. Introduction
The genus Verbascum L. belongs to the family Scrophulariaceae and is commonly known as mullein. The genus Verbascum includes about 459 natural and hybrid species and is distributed mainly in Asia, Europe, and North America (Heywood, 1993; POWO, 2023). In Turkish flora, there are 253 natural species and 130 hybrid species belonging to it. The rate of endemism of the genus in Türkiye is about 80%, and 198 species were classified as endemic (Karavelioğulları et al., 2014). Verbascum scamandri Murb., known as “Kazdağı Mullein” and distributed in Kazdağı, Türkiye, is a biennial plant species, 50–80 cm high, with short and dense stellate hairs (tomentose) or glabrescent. The stem is cylindrical, long, and simple. Basal leaves greenish above, greyish, or yellowish tomentose beneath, 5–15 × 2.5–5 cm, leaf margin crenate, petiole 2–8 cm; cauline similar but smaller. The inflorescence is loose, simple, interrupted below, with flowers in clusters of 2–4 (Huber-Morath, 1978). V. scamandri is an endemic species to Türkiye and is classified as endangered (EN, B1-B2a) according to IUCN criteria (IUCN, 2012).
Studies on Verbascum species have identified a variety of secondary metabolites, including iridoid glycosides, phenylethanoid glycosides, flavonoids, saponins, monoterpenoid glucoside, neolignan glucosides, phenolic acids, steroids, and spermine alkaloids (Tatlı & Akdemir, 2004). In addition, the secondary metabolites of Verbascum species are known to be used in traditional medicine for the treatment of diseases such as respiratory tract diseases, eczema, tumor treatment, asthma, and migraine (Kozan et al., 2011; Turker & Camper, 2002). The presence of secondary metabolite compounds such as verbascoside, luteolin, and aucubin has been identified in many studies on the majority of Verbascum species (Abougazar et al., 2003; Akdemir et al., 2011; Dimitrova et al., 2012; Grabias & Swiatek, 1987; Hussain et al., 2009; Tatli et al., 2007; Zhao et al., 2011). According to recent studies, biological activities of verbascoside compounds in Verbascum nigrum, Verbascum phlomoides, and Verbascum thapsus species were found to exhibit antioxidant properties (Mihailović et al., 2016), and Verbascum mucronatum species was found to have wound healing activity, antinociceptive and anti-inflammatory potentials (Akdemir et al., 2011). When examining the biological activities of the compound aucubin, which was purified from Verbascum lasianthum, it was found to have antinociceptive and anti-inflammatory effects (Kupeli et al., 2007). When examining the biological activities of the compound luteolin, it was found that luteolin obtained from V. thapsus had antiproliferative effects (Zhao et al., 2011), Verbascum nubicum extracts exhibited hepatoprotective effects (El Gizawy et al., 2019), and Verbascum bugulifolium extracts had antioxidant, anti-inflammatory, and antimicrobial effects (Gökmen et al., 2021).
Plant tissue culture techniques are used in many commercial applications, such as haploid plant production, gene transfer, somatic hybridization, interspecific hybridization, synthetic seed production, disease-free plant production, secondary metabolite production, micropropagation, and conservation of genetically valuable plant species. Callus culture is one of the types of plant tissue culture, in which the formation of undifferentiated cells is induced by high amounts of cell divisions and proliferation resulting from the cultivation of plant explants in suitable nutrient media (Sokmen & Gurel, 2001). A number of studies have demonstrated that callus culture is an effective method for producing medically important compounds, indicating that it can be used to synthesize various valuable plant metabolites (Echeverri et al., 2019; Khurshid et al., 2020; Li et al., 2021; Purwianingsih et al., 2016).
