. Introduction
The development of plants is controlled not only by the genetic information they carry but also by the external environment. Light is one of the most critical factors determining a plant’s proper development and yielding. By correctly choosing the lighting conditions (both the quality and quantity of the spectrum), it is possible to modulate the plants’ growth, which is an attractive and more eco-friendly alternative to chemical growth regulators (Paradiso & Proietti, 2022).
Modern horticulture is facing resource shortages, such as land area, irrigation water, etc. Moreover, vegetables are traditionally grown in soil-based open field systems, and in most regions, it is impossible to grow most crops stably year-round with high quality because of seasonality, environmental extremes, and pests (Nguyen et al., 2021). Production of plants indoors in a controlled environment has several advantages over traditional cultivation methods in the glasshouse or field, such as easier agrotechniques, decreased diseases and pests, and increased product quantity and quality (Costa et al., 2014).
Indoor cultivation of vegetables requires the usage of artificial lighting systems. Over the years, several types of lamps have been used, such as high-pressure sodium lamps (HPS), metal halide (MH) lamps, and fluorescent tubes (FL). However, the distribution and variation of the spectrum of conventional light sources are fixed and may not be ideal for the light requirement of different plant species. In recent years, light-emitting diodes (LEDs) have become vastly popular and used in practical lighting solutions. LEDs have great potential for horticultural lighting due to their energy efficiency, longevity, and flexibility of use. Moreover, LEDs are becoming more suitable for research and commercial horticulture under controlled conditions due to their low radiation, heat, and broad spectral match. LEDs are constantly improved regarding transparent covering, emitter materials, and secondary optics. New technologies for their production are also developed. A dynamic increase in demand for these lamps leads to a drop in their price (Hamedalla et al., 2022; Sabzalian et al., 2014;).
In horticulture, LEDs can be effectively used in indoor cultivation to improve plant growth (Yan et al., 2019). They are the first light source to provide the capability of accurate spectral composition control, allowing the wavelengths to match plant photoreceptors to optimize production and influence plant morphology and biochemical activity. While photosynthesis does not require continuous light of full spectrum, LEDs can produce sufficient photon fluxes of specific wavelengths on and off rapidly (Yeh & Chung, 2009). Red and blue wavelengths of visible light are absorbed by chlorophylls and are essential for photosynthesis and growth. Moreover, various wavelength ranges in and around the visible spectrum, such as ultraviolet and far-red, act as specific signals that are absorbed by photoreceptors in plants, i.e., phytochromes (red and far red radiation), cryptochromes (blue and UV-A radiation), and phototropins (blue radiation). Reception of light signals through photoreceptors leads to the induction of metabolism in plants (Kim et al., 2014). On the other hand, LED lamps, as sophisticated semiconductor devices, require a higher degree of hardware and lighting design with controls compared to other light sources (Wu et al., 2020). Several crop plants, such as lettuce, radish, and spinach, have been tested under various light conditions using LEDs (Nair & Dhoble, 2015). Nonetheless, much research must still be focused on improving crop yield in different plant species by adjusting the wavelength of light emitted by LEDs.
Cucumber (Cucumis sativus L.) and tomato (Solanum lycopersicum L.) are among the most important vegetables in the human diet. Even though their nutritional value is limited, the massive consumption of these crops makes them an essential source of macro and micronutrients (Yahia et al., 2019). The seedling stage crucially affects the growth and yielding of vegetable crops. Production of high-quality seedlings that can be well adapted when transplanted into the field or greenhouse is of great importance for cucumber and tomato producers (Kim et al., 2014). Therefore, studies on the possible indoor production of high-quality seedlings of these crops using modern and cost-effective lighting systems are desired.
The aim of this study was to verify the usefulness of wide-spectrum light-emitting diodes in the indoor production of cucumber and tomato seedlings. The scientific hypothesis assumed that LEDs should be an efficient and cost-effective alternative to traditionally used fluorescent lamps for cultivating high-quality seedlings due to the specific light spectrum affecting both photosynthesis and photomorphogenesis.
. Materials and methods
Seeds of cucumber (Cucumis sativus L.) ‘Parys F1’ and tomato (Solanum lycopersicum L.) ‘Poranek’ were sown at the end of March 2019, individually into 0.25-L plastic pots (7 × 7 × 9 cm), filled with a mixture of peat substrate (Hartman, Poznań, Poland) and perlite (Perlit, Šenov u Nového Jičína, Chech Republic) (2:1, v/v) and placed in a glasshouse (53°07′12.0″ N 18°00′29.4″ E) in natural photoperiod.
