. Introduction
Plants, including maize (Zea mays L.), are subjected to a variety of biotic and abiotic stresses during growth and development. Among these stresses, drought is one of the major abiotic factors that limit crop production. In the past decade, global losses in crop production due to drought have totaled about 30 billion dollars (Gupta et al., 2020). Water stress is likely the most important environmental factor that adversely affects plant growth and development. The effects of drought on plants have been studied for a long time, and some adaptive mechanisms to cope with water deficiency have been examined on biochemical and molecular levels (Farooq et al., 2009; Gupta et al., 2020). During ng the past few years, several studies emphasizing the role of drought signals, stress-protectant metabolites, and phytohormones in the model plant Arabidopsis thaliana have been published and reviewed by Takahashi et al. (2020). Despite these advances in the understanding of plant responses to drought at the cellular, physiological, and morphological levels, our knowledge about these mechanisms in crops is still limited.
The role of plasma membrane (PM) H+-ATPases in regulating root growth and development is well-established, involving their facilitation of plant responses to diverse stresses, including nitrogen deficiency, low phosphorus stress, and salinity (Janicka et al., 2018; Młodzińska et al., 2015; Yuan et al., 2017). Previous results have shown that selected isoforms (autoinhibited PM AHA2 and AHA7) are involved in the control of Arabidopsis root elongation and development in response to nitrogen and phosphorus status (Młodzinska-Michta, 2022; Młodzińska et al., 2015). Reduced H+-ATPase activity in response to salt stress has been linked with a reduction of leaf expansion in salt-sensitive maize (Pitann et al., 2009). Since PM H+-ATPase is involved in regulating cell elongation, it was assumed that its activity and gene expression under osmotic stress might also be responsible for the adaptation of the maize root system to drought. We have carried out an investigation of the mechanisms that allow roots to adapt to osmotic stress in two maize varieties: drought-sensitive Abelardo and drought-tolerant Calo. The two varieties of maize were selected based on agronomic data and field experiments conducted by the Syngenta company between 2019 and 2021. The results indicated that the Calo variety exhibits greater resistance to drought stress and is characterized by a high yield potential. Because root growth plays a crucial role in adaptive responses (Chapman et al., 2012; Darwin, 1880), we assumed that acidification of the apoplast and an increase of cell wall extensibility in root tissue would be the key factors responsible for growth promotion, and therefore we looked for changes in PM H+-ATPase activity. Previous studies have revealed the expression profile of four genes for plasma membrane proton pumps (MHA1–MHA4) in the roots of maize seedlings, with the prevalence of the genes MHA3 and MHA4. The exposure of maize seedlings (hybrid Oster) to salt stress (0.1 M NaCl) activated the expression of the MHA4 gene. These results suggest that this isoform may be important for adaptive responses to salinity (Kovalenko & Palladina, 2016). Therefore, to investigate the effects of PEG treatment on the transcription of genes encoding plasma membrane proton pumps, real-time RT-PCR with primers specific for the MHA1, 2, 3, and 4 isoforms was performed.
In maize roots, the components that fine-tune PM H+-ATPase activity remain unclear. Particularly, the differences between the roots of drought-sensitive and drought-tolerant plants are unknown. The enzyme activity is modulated by its regulatory domain in the carboxy-terminal region (the R-domain). The phosphorylation of the penultimate amino acid Thr-947 in the R-domain and the subsequent binding of a 14-3-3 protein is the major common mechanism by which PM H+-ATPases are activated in plant cells (Falhof et al., 2016). The activity of PM H+ATPases is also known to be regulated by lipids, namely lysophospholipids, which are formed during membrane shaping by phospholipases. Lysophosphatidylcholine was the first factor found to activate the plant plasma membrane H+-ATPases (Palmgren & Sommarin, 1989). It was confirmed that lysophospholipids alter the PM H+-ATPase activity by changing the conformation of the R-domain (Morales-Cedillo et al., 2015). The role of other lipid components of cellular membranes, in particular polyisoprenoid alcohols (i.e., polyprenols and dolichols), is also being studied. A possible role of polyisoprenoids, as modulators of the physicochemical properties of membranes, in plant defense against biotic and abiotic stresses has been proposed (Surmacz & Swiezewska, 2011). However, the reorganization of isoprenoid metabolism after osmotic stress in maize has not been studied yet. Therefore, we have investigated the changes in polyisoprenoid content in the plasma membrane during osmotic stress in the roots of the Abelardo and Calo maize varieties.
It has been widely reported that the accumulation of osmolytes, such as proline (Pro), in response to drought facilitates water uptake and protects cells against ROS accumulation (Ashraf & Foolad, 2007). Therefore, in the present study, the levels of proline were analyzed in control and drought-stressed roots. Furthermore, for the first time, the Normalized Difference Vegetation Index (NDVI) – an agricultural measurement tool, was used under laboratory conditions to analyze individual leaves of maize from plants subjected to osmotic stress. This tool serves to estimate drought by analyzing remote-sensing images. A higher value of NDVI refers to healthy vegetation and optimal chlorophyll status, while a low NDVI value indicates the weaker condition of the plants (Drisya et al., 2018). The analyses of these parameters (Pro levels and NDVI) help us to understand the mechanisms that support the adaptation of maize seedlings to drought stress.
