. Introduction
Taphrina deformans (Berk/Tul) is the causative agent of peach leaf curl. All species of the Taphrina genus are dimorphic (Kramer, 1987; Mix, 1935; Rodrigues & Fonseca, 2003). In its biological cycle, the pathogenic fungus had a strictly expressed parasitic mycelial stage, which ended with the formation of asci with ascospores in the infected plant tissues (Caporali, 1965). The pathogen could not be detected visually during the saprophytic asymptomatic stage. The classical way of its detection consisted in isolation of the yeast-like form known as Lalaria deformans R. T. Moore (Moore, 1998; Tavares et al., 2004).
Plant leaves can be adapted to different abiotic and biotic conditions (Taratima et al., 2021). The parasitizing dikaryotic mycelium was found to develop mainly in the intercellular spaces of the mesophyll tissue. Infected leaves had increased levels of cytokinins, indole-3-acetic acid, and tryptophan. Due to hypertrophy and hyperplasia, the symptoms led to deformation of leaves and, less often, fruits and shoots (Giordani et al., 2013; Matsuyama & Misava, 1961; Syrop, 1975; Sziraki et al., 1975; Tsavkelova et al., 2006). Anatomical changes were observed in infected leaves around the hyphae of the parasitizing mycelium of T. deformans, associated with an alteration in the shape of mesophyll cells, the volume and lamellar system of chloroplasts, the absence of starch grains, and a reduced number of chloroplasts (Hansen et al., 2007; Huang et al., 1993). The number of active dictyosomes and free ribosomes was reported to increase as well (Marte & Gargiulo, 1972; Syrop, 1975). The resulting increased vesiculation of the plasma membrane changed its permeability, which caused loss of electrolytes, amino acids, and sugars from the infected cells. In addition, the cellulase activity of T. deformans-infected leaves, an indicator of the synthesis of cellulose-degrading enzymes, was enhanced (Bassi et al., 1984).
Electron microscopy has become a routine method to visualize, elucidate, and probe the nature of host-pathogen relationships (Czymmek et al., 2023; Łotocka et al., 2023). Changes occurring in the dynamic plant-pathogen system suggested altered cellular functions due to biotic stress (Park, 2006). The physiological and biochemical status of infected leaves changed. The impact of T. deformans on the metabolism of infected leaves was related to a decrease in the rate of photosynthesis and the content of plastid pigments, carbon balance, gas exchange, changes in respiration, chlorophyll fluorescence, and CO2 assimilation (Moscatello et al., 2017; Piperkova & Vasilev, 2000; Raggi, 1994, 1995). The reduced chlorophyll content was not related to increased chlorophyllase activity. The enzymatic activity was significantly reduced to almost the same extent as the chlorophyll content, suggesting a relationship between the reduction of chlorophyllase activity and the reduced capacity for chlorophyll biosynthesis in infected cells (Montalbini & Buonaurio, 1986). It was supposed that the cause of the reduced photosynthetic activity was the lower number of chloroplasts in cells located around the parasitizing hyphae of the pathogen (Huang et al., 1993; Marte & Gargiulo, 1972; Syrop, 1975).
Studies of the T. deformans genome allow the identification of important essential genes for the pathological process. The genome includes genes related to the degradation of the cell wall (cellulases and cutinases), the synthesis of secondary metabolites facilitating the infection process, and the biosynthesis of plant hormones responsible for the characteristic leaf deformations, as well as some genes that determine the resistance to fungicides (Cissé et al., 2013).
In our previous studies, we confirmed the changes in the physiological, biochemical, and anatomical parameters in T. deformans-infected leaves mentioned above and highlighted some details of the pathological process (Koleva-Valkova et al., 2017; Piperkova, 2023; Piperkova & Vasilev, 2000; Semerdijeva et al., 2014). Nevertheless, the structural-functional picture of the pathogen–host interaction is not fully clear. The aim of our study was to show some details in ultrastructural changes induced by T. deformans and their connection with the functional disorders in peach leaves.
. Materials and methods
Plant material
Fully developed leaves of peach (Prunus persica (L.) Batsch), both healthy and infected by T. deformans, were collected in April 2018 from 5-year-old peach trees (cv. Fayette) grown in the Experimental Field of the Agricultural University of Plovdiv, Bulgaria. The soil of the field (Latitude 42°8′19″ N, Longitude 24°48′7″ E) is Mollic Fluvisols. The plant material was collected at the peak of the peach curl disease before the formation of asci with ascospores.