At the beginning of April, during the first leaf emergence phase, the plants were transferred to a non-sterile growth room with a controlled environment (22 °C, 16-h photoperiod, 65% RH). Pots with plants were distributed evenly on shelves (30 plants per shelf) illuminated with the following LED tubes: AP67, AP673L, or G2 (T8 tubes, L-series, Valoya, Helsinki, Finland, Table 1). Control plants were grown under standard cool daylight TLD 54/36W fluorescent tubes (FL) with a color temperature of 6200 K (Koninklijke Philips Electronics N.V., Eindhoven, the Netherlands). The PPFD was measured using an FR-10 photometer (Optel, Opole, Poland) at the central area of an empty shelf surface and was established from 50 (G2; maximal level) to 65 µmol m−2 s−1 (FL, AP67, and AP673L) by adjusting the distance between the light source and the shelf surface. Next, the light spectrum and relative intensity for each light source were measured with the spectroradiometer (Spectro Light, Łódź, Poland) (Figure 1).
Table 1
Applied LEDs spectrum data (Valoya, Helsinki, Finland).
Figure 1
Relative intensity and spectral characteristics of the light sources (FL, AP67, AP673L, G2) used in the experiment, provided by the spectroradiometer (Spectro Light, Łódź, Poland).
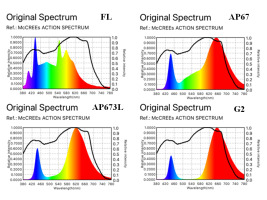
The plants were grown for three weeks. During this period, the seedlings were watered daily, and the temperature of the substrate was measured with a standard thermometer.
At the end of the experiment, at the beginning of May 2019, a comparative analysis of the plants was performed. The leaf chlorophyll content index (CCI) was measured with a CCM-Plus chlorophyllmeter (Opti-Sciences, Hudson NH, USA). The biometrical evaluation included the number of leaves, shoot length (cm), shoot and root fresh weight (g FW), and length of the longest root (cm). Moreover, a detailed analysis of the root system architecture was performed, i.e., the total length of the root system (cm), root area (cm2), volume (cm3), and mean root diameter (mm). For this purpose, root systems were excised from the shoots and scanned (Epson STD4800 scanner, Suwa, Japan) in transparent polypropylene cuvettes filled with water. Next, pictures of root systems were analyzed using the imaging software WinRHIZOTM (Reagen Instruments, Quebec, Canada).
The results were statistically verified using the analysis of variance (ANOVA) for a one-way experiment in a completely randomized design. Object means ± standard deviations (SD) were compared with Tukey’s test at the significance level p = 0.05.
. Results
The type of applied light source significantly affected the heating of the cultivation substrate (Figure 2). Control FL tubes kept the highest temperature throughout the experiment compared to all LED modules. On the other hand, the lowest substrate temperature was reported with G2 lamps.
Figure 2
Substrate temperature during the cultivation of cucumber and tomato seedlings on the shelves equipped with various types of light sources (FL, AP67, AP673L, G2).
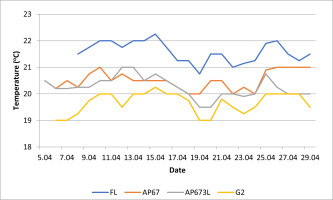
A comparison of tomato and cucumber shoots grown in various light conditions is shown in Figure 3 and Figure 4. At the beginning of the experiment, all cucumber shoots looked the same and were of similar height. In the second week, differences between the plants were observed depending on the light source. Plants grown under the G2 tubes became significantly longer. At the end of the experiment, their leaves contained less chlorophyll than those of the other experimental combinations (Table 2). Moreover, seedlings grown under the G2 lamp had the longest and flaccid shoots (17.52 cm) (Figure 3). On the other hand, the aerial parts of plants grown under FL and AP673L LEDs did not differ significantly in terms of the studied traits (Table 2). There was also no impact of the studied factor on any of the analyzed root parameters in cucumber (Table 3), including the longest root length (data not shown).
As for tomato, the seedlings were of comparable size at the beginning of the research. In the second experimental week, however, obvious differences in the habit of the plants were noticed, depending on the lamps used. All tested LEDs stimulated the elongation of shoots, which made the seedlings limp (Figure 4). In the last week of the experiment, the plants had the same chlorophyll content, regardless of the light spectrum used (7.90–9.00 CCI). However, seedlings from the control object (FL) were more compact (lower number of leaves, shoot length, and fresh weight) than those grown under LEDs (Table 2). There was also an evident influence of the light spectrum on the development of the root system in tomato (Figure 5). Roots produced in the control object were shorter (133.3 cm) and of the lowest fresh weight (0.08 g) and volume (0.13 cm3) compared to those from the experimental objects with LEDs (199.36–270.32 cm; 0.23–0.60 g; 0.26–0.29 cm3, respectively; Table 3).
Figure 3
Growth of cucumber ‘Parys F1’ seedlings in the following weeks of the experiment (bar = 3 cm), NA – not available.
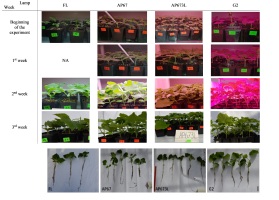
Figure 5
Root scans of cucumber ‘Parys F1’ (upper row) and tomato ‘Poranek’ (lower row) at the end of the experiment (bar = 3 cm).