. Material and methods
Plant material
The maize grain Abelardo and Calo (FAO 220-230) were provided by the company Syngenta Poland: (https://www.syngenta.pl/uprawy/kukurydza/sy-abelardo, https://www.syngenta.pl/uprawy/kukurydza/sy-calo). Seeds were surface sterilized in ACE (regular bleach) for 5 min, rinsed with distilled water, and germinated in the dark at 28 °C for 48 h. All plants (for root phenotyping, biochemical and genetic analyses) were grown in hydroponic cultivation on Hoagland’s solution (pH = 6.0) (Hoagland & Arnon, 1950) under the following environmental conditions: 16/8 light/dark cycle, 180 µmol−1 m−2 light intensity, and 21 °C. After seven days, the nutrient solution was replaced for 24 hours with Hoagland’s medium supplemented with 20% PEG 6000 (Sigma-Aldrich) while control plants were transferred to regular Hoagland’s medium. Total root length and fresh and dry weights (FW and DW) were measured for eight-day-old plants. Experiments were performed in triplicate; n = 25 seedlings were used for each biological replica.
Isolation of plasma membrane vesicles and protein concentration
Roots of eight-day-old plants (30–50 g) were cut and ground in cold homogenization buffer (25 mM BTP-MES pH = 7.5 containing 330 mM sorbitol, 5 mM EDTA, 5 mM DTT, 0.5 mM PMSF, 0.2% BSA) following a modified two-phase DEXTRAN/PEG system method of Kłobus (1995). The homogenate was filtered and centrifuged for 10 min at 4 °C at 18,000 × g. The supernatant containing microsomal membranes was ultracentrifuged at 100,000 × g for 34 min. The obtained microsomal pellet was re-suspended in 5 mM BTP-MES buffer pH = 7.5, containing 5 mM KCl, 0.1 mM EDTA and 330 mM sorbitol and proceeded to an eight-gram, two-phase 6.2% DEXTRAN 450,000–650,000/ PEG 3,350 partitioning system. PM vesicles were recovered in the polyethylene glycol-rich upper phase and other cellular membranes in the interface and lower phase. The upper phase was washed and used as a source of the plasma membrane by centrifugation at 100,000 × g for 34 min. The PM pellet was re-suspended in re-suspension buffer and immediately used for H+ pumping activity analyses or stored at minus 70 °C for Western-Blot experiments. This method allows for obtaining plasma membrane vesicles with a high purity level and defined right-side orientation.
Protein concentration was determined using a method published earlier (Bradford, 1976) with BSA (Bovine Serum Albumin) as a standard.
Hydrolytic and H+ pumping activity of plasma membrane H+-ATPase
The hydrolytic activity of the vanadate-sensitive H+-ATPase was determined according to the procedure of Gallagher and Leonard (1982). Isolated plasma membrane fraction (35 µg of protein) was incubated in a reaction mixture containing 165 mM TRIS-MES (pH 7.5) with 250 mM KCl, 12.5 mM MgSO4, 0.1 mM NaN3, 0.1 mM Na2MoO4, 50 mM NaNO3, 8 µM Na3VO4 and 0.02% Triton X-100. The ATPase activity was expressed as the difference between the activity measured in the absence and the presence of Na3VO4. The Pi released during the reaction was determined according to Ames (1966) in the presence of 0.2% (w/v) sodium dodecyl sulphate. H+ transport activity across inside-out oriented vesicles was measured spectrophotometrically as a quenching of acridine orange absorbance at 495 nm (A495) according to Kłobus and Buczek (1995). The assay medium consisted of PM vesicles (approx. 50 µg of protein), 25 mM BTP-MES (pH 7.5), 330 mM sorbitol, 50 mM KCl, 0.1% BSA, 10 µM acridine orange and 0.05% Brij 58. Proton transport was initiated by the addition of 3.75 mM Mg-ATP. Passive proton movement through the membrane was determined without Mg-ATP in the reaction medium.
Immunodetection of plasma membrane H+-ATPase protein in roots
Plasma membranes (20 µg of protein) were incubated in 4xSDS Sample Loading Buffer with 8 mM urea at 70 °C for 10 min and separated on 7.5% SDS-polyacrylamide gels (Laemmli, 1970). After electrophoresis (25 mA), proteins were electrotransferred (55 V) to nitrocellulose for 1 h using a BIO-RAD MiniProtean apparatus. Transfer buffer contained 25 mM Tris, 150 mM Gly, and 10% (v/v) methanol. To identify the plasma membrane H+-ATPase, the blots were incubated overnight (8 °C) with polyclonal antibodies against plasma membrane H+-ATPase (AS07 260, Agrisera). The antiserum was diluted 1:1,000. After repeated washing, the nitrocellulose membrane was incubated at room temperature for 1 hour with 1:10,000 diluted secondary antibody anti-rabbit IgG horse radish peroxidase conjugated (AS09 602, Agrisera) and visualized by staining with Western Lightning Plus, Chemiluminescent Substrate (Perkin-Elmer). Blots were scanned with a ChemiDoc Imaging System and analyzed with Image Lab Software, BIO-RAD.
Proline determination
Colorimetric determination of proline content proceeded according to Bates et al. (1973). In brief, fresh roots from eight-day plants (0.5 g) were incubated at 100 °C for 1 hour with a solution of ninhydrin and glacial acetic acid. The toluene-soluble proline was measured at 520 nm. The method was calibrated for each determination with standard proline solutions (the linear detection range of proline was 0.5–2.0 µM).