Despite the traditional medicinal use and valuable secondary metabolites of Verbascum species, only a few in vitro studies have been reported. These include the establishment of an in vitro culture protocol for Verbascum thapsus (Turker et al., 2001); in vitro propagation of Verbascum letourneuxii (Farid et al., 2020) and Verbascum eriophorum (Yordanova et al., 2016); organogenesis and somatic embryogenesis studies in Verbascum speciosum and Verbascum sinuatum (Karamian & Ghasemlou, 2012, 2014); investigation of phenolic accumulation in Verbascum thapsus through callus culture (Al-Jibouri et al., 2016) and verbascoside production in V. thapsus using cell suspension culture (Temporiti et al., 2020); and exploration of secondary metabolite production in Verbascum eriophorum through Agrobacterium rhizogenes-mediated gene transfer (Marchev et al., 2016). However, there is currently no study on in vitro culture optimization in Verbascum scamandri. Therefore, the aims of our study were to optimize for the first time the conditions for the in vitro callus culture of Verbascumscamandri; to determine and compare the amounts of verbascoside, luteolin, and aucubin metabolites in calli samples, leaf samples of the in vivo collected plants, and leaf samples of in vitro growing plants.
. Material and methods
Plant material
Verbascum scamandri plants were collected from Bayramiç, Çanakkale, Türkiye (39°47′26.4″ N 26°53′48.2″ E and altitude of 625 m) during the flowering period in August 2021. The taxonomic identification was done according to the genus Verbascum L. in Flora of Turkey and the East Aegean Islands (Huber-Morath, 1978), and checked with reference collection in Çanakkale Botanic Garden Herbarium (CBB, Çanakkale, Türkiye) by Prof. Dr. Ersin KARABACAK from Çanakkale Onsekiz Mart University, Faculty of Science, Department of Biology. A voucher specimen was recorded in the CBB under the number CBB00002743. Rosette leaves were excised from these field-grown plants and washed with distilled water. The leaves were used for secondary metabolite analysis. Seeds were collected from these field-grown plants and used to obtain mother plants as the source of explants for the establishment of in vitro experiments.
Surface sterilization
Verbascum scamandri seeds were sterilized in 5% (v/v) sodium hypochlorite for 20 min, and the seeds were rinsed 4–5 times with sterile distilled water. The seeds were inoculated on Murashige and Skoog (MS; Murashige & Skoog, 1962) basal medium containing 3% (w/v) sucrose, 0.7% (w/v) phyto agar, and the medium was adjusted to pH 5.75 before autoclaving at 121 °C for 15 min. All cultures were kept in the growth chamber at 25 ± 2 °C under 16 h light/8 h dark photoperiod at a light intensity of 72 µmol m−2 s−1, 50 ± 5% humidity. Basal medium, sucrose, phyto agar, and plant growth regulators (PGRs) were obtained from Duchefa Biochemie (Haarlem, The Netherlands). The 14-week-old plants derived from in vitro germinated seeds were used as the source of explants for the establishment of callus culture. Also, the leaves of these plants were used for secondary metabolite analysis.
Callus induction
Leaf explants (5 × 5 mm) were inoculated on MS basal medium containing 3% (w/v) sucrose, 1 g/L polyvinylpyrrolidone (PVP, Sigma Aldrich), 0.7% (w/v) phyto agar, and supplemented with combinations of PGRs: 0, 0.1, 0.5, 1 mg/L auxin [Naphthaleneacetic acid (NAA) and 2,4-Dichlorophenoxyacetic acid (2,4-D)] and 0, 0.5, 1, 2, 3 mg/L cytokinin [6-Benzylaminopurine (BAP) or Kinetin (Kin)]. Five explants were cultured per petri dish, and at least three replicates were used for each treatment. After 3 weeks of culturing, callus induction rate, callus browning rate, texture, and color data were recorded for all treatments, and calculated using the following formulas:
After 3 weeks, the initial calli were subcultured on MS basal medium supplemented with the same PGRs as the callus induction media. The subculture was performed twice for callus development. During subcultures, various data were recorded, such as the biomass, diameter, texture, color of proliferated calli, and callus growth index was calculated using the following formula:
Secondary metabolite extraction and HPLC analysis
Leaf samples of the collected plants, leaf samples of in vitro growing plants, and calli samples were used for secondary metabolite analysis. Secondary metabolites were extracted using a modified method based on Çölgeçen et al. (2018). Leaf samples of the collected plants and in vitro growing plants were dried on blotting paper for 3–4 days at 22 ± 2 °C. Fresh weights were calculated from calli samples of the first and second subcultures. Dry weights were recorded after drying the biomass in an oven (Memmert INB 500) at 37 °C for 72 h. 0.1 g of each dried sample (leaf samples of the collected plants, leaf samples of in vitro growing plants, and calli samples) was powdered to a powder with liquid nitrogen and then mixed with the extraction solvent (10 mL of 99% Methanol) in an orbital shaker (WiseShake SHO-2D) at 22 ± 3 °C for 48 h. Then the samples were centrifuged at 8000 rpm for 20 min using a Hettich Universal 320 centrifuge. After centrifugation, the supernatants were separated and dried in a water bath (Daihan Scientific WB-11) at 40 °C for 1 day until MeOH evaporated. The final extracts were solubilized with 1 mL of 99% MeOH and filtered with Millipore filter (0.45 µm).