Transmission electron microscopy (TEM)
The mesophyll ultrastructure was studied by transmission electron microscopy following the protocol described by Doncheva et al. (2009) and Giordani et al. (2013). Briefly, approx. 2 mm2 leaf segments from the middle of fully developed leaves of cv. Fayette (control and naturally infected by T. deformans) were fixed in 3% (v/v) glutaraldehyde in 0.1% M Na-cacodylate buffer (pH 7.2) for 12 h at 4 °C and then rinsed three times in the same buffer. The samples were postfixed in 1% (w/v) OsO4 in the same buffer for 3 h. The samples were dehydrated in ethanol series (from 25 to 100%) and embedded in Durcupan ACM (Fluca, Sigma-Aldrich). Ultrathin sections (75–90 nm thick) were cut with a Reicherd Jung ultramicrotome (Wien, Austria). The sections were stained with uranyl acetate and lead citrate (Reynolds, 1963). Observations of the ultra-thin sections were performed using a JEOL 1200 EX (Tokyo, Japan) electron microscope.
. Results
Healthy mesophyll cells
The ultrastructure of the healthy leaves showed oval-cylindrical mesophyll cells. In the cytoplasm, chloroplasts and the usual organelles: mitochondria, Golgi apparatus, and nuclei were found around a central vacuole. All of them had a well-developed membrane system (Figure 1A). The plastid apparatus had typical chloroplast architecture with a developed internal grana membrane connected with long stroma thylakoids oriented parallel to their longitudinal axis. Plastoglobules and small starch grains in the chloroplasts were found (Figure 1B).
Infected mesophyll cells
The mesophyll cells of the T. deformans infected leaves had an altered shape from oval-cylindrical to isodiametric. A centrally located vacuole was found, which restricted the cytoplasm to the cell wall, confining it to a thin layer thickened around the chloroplasts and the nucleus (Figure 2A–B). The ultrastructure of the chloroplasts showed a change in their shape and degradation of their membrane systems, thylakoids, grana, and stromal lamellae, as shown by Ghosh et al. (2017) in other fungal diseases. Abnormal, spherical, and irregularly oval chloroplasts with electron dark stroma between thylakoids were found. Large starch grains and cytosol were visualized in the chloroplasts as a membrane-bounded inclusion (Figure 2C–D). The destructive processes in the mesophyll cells of leaves affected by peach leaf curl spread to extensive areas with residual fragments of the rough endoplasmic reticulum and other completely intact cell compartments (Figure 2C, Figure 2E, Figure 3A). Active Golgi dictyosomes were found in the cytoplasm (Figure 2E). The middle lamella was enlarged and was penetrated by hyphae of the pathogen (Figure 2A). The ultrastructural analysis of the T. deformans infected leaves showed contact between the cell walls of the pathogen and the host. The wall of the mycelial cell had a lower electron density than that of the plant cell. Probably, the mycelial cell wall not only established a mechanical contact but also penetrated the host’s cell wall (Figure 3A, Figure 3C–D).
Figure 2
TEM micrographs of part of the infected peach leaf. Parasite hyphae (H) growing in the middle lamella (ml) between the hypertrophied cells: (A) magnified 2500x; (B) magnified 4300x. Signs of regression of the chloroplast with a changed shape near the mesophyll cell wall (mcw) show large starch granules (Sg) and cytosol (Cy) as a membrane-bounded inclusion: (C) magnified 25000x; (D) magnified 26000x. Details of the infected mesophyll show active Golgi bodies (G) and rough endoplasmic reticulum (RER) in the mesophyll cell (Mc) around pathogenic hyphae: (E) magnified 7500x. Plasmodesmata (Pd) are numerous between infected mesophyll cells: (F) magnified 7000x.