Measurement of pigments (chlorophylls, carotenoids, and anthocyanins) content in leaves
The photosynthetic pigments were established based on the method of Wellburn (1994). Leaves of PEG-treated and control plants (20–35 mg) were collected, and pigment estimation was conducted according to the procedure described by Tiwari et al. (2021). For the extraction of chlorophylls and carotenoids, maize leaves were kept in 1 ml of 80% acetone in the dark overnight, and for anthocyanin extraction, leaves were homogenized in 1% acidified (HCl) methanol at room temperature. All these procedures were carried out in semi-darkness. The absorbance of the supernatant was observed at 646.8, 663.3 and 470 nm for chlorophylls and total carotenoids as well as at 520 and 650 nm for anthocyanins, respectively.
Normalized Difference Vegetation Index (NDVI) of maize leaves
The Normalized Difference Vegetation Index (NDVI) quantifies vegetation by measuring the difference between near-infrared and red light, thus measuring the state of plant health. Multispectral images of proximity, without georeference, were taken with the RedEdge MX multispectral camera (Micasense Inc., Seattle, Washington, USA) from a height of 120 cm, which allowed for a field resolution of 0.08 cm/pixel. Pictures were taken in 5 channels: blue (475 nm, 32 nm gate), green (560 nm, 27 nm gate), red (668 nm, 16 nm gate), Red Edge (717 nm, 12 nm gate), near-infrared (842 nm, gate, 57 nm). The image resolution for each band was 1280 × 960 pixels (1.2 megapixels), and the images were saved as uncompressed tiff files with 12-bit color depth. For further analysis, the red and NIR channels were used to calculate the NDVI, according to the formula: NDVI = (NIR-RED) / (NIR + RED) (Tucker, 1979; Tucker et al., 1980). The photos were triggered remotely over the Wi-Fi network using a computer under artificial lighting. The obtained images in the RED and NIR bands were imported into Fiji (Schindelin et al., 2012), and then, using the Region of Interest (ROI) tool, the average reflectance of the selected polygons was read. The next stage was the export of data to the R version 3.6.2 program (R Core Team, 2019) for the calculation of the NDVI index and basic statistics.
Real-time PCR analysis of PM H+-ATPase genes transcription
Total RNA was extracted and purified using a commercial Eurx kit (Molecular Biotechnology Products, Gdańsk, Poland) according to the manufacturer’s instructions. Concentrations and purity of RNA preparations were measured spectrophotometrically (NanoDrop ND-1000, Thermo Scientific, USA). Purified RNA samples were used as templates for first-strand cDNA synthesis using the High-Capacity cDNA Reverse Transcription Kit (Applied Biosystems) following the manufacturer’s protocol. Real-time PCR was conducted using a Light Cycler 480 II system (Roche) and the Real-Time 2xPCR Master Mix SYBR kit for PCR amplifications (A&A Biotechnology). The following conditions were applied: 30 s at 95 °C; 40 cycles of 10 s at 95 °C, 10 s 56 °C–58 °C and 12 s at 72 °C, with final melting for 15 s at 65 °C. The gene encoding the ZmTUA5 (alpha-tubulin) protein was used as an internal standard for normalizing the expression of all investigated genes (ZmMHA1–ZmMHA4). The gene-specific primer sequences used are shown in Table S1. The expression levels of all isoforms were normalized to the expression level of ZmTUA5 (α-tubulin). All amplification efficiencies were superior to 2.0. The ratio of relative expression was calculated according to Pfaffl’s model (Pfaffl, 2001). The data are based on three independent replicates.
Lipid extraction and polyisoprenoid profiling in plant material
Plant material from roots or leaves of eight-day-old maize plants was air-dried, homogenized in 20 ml of acetone:hexane mixture (1:1, v/v), supplemented with an internal standard (Prenol-19, 10 µg), and left in the darkness for 48 h with occasional mixing. The obtained extracts were processed and analyzed as described previously (Akhtar et al., 2017). In brief, the extracts were filtered and evaporated under a nitrogen stream. Crude lipid extracts were hydrolyzed, extracted with hexane, separated on silica gel columns, eluted with 10–15% diethyl ether in hexane, transferred to isopropanol, and analyzed by HPLC-UV using a Zorbax XDB-C18 reverse-phase column (Agilent) and detection by absorption at 210 nm. Identification was based on comparison with external standards; quantification was relative to the supplied internal standards. Preparations of the plasma membrane fractions (0.25 ml) were added to 10 ml of chloroform:methanol mixture (1:1, v/v), supplemented with an internal standard (Prenol-19, 3 µg) and left in the darkness for 48 h with occasional mixing. The obtained extracts were filtered, hydrolyzed, and extracted with hexane as described above, and then directly transferred to isopropanol and analyzed by LC-MS using a Waters Acquity UPLC apparatus coupled with a Waters Xevo TQ-MS triple-quadrupole mass spectrometer. Chromatographic separation was performed using a reversed-phase Accucore C30 column (150 mm × 2.1 mm, 2.6 µm, Thermo Fisher Scientific). The mobile phase A consisted of 10 mM ammonium formate with 0.1% formic acid in ACN/MeOH/H2O (40:20:20, v/v/v) and mobile phase B of 10 mM ammonium formate with 0.1% formic acid in IPA/ACN (90:10, v/v). The gradient started with 90% phase A, then ramped to 35% over the next 3 min. Phase B content increased by 5% every 5 min of isocratic elution until reaching 99% at the end of the gradient. The total run time was 47 min. The mass spectrometer operated in selected-reaction monitoring mode (SRM). Data was collected for ammonium adducts in positive electro-spray ionization (ESI) with 2.0 kV capillary voltage and 25.0 V cone voltage. Acquired spectra were processed using TargetLynx XS 4.1 (Waters) software. All polyprenol and dolichol standards used were from the Collection of Polyprenols, IBB PAS.