The secondary metabolites analyses of the samples were performed using high-performance liquid chromatography (HPLC) with diode-array detector (DAD, SPD-M20A). HPLC-DAD analysis was performed by a service provider at the Burdur Mehmet Akif Ersoy Scientific and Technology Application and Research Center. The standards were obtained from Sigma-Aldrich, and the standard curve was used with verbascoside, luteolin, and aucubin for quantification in the samples. HPLC-DAD analysis was performed using a Shimadzu Prominence device with a Zorbax C18 column (250 × 4.6 mm, 5 µm). Mobile phase A (3% formic acid) and mobile phase B (3% methanol) were delivered in a gradient method (3 min 93% A + 7% B, 28 min 72% A + 28% B, 60 min 67% A + 33% B, 62 min 58% A + 42% B, 70 min 50% A + 50% B, 75 min 30% A + 70% B, 90 min 93% A + 7% B with a flow rate of 0.8 mL/min and 50 µL sample injection modifying the methodology described by Caponio et al. (1999) and Janković et al. (2010). Detection wavelengths were set at 220, 230, and 240 nm. The secondary metabolite amounts of the samples were calculated in mg per 1 g dry weight. All the steps of our study have been summarized schematically in Figure 1.
. Results
Callus induction
To optimize the callus culture of V. scamandri, we evaluated the effects of various concentrations and combinations of cytokinin (BAP, Kin, 0, 0.5, 1, 2, 3 mg/L) and auxin (NAA, 2,4-D, 0, 0.1, 0.5, 1 mg/L). Callus induction occurred on leaf explants in 2–3 weeks of culture. The results of our study have shown that the use of BAP (0, 0.5, 1, 2, and 3 mg/L) and NAA (0, 0.1, 0.5, and 1 mg/L) alone or in combination resulted in the development of shoot in the calli, rather than remaining in an undifferentiated stage during the 3-week culture period. In addition, 1 g/L PVP was added to the media to prevent browning in the explants and calli. However, browning was observed in most treatments used for callus formation. The MS media supplemented with (1) 2 mg/L Kin, (2) 0.5 mg/L Kin + 0.5 mg/L 2,4-D, (3) 2 mg/L Kin + 0.5 mg/L 2,4-D, and (4) 3 mg/L Kin + 0.5 mg/L 2,4-D were selected for callus development according to the criteria of callus induction rate, suitable color, texture, and browning rate. Then, the in vitro culture experiment was carried out with these media. After 3 weeks of callus initiation, data on callus induction rate, texture, and color were recorded for each PGR treatment and are presented in Table 1. When comparing the concentrations and combinations of cytokinin and auxin, it was observed that adding the combination of Kin + 2,4-D to the MS medium resulted in 100 ± 0.00% stimulation of callus induction.
Table 1
Callus induction rate, browning rate, color and texture data of callus on induction media.
