in the studied areas and proved that two recognized taxonomically different palynomorph assemblages represented taxa characteristics of the border of both periods and could be used as age indicators.
Turnau and Narkiewicz (2011) studied the boundary between the Middle and Upper Devonian periods in Western Pomerania. The authors defined biohorizons based on the first appearance of spore species and intercalibrated this spore zonation scheme with the “standard” and alternative conodont zonations. Such calibrated spore-based zones can then be determined independently on conodonts that are not as widespread as spores.
Turnau (1985, 1986, 2011) developed the entire stratigraphy of the Polish Devonian period.
Miospores have also been used in biostratigraphy, such as those from the boundary of the Middle and Upper Devonian in Western Pomerania and associated with conodonts (boreholes in the Lublin area) studied by Turnau and Narkiewicz (2011). The authors defined biohorizons based on the first appearance of spore species and intercalibrated this spore zonation scheme with the “standard” and alternative conodont zonations. Thus, spore-based zones can be determined independently on conodonts that are not as widespread as spores.
Using miospore analysis, Pluta and Górecka-Nowak (2018) changed the age of the Pogorzała Formation in the Świebodzice Unit from the Upper Devonian (previously determined based on marine fossils) to the late Lower Carboniferous. In other studies (Górecka-Nowak et al., 2021; Górecka-Nowak & Majewska, 2002) on the age of the Carboniferous stages based on palynology, the Szczawno Formation (Szczawno) was revised, and correlated with the corresponding strata of the British chronostratigraphy.
Carboniferous miospores of Silesian productive strata were examined by Jachowicz in the 1960s of twentieth century and subsequently by Górecka, Karczewska, Kmiecik, Krawczyńska-Grocholska, Kruszewska and Turnau (for details and references see Kmiecik, 2001; Turnau & Filipiak, 2003). The palaeostratigraphic and palynofacial aspects of the Devonian/Carboniferous boundary in the Holy Cross Mountains were studied by Filipiak (2004, 2005). Data from the western part of the mountains provided new palynostratigraphic information. The author determined an almost complete palynological zonation covering the latest stage of the Devonian and the earliest stage of the Carboniferous.
Kruszewska (1963) and Górecka (1969) have conducted palynological and palynostratigraphical studies concerning the Carboniferous. Studies on the complex stratigraphy of the Carboniferous succession were conducted by Górecka-Nowak (2008, 2010) based on rocks from nine wells in Greater Poland. The timing of Carboniferous deposits at these sites was reinterpreted, and deformation in strata was discussed based on miospore assemblages.
The Carboniferous–Permian transition has been studied in detail by Górecka and Parka (1980) and Krawczyńska-Grocholska and Grocholski (1976) (for more references see A. Jachowicz & Dybová-Jachowicz, 1987).
Kmiecik (1987) and Kmiecik and Knafel (1988) have studied Carboniferous deposits at the margin of the Polish part of the EEP.
. Summary
This review comprises select achievements of Polish palynology concerning studies on Paleozoic acritarchs, spores, megaspores, and pollen grains over the last 100 years. Although the majority of the investigations were by Polish authors and on Polish materials, Polish palynologists cooperate widely with many countries, for example, in correlations of different geological units or terranes. In Poland, palynology as a branch of science developed in the twentieth century and is a milestone in Polish paleobotany.