Statistical analysis
The data were subjected to analysis of variance, and post hoc comparisons were carried out with Anova and Sidak’s multiple comparison tests or with unpaired t-tests with Welch’s correction (as appropriate), with significance set at p < 0.05. Graphpad Prism 6.0 or 9.5 software was used for graph design and statistical calculations. The values are the means and SD of three replicates from three independent experiments.
. Results
Root growth characteristics of maize seedlings under osmotic stress
To analyze phenotypic responses to drought stress, total root length was measured in control and stressed plants (Figure 1A). Total root length was significantly influenced by drought stress in the Abelardo cultivar. Compared to the control conditions, drought caused a decrease in root growth by almost 50% after 24 hours of PEG treatment. The length of Calo roots did not show any visible changes after exposure to PEG compared to control plants. Although a slight (approx. 10–15%) decrease of the fresh (FW) and dry weight (DW) of roots was observed in both Abelardo and Calo plants upon drought stress (compared to control, non-treated plants), these differences were not significant (Figure 2A–B, respectively). There was no remarkable morpho-physiological difference between non-stressed and stressed roots of the Calo cultivar (Figure 1C). On the other hand, symptoms such as leaf tip yellowing and reduction in root growth were observed in drought-sensitive Abelardo cultivar (Figure 1B).
Figure 1
Effect of osmotic stress on root length of corn (Zea mays L.) Abelardo (drought-sensitive) and Calo (drought-tolerant) cultivars (A). Values represent means ± SE of three independent experiments. The asterisk (*) marks significant differences according to Sidak’s posthoc multiple comparison test (two-way ANOVA) at p < 0.05. The morphology of eight-day-old maize seedlings Abelardo (B) and Calo (C) cultivars exposed to drought. Symptoms such as leaf yellowing (red arrows) and root growth reduction were observed in the drought-sensitive Abelardo cultivars (B).
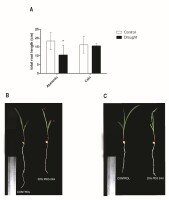
Figure 2
Effect of osmotic stress on root fresh weight (FW) (A), root dry weight (DW) (B), and fresh weight (C) and dry weight (D) of green parts (shoots and leaves) in Abelardo and Calo corn cultivars, respectively. The asterisk (*) depicts significant differences according to Sidak’s posthoc multiple comparison test (ANOVA, two-way) at p < 0.05.
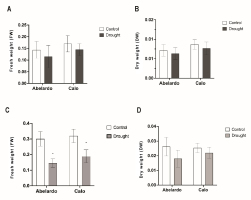
Drought stress restricted not only the elongation of roots but also the fresh weight and production of the dry mass of the above-ground plant parts. Drought (after 24 h) noticeably reduced the fresh weight of green parts in Abelardo and Calo plants (Figure 2C). There were no significant differences in the DW weight of above-ground parts noticed in both Abelardo and Calo cultivars (Figure 2D).
Pigment contents
Chlorophyll content declined under osmotic stress compared to control plants. Although a decrease in chlorophyll A and B was observed after 24 h of PEG treatment in both cultivars, significant differences were noticed only for chlorophyll A (Table S1). Carotenoid content also decreased in PEG-treated plants compared to control.
The content of anthocyanins significantly increased in Abelardo and Calo leaves after 24 h of PEG treatment relative to the control. Moreover, the Calo cultivar accumulated more anthocyanins than Abelardo under conditions of osmotic stress (Table S1).
The NDVI index (Figure S1) also indicates Calo’s better fitness than Abelardo’s in conditions of induced drought.
Proline content in roots
The concentration of proline in roots was greatly affected by osmotic stress, as shown in Figure 3. After PEG treatment, the proline content increased remarkably and reached levels almost 2.5- and 2-fold higher than control levels for Abelardo and Calo roots, respectively. In addition, proline content was higher in the roots of Calo compared to Abelardo under both types of growth conditions (Figure 3).
PM H+-ATPase hydrolytic activity and H+ pumping
To examine the effect of drought on PM H+-ATPase, the hydrolytic activity and proton flux were measured in membrane fractions. The hydrolytic activity of this enzyme (measured as phosphate release) for Abelardo roots treated with PEG was notably lower (about 20%) than for control roots. This suggests that drought stress caused a decrease of PM H+-ATPases activity in the roots of the drought-sensitive Abelardo plants. On the contrary, in the drought-tolerant Calo plants, stimulation of the ATP hydrolytic activity of PM H+-ATPases (about 17%) was observed (Figure 4).
Figure 4
Percentages of H+-ATPase hydrolytic activity of plasma membrane fractions from root samples of maize seedlings (Abelardo and Calo) under control and osmotic stress (drought) conditions. Values represent means ± SE of three independent experiments.