In our study, induced calli were subcultured twice in an MS basal medium containing the same concentrations and combinations of PGRs as the callus induction media (see above 1–4) at three-week intervals (Figure 2). Changes in color and texture of the calli samples were observed during the development of calli in subculture at the end of six weeks. Green and compact (Medium no 1), yellow-white and friable-compact (Medium no 2), yellow and compact (Medium no 3), or yellow and texture of friable-compact (Medium no 4) calli were observed during the culture (Figure 3). When the results of callus diameters in the first and second subcultures were evaluated, we determined that they ranged from 5.40 to 15.73 mm in MS medium containing 2 mg/L Kin (Medium no 1), from 5.19 to 7.85 mm in MS medium containing 0.5 mg/L Kin + 0.5 mg/L 2,4-D, (Medium no 2), from 4.61 to 8.93 mm in MS medium containing 2 mg/L Kin + 0.5 mg/L 2,4-D (Medium no 3), and from 3.58 to 8.85 mm in MS medium containing 3 mg/L Kin + 0.5 mg/L 2,4-D (Medium no 4) (Figure 4). The callus growth index was found to be 9.51 on the medium containing 2 mg/L Kin, 1.79 on the medium containing 0.5 mg/L Kin and 0.5 mg/L 2,4-D, 1.72 on the medium containing 2 mg/L Kin and 0.5 mg/L 2,4-D, and 1.42 on the medium containing 3 mg/L Kin and 0.5 mg/L 2,4-D (Figure 5). When we evaluated the data statistically, we determined that there was a significant increase in both callus diameter (15.73 ± 4.39 mm) and growth index (9.51), and the highest increase was observed in the culture medium containing 2 mg/L Kin (Medium no 1). The dry weight of calli was calculated, and 0.1 g was used from each sample.
Figure 2
Proliferation of calli in first (left sides) and second (rigt sides) subcultures in MS media containing (A) 2 mg/L Kin; (B) 0.5 mg/L Kin + 0.5 mg/L 2,4-D; (C) 2 mg/L Kin + 0.5 mg/L 2,4-D; (D) 3 mg/L Kin + 0.5 mg/L 2,4-D.
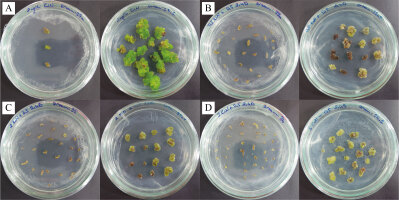
Figure 3
Texture and color appearance of proliferated calli. (A) Green and compact (2 mg/L Kin); (B) Yellow-white and friable-compact (0.5 mg/L Kin + 0.5 mg/L 2,4-D); (C) Yellow and compact (2 mg/L Kin + 0.5 mg/L 2,4-D); (D) Yellow and friable-compact (3 mg/L Kin + 0.5 mg/L 2,4-D) callus (bar = 5 mm).
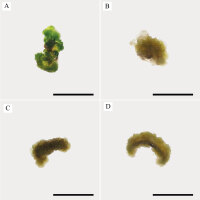
Figure 4
Measurements of the diameter (mm) of the proliferated calli in the first subculture and the second subculture on 0th and 21st day in MS basal medium supplemented with 2 mg/L Kin (1); 0.5 mg/L Kin + 0.5 mg/L 2,4-D (2); 2 mg/L Kin + 0.5 mg/L 2,4-D (3); 3 mg/L Kin + 0.5 mg/L 2,4-D (4). Means with the same letter in the columns are not significantly different at p ≤ 0.05.
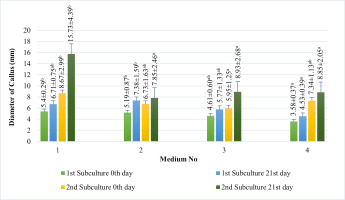
Figure 5
Callus growth index of proliferated calli in MS medium containing 2 mg/L Kin (1); 0.5 mg/L Kin + 0.5 mg/L 2,4-D (2); 2 mg/L Kin + 0.5 mg/L 2,4-D (3); 3 mg/L Kin + 0.5 mg/L 2,4-D (4) after the second subculture. Means with the same letter in the columns are not significantly different at p ≤ 0.05.