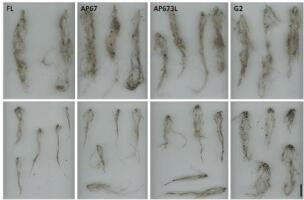
Table 2
Parameters of the aerial parts of cucumber ‘Parys F1’ and tomato ‘Poranek’ seedlings, depending on the light source (FL, AP67, AP673L, G2) used.
Table 3
Parameters of cucumber ‘Parys F1’ root systems and tomato ‘Poranek’ seedlings, depending on the light source (FL, AP67, AP673L, G2) used.
. Discussion
Changing climatic conditions have resulted in more persistent droughts, floods, and storms, as well as increased activity of pests and diseases that pose severe threats to agriculture. A potential solution to this situation is to endeavor indoor and urban farming, enhanced by proper lighting conditions, such as LEDs, thereby eliminating weather-related crop failures (Nair & Dhoble, 2015).
One of the main advantages of LEDs over other lighting systems is that they generate much less heat and, therefore, generate less cooling costs (García-Caparrós et al., 2020). This was confirmed in the present study as LEDs kept lower substrate temperatures than FL tubes. The lowest substrate temperature was reported with G2 lamps, probably due to a lower light intensity used in this experimental object (50 µmol m−2 s−1).
The present study found that seedlings of cucumber grown under the G2 tubes had the longest and flaccid shoots. Moreover, their leaves contained less chlorophyll than those of the other experimental combinations. The probable reason for this phenomenon is the meager share of green light (2%) in those lamps, a high share of unfavorable far red (25%), and a very high blue: green light ratio (25.9). Green light is considered the least efficient wavelength in the visible spectrum for photosynthesis. However, because of its lower absorptance, it can penetrate deeper than red or blue light and increase leaf photosynthesis in the lower chloroplasts. Green light also regulates plant architecture (Liu & van Iersel, 2021). Likewise, LEDs with a high share of red to blue light (70:30) stimulated the elongation of cucumber ‘Built No. 4’ plants (Hamedalla et al., 2022). Therefore, the G2 LED module should not be used commercially with this species, as compact growth is preferable in seedling production (Randall & Lopez, 2014). It is also not recommended to use the AP67 lamp due to the supra-optimal features of seedlings, i.e., too long shoots (13.86 cm) with a small number of leaves (3.80) and a slightly lower chlorophyll content (15.41 CCI). Conversely, since LEDs consume less energy and have a longer life span, generating fewer costs than traditional fluorescence tubes (Kulus & Woźny, 2020), the AP673L diodes can be recommended to produce cucumber ‘Parys F1’ seedlings. Since the results presented by Lanoue et al. (2021) indicate that mini-cucumber ‘Bonwell’ plants can be grown for a complete production cycle under continuous lighting with LEDs without any photoperiod-related injury, this approach could also be tested with ‘Parys F1’ cultivar to reduce the light intensity/light capital costs.
Interestingly, none of the LED lamps tested here had a favorable effect on the production of tomato ‘Poranek’ seedlings compared to the FL control. The obtained plants were too long and flaccid for horticultural purposes. Even though LEDs can be very useful in plant production, not all species react positively to this light source (Miler et al., 2019). One should also remember the cultivar-specific responses of plants to lighting conditions. For example, specific light spectrum ratios induced different chemical composition responses in each cultivar of holy basil (Ocimum tenuiflorum L.) and at each developmental stage (Chutimanukul et al., 2022). Hence, other cultivars of tomato should also be studied. Moreover, other light spectra should be considered, e.g., emitting at least a small share of UV light, with more blue light and/or higher PPFD. Recently, farmers started a new trend in tomato cultivating with changing flavors by adding vitamin C and then introducing pink LED light. The variation from red to fuchsia pink light gave a vast difference in the flavor of the fruit (Nair & Dhoble, 2015). Monochromatic LEDs (blue, green, white) were tested in the cultivation of tomato ‘Cuty’ seedlings. Fresh weights of shoots and roots under LED treatment, especially red or green, were higher than those under the control FL light (Kim et al., 2014). Interestingly, tomato seedlings cultivated under higher percentages of blue light (up to 75%) had desirable characteristics such as shorter stem length and greater plant compactness (Hernández et al., 2016). Therefore, more studies are still needed on this species.
. Conclusions
Wide-spectrum LEDs AP673L, with 53% red, 17% far-red, 16% green, and 14% blue light, can be recommended to produce cucumber seedlings. The obtained plants are of good quality, comparable to those from the FL control. On the other hand, the tested LEDs are not suitable for tomato seedling production as they stimulate the growth of flaccid plants, although with a well-developed root system. As for this species, more research is needed to define the optimal light spectrum for optimal growth of aerial parts emitted by LEDs that would reduce production costs.