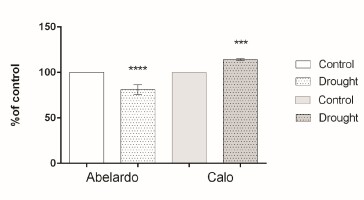
The percentage of ATP-dependent H+ transport and the quenching rate of AO absorbance at 495 nm during 3 min in the in-side oriented plasma membrane vesicles is shown in Figure 5, Figure S2. These results demonstrate that PM vesicles isolated from PEG-treated roots in the Calo variety display a rapid increase (about 40%) in H+ transport compared to control plants. In contrast, PM vesicles purified from stressed roots of Abelardo plants showed a decrease in H+ extrusion by about 20% of the control. Thus, proton transport driven by plasma membrane H+-ATPases in maize roots was significantly affected by osmotic stress, and this observation was consistent with the observed here, and this observation was consistent with the observed changes in ATP hydrolytic activity.
Gene expression levels of MHA1, 2, 3, 4 and immunodetection of plasma membrane H+-ATPases in roots under osmotic stress
To investigate the effects of PEG treatment on the transcription of genes encoding plasma membrane proton pumps, real-time RT-PCR with primers specific for the MHA1, 2, 3, 4 isoforms was performed. In root tissue material, primer-specific amplification was detected for all isoforms (Figure 6A–D). However, MHA1 was expressed at a very low level under the experimental conditions used. The obtained data indicated that the relative transcription of MHA1 was lower in PEG-stressed roots of both varieties than in control plants (Figure 6A). Compared with the controls, PEG treatment of plants did not affect the expression of MHA2 in the roots of Abelardo and Calo plants (Figure 6B), while it induced the expression of MHA3 in roots, especially in Calo seedlings (Figure 6C). In addition, PEG treatment resulted in a significant decrease of the MHA4 transcript (about 45% of control) in Abelardo roots, in contrast to Calo roots, for which MHA4 expression was significantly (almost 2-fold) increased (Figure 6D).
Figure 6
Relative changes in transcript levels of PM H+-ATPases-encoding genes MHA1-4 from maize roots of Abelardo and Calo variety. The plants were grown under control and osmotic stress (drought) conditions. Relative expression intensity was calculated using Pfaffl-equitation in comparison to tubulin as an internal control. Values are the means of three replicates ± SE. (*) indicates statistically significant differences (p < 0.05). (A) MHA1, (B) MHA2, (C) MHA3, (D) MHA4.
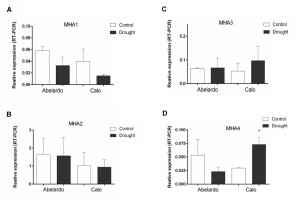
To detect the quantity of H+-ATPase protein in plasma membrane fractions isolated from the roots of stressed and non-stressed Abelardo and Calo plants, the Western-blot analysis using polyclonal antibodies was conducted. The signal intensity corresponding to plasma membrane H+-ATPase protein increased after plant treatment with PEG. Moreover, the level of PM H+-ATPases was distinctly enhanced in stressed roots of Calo plants, indicating that drought leads to an increase in the abundance of PM proton pumps in root tissues (Figure 7).
Figure 7
Immunodetection of PM H+-ATPase in plasma membrane fractions of maize roots. Plasma membrane proteins (20 mg) isolated from control and drought-stressed Abelardo and Calo plants were separated by electrophoresis, transferred onto nitrocellulose membranes, and immunoblotted with polyclonal antibodies raised against PM H+-ATPase (Agrisera AS07 260). M corresponds to the molecular mass of the protein marker in kDa. (1) Abelardo control, (2) Abelardo drought, (3) Calo control, (4) Calo drought.
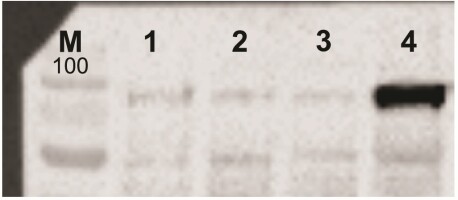
Multiple alignment of protein sequences from Arabidopsis thaliana (AHA), Nicotiana plumbaginifolia (PMA), Oryza sativa (OSA), Triticum aestivum, and Zea mays (MHA) PM H+-ATPases was conducted to construct a phylogenetic tree (Figure S3). The maize proteins were assigned to two (I and II – containing genes widely expressed in the plant organism) out of the five established subfamilies as previously described by Arango et al. (2003). Notably, MHA3 did not fit into any of the defined subfamilies and was thus excluded from the phylogenetic analysis. MHA2 and MHA4 were both classified under subfamily II, while MHA1 was grouped within subfamily I.
Polyisoprenoid levels in maize roots under osmotic stress
To gain basic insight into the polyisoprenoid metabolism of maize plants under osmotic stress, we first isolated total polyisoprenoids from the leaves and roots of stressed and non-stressed Abelardo and Calo plants and analyzed them by HPLC-UV. The obtained polyisoprenoid profiles show that maize plants accumulate a mixture (family) of polyprenols and dolichols of medium-length chains: from 13 to 17 isoprenoid units, with Pren- and Dol-15 dominating (Figure 8A, Figure S4A). In leaves, there was a domination of polyprenols over dolichols; in roots, the amounts of polyprenols were similar as in leaves, but dolichols were present at slightly higher levels, and the Pren:Dol ratio was closer to 1 (Figure 8B, Figure S4B). It has been previously shown that in Arabidopsis, polyprenols are mainly present in photosynthetic tissues, whereas dolichols are present in roots (Surowiecki et al., 2019); however, it is also known that the polyprenol:dolichol ratio differs significantly between plant species (Basyuni et al., 2017; Swiezewska & Danikiewicz, 2005).