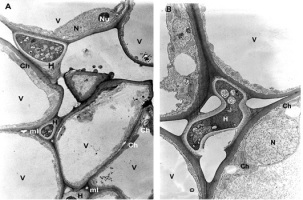
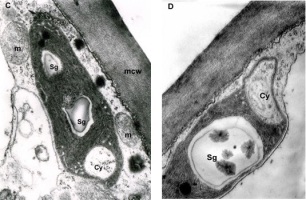
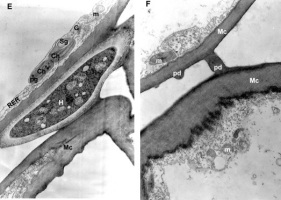
Figure 3
TEM micrographs. Details of unusual connection between parasite hyphae (H) and mesophyll cells (Mc) with loosening of the host cell wall: (A) and (B) magnified 18000x; (C) magnified 14000x; (D) magnified 18000x.
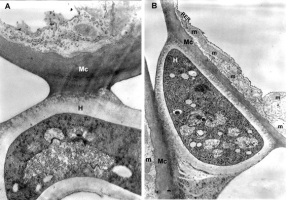
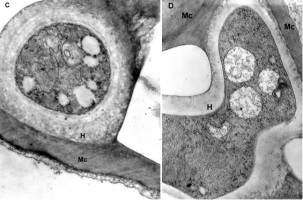
The transport of assimilates in the leaves was carried out via the symplast pathway by the plasmodesmata using the intercellular endoplasmic reticulum. The high functional load of the tissues determined the formation of more plasmodesmata (Figure 2F).
. Discussion
The harmful effect of peach leaf curl resulted from the biotic stress associated with pathological structural-functional changes induced by T. deformans. As a result, the leaves prematurely senesced and fell off, which affected the plant fruit yield and frost resistance.
The T. deformans-induced synthesis of phytohormones resulted in severe hypertrophy and alterations in the shape of the host mesophyll cells (Sziraki et al., 1975). Hypertrophied cells dedifferentiated and re-acquired the capacity to divide and actively multiply, i.e., hyperplasia occurred. Young cells were developed, which, together with the old ones, formed heterogeneous tissue (Marte & Gargiulo, 1972). The results of the ultrastructural analysis supported our previous findings on pathogen-infected leaves of the Fayette cultivar obtained by light microscopy. A statistically significant difference of 76.58 µm increased thickness of the mesophyll tissue was found as well as an alteration in the shape of the cells of the palisade layer from oval cylindrical to isodiametric. The altered mesophyll differentiation was associated with a change from a dorsoventral to isolateral leaf lamina type (Semerdijeva et al., 2014). We suggested that the hypertrophy of the mesophyll cells may have been caused by the reduced lignification of the cell walls, which was indirectly evidenced by the reduction of their content of total polyphenols and the increased amount of the anti-stress enzyme syringalgazine peroxidase (Koleva-Valkova et al., 2017).
The parasitizing hyphae of T. deformans grew larger in the intercellular matrix of the mesophyll cells, which was possible due to the expanded middle lamella, as proposed by Giordani et al. (2013). The pathogen made its way through mechanical impact and the synthesis of enzymes breaking down polygalacturonic acids. Increased amounts of such enzymes (polygalacturonases, pectinesterases, and galactanases) were also found in other fungal diseases (Jarvis et al., 1981). The partial dissolution of the cell walls was caused through the synthesis of polysaccharide-degrading enzymes, including cellulase. Bassi et al. (1984) proved the ability of T. deformans to synthesize cellulose-degrading enzymes.
The alteration in the plasma membrane of the mesophyll cells, around which the pathogen hyphae were located, was often accompanied by the degradation of the cell walls (Figure 2A–B, Figure 3A–D). The boundary between the cell walls of the plant cells and the T. deformans hyphae was not distinguishable. The pathogen may have penetrated the mesophyll cell walls (Bassi et al., 1984; Caporali, 1965). The effect caused by the fungal infection has been reported to be more pronounced in susceptible peach cultivars than tolerant ones (Giordani et al., 2013). The genome of T. deformans encodes a complete set of carbohydrate-active enzymes, including those required to degrade plant cell walls. The pathogen contains cellulases and xylanases, which can degrade cellulose and hemicellulose, but has no lignin-degrading enzymes (Cissé et al., 2013). This fact confirms the possibility of the pathogen parasitizing hyphae to enter but not wholly disrupt the cell walls of infected leaves. Syrop (1975) believed that the pathogen and host cells did not establish contact. Bassi et al. (1984) suggested the existence of a boundary line between them. Our analysis of the ultrastructure of the mesophyll cells infected with T. deformans visualized the partial degradation of the host cell walls and supported the findings reported by Cissé et al. (2013).