Figure 8
Content of polyisoprenoid alcohols (polyprenols and dolichols) in the roots of Abelardo and Calo seedlings under control and drought stress conditions. There are shown representative HPLC-UV spectra of polyisoprenoid profiles for roots of non-stressed Abelardo and Calo seedlings (A), as well as a quantification of the obtained results per dry weight (B). The content of polyisoprenoid alcohols in PM was measured by LC-MS in isolated plasma membrane fractions from roots of stressed and non-stressed Abelardo and Calo seedlings (C). P – polyprenols, D – dolichols. All values represent means ± SD of three independent experiments. Significant differences (p < 0.05) based on unpaired t-tests with Welch’s correction are indicated with asterisks (*); ns stands for non-significant.
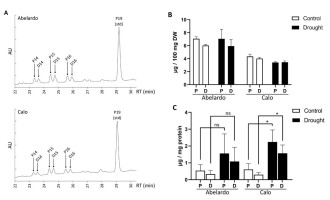
Interestingly, Abelardo plants contained more polyisoprenoids than Calo plants. Additionally, osmotic stress (achieved by PEG treatment) led to a slight increase in total polyprenol and dolichol content in the leaves, especially in the Abelardo cultivar, but at the same time, the content was stable or decreased slightly in the roots (Figure 8B, Figure S4B). These effects were minor.
Next, plasma membrane (PM) fractions from treated and non-treated maize roots were isolated, and polyisoprenoids were extracted and analyzed by LC-MS (Figure 8C). The same compounds were found here as in the total root extracts, but in untreated plants, no differences between the two cultivars were detected, showing that in Calo roots, a higher proportion of the total polyisoprenoids localizes to the PM than in Abelardo roots. In PEG-stressed plants a clear effect was observed: in both varieties we could see an increase in the content of polyprenols and dolichols in the PM fractions. In Abelardo roots, the increase was statistically insignificant due to large differences between biological samples: not all plants managed to achieve higher polyisoprenoid levels in the PM. In Calo roots, the response was consistent and significant. These results show that under drought conditions, relocation of polyisoprenoid alcohols to the PM from other cellular locations is taking place. In the roots of the drought-resistant Calo cultivar, this effect is stronger and more consistent than in the roots of drought-sensitive Abelardo seedlings, suggesting that PM relocation of polyisoprenoids could be contributing to the drought resistance of this maize cultivar.
. Discussion
The roots of plants play a critical role in sensing and responding to a variety of external environmental stimuli, primarily due to their direct contact with soil water and nutrients. Effective regulation of root growth is an essential adaptation for the efficient exploitation of water and nutrients during drought stress conditions (Li et al., 2022; Ludwiczak et al., 2021). Thus, it has been suggested that the acidification of the apoplast, which is maintained by plasma membrane H+-ATPases, may be involved in response to osmotic stress and could be one of the strategies for enhancing plant stress tolerance. In this study, we aimed to confirm the hypothesis of a correlation between an increase in the activity of PM H+-ATPase, its protein level, and total root elongation of the drought-tolerant maize Calo seedlings under osmotic stress (Figure 1, Figure 4, Figure 5, Figure 7). Our results revealed a significant increase in the activity of PM H+-ATPase in the drought-tolerant cultivar Calo under osmotic stress, indicating that plasma membrane H+-ATPase may promote the resistance of maize seedlings against osmotic stress. The increase in H+-ATPase activity was not observed in the drought-sensitive Abelardo plants, suggesting that this protein plays an important role in drought tolerance. These findings are consistent with previous research by Ovrutska & Bluma (2018), who reported that the maize drought-resistant cultivar Dostatok had higher H+-ATPase activity than the drought-sensitive cultivar Pereyaslivskiy. Additionally, screening of barley varieties with different levels of salt tolerance showed that salinity and droughttolerance correlated with higher basal PM H+-ATPase activity in root cells (Chen et al., 2008). Furthermore, in drought-sensitive Abelardo roots growing under short water stress, the activity of PM H+-ATPase and proton efflux were significantly reduced, leading to a decrease in primary root length (Figure 1, Figure 4, Figure 5). These results suggest that the activity of plasma membrane proton pumps is strongly correlated with root elongation and could potentially serve as a biomarker of drought resistance for maize varieties.
As suggested by previous research, the hydrolytic activity of the PM H+-ATPase may be hindered by the phosphorylation/dephosphorylation state of the pump protein. Fuglsang et al. (2007) reported that the protein kinase PKS5 is accountable for phosphorylating Ser-931 of the Arabidopsis AHA2 ATPase, which results in the binding of a 14-3-3 protein being prevented, thus keeping the enzyme in a state of low activity. Studies conducted on Arabidopsis roots showed that the RALF peptide that is secreted interacts with the surface protein kinase FERONIA. This interaction leads to the phosphorylation of Ser-899 of the PM H+-ATPase, inhibiting proton transport and resulting in RALF-induced extracellular alkalization and stunted growth (Haruta et al., 2014). Recent research has challenged the traditional theory of acid growth, proposing that low apoplastic pH promotes root growth. In their study, Li et al. (2021) discovered that auxin triggers two different signaling pathways that counteract each other, leading to the rapid regulation of the apoplastic pH, which is the direct growth-determining mechanism. Specifically, the PM H+-ATPase in roots is phosphorylated and activated via the cell surface receptor-like kinase Transmembrane Kinase 1 (TMK1), leading to apoplast acidification and promotion of root growth. On the other hand, intracellular TIR1/AFB-mediated signaling triggers cellular H+-influx, causing apoplast alkalinization and growth inhibition (Li et al., 2021).
The regulation of PM H+-ATPase activity is not solely dependent on protein modification through phosphorylation; it is also controlled by gene expression. In Zea mays, the plant PM H+-ATPase is encoded by a multigene family, and studies have identified four isoforms of the enzyme (MHA1, MHA2, MHA3, and MHA4) (Pii et al., 2016; Santi, 2003). The expression levels of these isoforms can affect H+-ATPase activity in the plasma membrane. Real-time RT-PCR results showed that, under control conditions, the relative transcription of MHA2 was unaffected by osmotic stress (Figure 6B). However, in Calo roots, a significant increase in MHA4 transcript was observed, while in Abelardo roots, the same transcript decreased (Figure 6D). These findings suggest that MHA4 is a particularly effective isoform of the PM H+-ATPase that plays an important role in proper root growth during maize’s adaptation to drought. Previous research has shown that isoforms MHA2 and MHA4, which are highly expressed in roots, may be critical for regulating root growth, particularly under adverse conditions such as salinity or soil water deficit (Kovalenko & Palladina, 2016).
According to Zhang et al. (2017), water stress has a negative impact on root growth and development, ultimately leading to impaired plant function. The results presented in this study demonstrate that osmotic stress caused a reduction in leaf biomass (Figure 2C–D) and a decrease in chlorophyll content in both cultivars tested (Table S2). These pigment-related responses not only provide valuable insights into the physiological adjustments of maize plants under osmotic stress but also emphasize the importance of considering multiple indicators, including pigment concentrations, in assessing plant stress responses. The variations in photosynthetic pigments complement the observed changes in plasma membrane H+-ATPase activity, offering a holistic view of the adaptive strategies employed by different maize varieties in response to osmotic stress. Interestingly, anthocyanin accumulation was also observed (Table S2), which has been shown to help regulate water homeostasis by acting as an osmoregulator. Plants can increase anthocyanin production in response to drought stress through transcriptional regulation of anthocyanin biosynthesis genes (Naing & Kim, 2021). Nakabayashi et al. (2014) similarly demonstrated that drought-induced anthocyanin accumulation in Arabidopsis is associated with increased tolerance to oxidative- and drought stress. The interplay between changes in plasma membrane H+-ATPase activity and the modulation of photosynthetic pigments highlights the nuanced and multifaceted nature of plant responses to osmotic stress. These secondary metabolites, which protect plants from growth inhibition under water stress conditions by reducing oxidative stress, may serve as useful biochemical markers for breeding drought-tolerant maize. The relatively low water potential of root cells also causes the accumulation of osmoprotectants, including proline, which undoubtedly affects the maintenance of proper turgor and the proper functioning of membrane and cytosolic proteins in cells (Shao et al., 2008; Zulfiqar & Ashraf, 2021). Proline is the most common endogenous osmolyte accumulated under various abiotic stresses, including salinity (Slama et al., 2015; Szabados & Savouré, 2010). Accumulation of a high concentration of proline in Abelardo and even higher in Calo roots was noticed under osmotic stress (Figure 3). The increased proline concentration under drought stress has been reported by Aslam et al. (2015), which is in line with our results. Transgenic wheat plants that accumulate higher Pro than wild type exhibited less lipid peroxidation of membranes during osmotic stress, indicating a role for Pro in reducing ROS damage during osmotic stress (Vendruscolo et al., 2007). ROS production by plants has been associated with responses to physiological stress, and polyprenols might potentially play the role of scavengers for reactive oxygen, protecting cells from oxidative stress. It has been postulated that unsaturated plant polyprenols and dolichols have antioxidant potential and may be responsible for maintaining the fluidity of cell membranes (Swiezewska & Danikiewicz, 2005). Baczewska-Dąbrowska et al. (2022) showed that under salt stress in street conditions, the leaves of Tilia × euchlora contained more polyprenols than non-saline growing leaves (Baczewska-Dąbrowska et al., 2022). These results suggest that the accumulation of polyisoprenoids in leaves might mitigate salt stress, possibly by acting as free radical scavengers and blocking the transport of sodium and chloride to leaves. Here, we show for the first time the polyisoprenoid profiles of maize leaves and roots. Our data shows that although in maize, the total pools of polyisoprenoids do not change under osmotic stress, in roots, the PM-associated pools increase, which confirms that they may play a role in adaptation to drought conditions. Polyisoprenoids have been shown to increase the fluidity and permeability of model membranes (Janas et al., 2000). Interestingly, thylakoids isolated from leaves of an Arabidopsis mutant deficient in polyprenols adopt an increasingly “fluid membrane” state (Akhtar et al., 2017). Even though the enhanced accumulation of polyprenols and/or dolichols is observed in numerous animal and plant tissues upon environmental stresses, the mechanism of their involvement in cellular responses to changing conditions is not clear (Surmacz & Swiezewska, 2011). For example, upon osmotic stress (sorbitol treatment of Arabidopsis hairy root cultures), the content of dolichols in the tissues increased considerably, and this effect was accompanied by the modulation of the relative activity of the isoprenoid-generating pathways in plant roots, with an increased contribution of the plastidic methylerythritol phosphate (MEP) pathway (Jozwiak et al., 2017). Moreover, the phenotype of the Arabidopsis thaliana mutant lew1, deficient in the synthesis of polyisoprenoid chains, revealed that the products are important for plant responses to drought stress (Zhang et al., 2008). Taken together, these data show that polyisoprenoids play a role in adapting plants to certain stress conditions. Numerous studies have been undertaken to dissect the metabolic responses of plants to abiotic stresses, which involve intra- and extracellular signaling and modifications of the cellular metabolism (Obata & Fernie, 2012). Recently, research has demonstrated that exogenous applications of polyamine to tea plants improve fatty acid unsaturation and plasma membrane H+-ATPase activity, which are associated with enhanced drought tolerance. Moreover, such application can increase polyphenol content and reduce ROS levels stimulated by drought stress (Na et al., 2022). Our findings indicate that maize plants may increase proline content (Figure 3) to maintain osmotic adjustment and enhance antioxidant components, such as anthocyanins (Table S2) and plasma membrane-bound isoprenoid alcohols (Figure 8), to achieve better protection against ROS under water stress. The accumulation of isoprenoids, possibly due to their putative protective role in osmotic stress conditions, may contribute to maintaining the correct structure and function of PM regulatory proteins and the plasma membrane. Furthermore, recent experiments have demonstrated that H+-ATPase activity is reliant on the sterol content of the plasma membrane.