Chloroplasts are one of the most dynamic organelles in the plant cell involved in photosynthesis and the synthesis of the main phytohormones. They play an active role in the protective response to plant stress and are crucial for inter-organelle signaling. These plastids are transferred from optimal places for photosynthesis to areas near the nucleus or the pathogen (Bhattacharyya & Chakraborty, 2018). Chloroplasts play a central role in the protective response of plants to infection by pathogens through the synthesis of secondary metabolites and protective compounds as well as phytohormones, such as jasmonic acid and salicylic acid (Kretschmer et al., 2020). The destruction of chloroplasts found in the present study, manifested by an alteration in their shape and structure, was correlated with alterations in their functions (Marte & Gargiulo, 1972). Unfortunately, the mechanism in which fungal plastid effectors are first secreted from fungal cells, pass through the biotrophic interface safely, and translocate into the cytoplasm and then into chloroplasts is still enigmatic and awaits future research (Kretschmer et al., 2020). The presence of assimilable starch in chloroplasts is not a constant feature. The number, size, and amount of starch grains vary greatly. These parameters depend on the ontogenetic age of the organelles and the structural and functional state of the tissues. The dynamics of accumulation of the assimilates and the amount of starch grains in chloroplasts are related to the degree of development of the plastid apparatus, the expansion of the reticulum, and the transformation of part of its elements into vacuoles. During the chloroplast development, single large starch grains are formed in the stroma. However, the accumulated starch is not a product of photosynthesis. Chloroplast senescence is a process characteristic of the temperate climatic zone associated with leaf fall. Chlorophyll is destroyed, and a large amount of starch is accumulated, subject to rapid outflow, after which the destruction of thylakoids begins with the associated increase in the number of plastoglobules (Koleva & Chakalova, 2008).
In the present study, the ultrastructural imaging showed that the chloroplasts in the T. deformans-colonized mesophyll cells had probably not completed their ontogenetic development and senesced prematurely. This may be associated with the impact of specific fungal protein effectors that influence photosynthesis, biosynthesis, and stability of chlorophyll as well as the formation of reactive oxygen species (ROS) (Kretschmer et al., 2020; Moscatello et al., 2017). This statement was confirmed in our previous studies, where we found that T. deformans-infected leaves exhibited reduced content of photosynthetic pigments (Koleva-Valkova et al., 2017). This was visualized by the formation of chlorotic or anthocyanin hypertrophied areas on the petiole in the peach leaf curl infected plants. In addition, the photosynthetic rate in the leaves was also decreased in the range from 17 to 45% (Piperkova & Vasilev, 2000).
Peach leaves infected with T. deformans were characterized by an active metabolism at the early stage of the disease (Marte & Gargiulo, 1972). Our earlier studies proved it by the enhanced respiration intensity in the infected leaves (Piperkova & Vasilev, 2000). The more intensive metabolism was provoked to satisfy the increased energy needs of the infected plant cells. This was proved by the presence of active mitochondria, active dictyosomes, and ribosomes on the tubules of the endoplasmic reticulum in the cells colonized by the pathogen. The stronger, unbalanced growth of the infected leaves and their negative carbon balance could be explained to a certain extent by the presence of compensatory mechanisms. The significant accumulation of cytokinin and auxins in infected leaves (Sziraki et al., 1975) probably increased their attractive power for photoassimilates. According to Goodman et al. (1986), the photoassimilates were redirected from healthy leaves, which had increased CO2 fixation, to the diseased ones and thus partially compensated for their carbohydrate deficiency. As the disease progressed, the metabolic activity was disrupted, and the infected tissues prematurely senesced and died.
. Conclusions
The performed TEM analysis of the mesophyll cells of healthy and T. deformans-infected peach leaves showed significant ultrastructural differences. Pathogenic hyphae penetrated the expanded middle lamella, entered the cell walls of the infected plant cells, and affected their structure. The connection between the pathogen T. deformans and plant cells in infected leaves was visualized. Strong destructive processes were detected, leading to unbalanced growth and alterations in the shape of the colonized cells, which appeared to be free of organelles. The latter were pushed into a thin cytoplasmic layer near the cell wall. Signs of degradation were also established in the chloroplasts. These ultrastructural alterations were associated with disruption of the mesophyll cell functions. As a result, the infected leaves senesced rapidly and died.