H+-ATPase activity is partly associated with an enhanced steady-state amount of H+-ATPases in the plasma membrane of maize seedling roots. Some interesting responses to ROS were observed in Arabidopsis and maize plants. For example, ROS accumulation in the root apex regulates the activity of calcium channels required for the polar growth of root hairs (Carol, 2006; Liszkay et al., 2004). Furthermore, auxin-dependent pH acidification of the apoplast and high superoxide accumulation facilitate cell wall loosening, which is required for cell elongation (Tognetti et al., 2012). Summarizing, expression of the MHA4 gene distinctly increased under osmotic stress along with stimulation of hydrolytic activity and of H+ pumping in drought-tolerant Calo versus drought-sensitive Abelardo seedlings. The increase in MHA4 expression and in PM H+-ATPase activity was closely associated with the total root growth (total root length, fresh and dry biomass) and with anthocyanin and proline content, which are critical for enhanced drought tolerance in plants. The deeper root penetration allows the plant to get greater exploration of soil for water and nutrients. Therefore, it is essential to enhance vertical root distribution to improve crop growth and development under drought stress.
. Concluding remarks and future directions
Taken together, the findings presented in this study unveil a novel perspective in plant physiology, emphasizing the pivotal role of plasma membrane proton pumps and associated physiological stress responses in the maintenance and promotion of root growth during drought conditions. The notable up-regulation of PM H+-ATPase activity, both at the transcriptional and post-transcriptional levels, signifies a previously underexplored aspect of environmental plasticity within the root system, intricately tied to the functional integrity of plasma membrane proton pumps. However, the mechanisms underlying the integration of PM H+-ATPases and signaling pathways via hormones such as auxins, brassinosteroids, and ABA in modulating root growth under drought remain unclear and require further investigation.
From an agricultural perspective, the plasma membrane H+-ATPase is an interesting target for enhancing sustainable crop production. Understanding the complexity of plasma membrane proton pump regulation in crops might provide new strategies for developing crops that are more tolerant to drought stress and other harsh environmental conditions. Interference RNA (RNAi) and CRISPR-Cas9 technology could be used to edit the promoter region of PM H+-ATPase and enhance its expression, leading to up-regulation of the PM H+-ATPase and increased tolerance to drought stress in crop plants. These insights underscore the urgency for further research to develop technologies and strategic interventions to enhance crop productivity. In the face of changing climatic conditions, this research not only contributes to our understanding of plant responses to stress but also underscores the imperative to innovate and secure global food production.
. Supplementary material
The following supplementary material is available for this article:
Figure S1. A comparison of NDVI values for Abelardo and Calo maize seedlings grown under control or drought stress (PEG) conditions. Values represent means ± SE of three independent experiments. Significant differences based on Sidak’s post-hoc test (p < 0.01) are indicated with an asterisk (*).
Figure S2. Representative kinetic curves are presented. The reaction was started by adding ATP.
Figure S3. Phylogenetic analysis was conducted on PM H+-ATPases from maize (Zea mays), Arabidopsis thaliana, Nicotiana plumbaginifolia, Triticum aestivum and rice (Oryza sativa) in this study. The amino acid sequences of PM H+-ATPases, including MHA1, MHA2 and MHA4 from maize, AHA1–AHA11 from A. thaliana, PMA1–PMA6, PMA8, and PMA9 from N. plumbaginifolia, two isoforms 1 and 2 from Triticum aestivum and OSA1–OSA3 from O. sativa, were compared to generate a phylogenetic tree. The Neighbor–Joining method of MEGA5.05 (http://www.megasoftware.net/) was employed to construct the phylogenetic tree. The bootstrap test with 1,000 replicates was performed to assess the reliability of the tree, and the percentage of replicate trees in which the associated taxa clustered together is indicated next to the branches.
Figure S4. Content of polyisoprenoid alcohols (polyprenols and dolichols) in the leaves of Abelardo a.nd Calo seedlings under control and drought stress conditions. Shown are representative HPLC-UV spectra of polyisoprenoid profiles for leaves of non-stressed Abelardo and Calo seedlings (A), as well as a quantification of the obtained results per dry weight (B). P – polyprenols, D – dolichols. All values represent means ± SD of three independent experiments.
Table S1. Gene-specific primer sequences for real-time PCR.
Table S2. Pigment content in maize leaves (Abelardo and Calo) under control and under drought stress conditions. Values present means ± SE of three independent experiments.