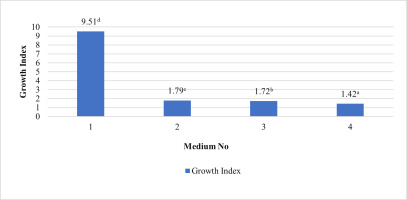
Secondary metabolite extraction and HPLC results
The amounts of verbascoside, luteolin, and aucubin compounds in calli obtained from tissue culture, leaf samples of in vitro growing plants and leaf samples of the collected plants were analyzed using HPLC (Figure 6). We also compared the levels of these secondary metabolites among the samples. According to HPLC results, when comparing the verbascoside, luteolin, and aucubin content of the callus with that of the leaf samples of the collected plants, all callus samples contained all three metabolites (Figure 7). According to the results, the verbascoside content in the leaf of collected plants was 7.03 mg/g, luteolin was 0.66 mg/g, and aucubin was 2.99 mg/g. The leaf of in vitro plants had 1.62 mg/g verbascoside, 0.18 mg/g luteolin, and 1.32 mg/g aucubin. On the other hand, we determined that the verbascoside content in callus tissues ranged from 1.05 to 13.77 mg/g, the luteolin content ranged from 0.25 to 0.51 mg/g, and the aucubin content ranged from 1.44 to 9.32 mg/g (Figure 7). In callus tissue, the content of these metabolites was dependent on the PGRs used. When we statistically evaluated the data, we found that the maximum content of the secondary metabolites in callus samples was 13.77 ± 0.00 mg/g of verbascoside in MS medium containing 2 mg/L Kin (Medium no 1), 0.51 ± 0.02 mg/g of luteolin in MS medium containing 2 mg/L Kin + 0.5 mg/L 2,4-D (Medium no 3), and 9.32 ± 0.34 mg/g of aucubin in MS medium containing 0.5 mg/L Kin + 0.5 mg/L 2,4-D (Medium no 2).
Figure 7
Amount of verbascoside, luteolin and aucubin compounds of leaf samples of the collected plants and leaf samples of in vitro growing plants and calli samples proliferated in MS medium containing 2 mg/L Kin (1); 0.5 mg/L Kin + 0.5 mg/L 2,4-D (2); 2 mg/L Kin + 0.5 mg/L 2,4-D (3); 3 mg/L Kin + 0.5 mg/L 2,4-D (4). Means with the same letter in the columns are not significantly different at p ≤ 0.05.
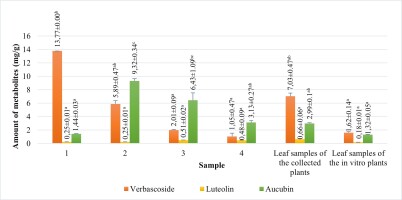
. Discussion
According to the results of the callus induction trials we conducted, it was observed that the use of BAP and NAA alone or in combination resulted in the differentiation of explants into shoots and meristemoids. However, Kin + 2,4-D combinations were found to be more effective for callus culture of Verbascum scamandri than BAP, NAA, or BAP + NAA combinations and stimulated more callus formation. In addition, an increase in auxin content promoted callus formation, and it was observed that developing calli did not differentiate into primordia in media containing the Kin + 2,4-D combinations. When evaluating the use of Kin alone and Kin + 2,4-D combinations, it was observed that media containing Kin + 2,4-D was more effective in the promotion of callus induction. Furthermore, calli obtained in these media were green or green-yellow in color and had a friable texture. Previous studies have shown that explants in media containing Kin have induced shoot formation in Verbascum speciosum Schard. and Verbascum thapsus species (Karamian & Ghasemlou, 2012; Turker et al., 2001). In the study on Matthiola incana, callus induction rates of 52.1% and 29.7% were achieved in media containing 0.5 and 1 mg/L Kin alone, respectively (Kaviani et al., 2013). Therefore, it has been observed that media combination auxin and cytokinin are more effective for callus initiation in V. scamandri. This is thought to be due to the co-stimulation and regulation of cell division by auxins and cytokinins. Studies have shown that the combined use of cytokinin and auxin is more effective than using them alone (Shirin et al., 2007; Yasmin et al., 2003). Since callus initiation occurs with equal amounts of exogenous and endogenous PGRs, it is thought that the ratios of auxin and cytokinin are balanced in media containing Kin concentrations (0.5, 2 and 3 mg/L) and 0.5 mg/L 2,4-D. In a study on Withania coagulans, the best callus formation was reported to be achieved in a medium containing Kin and 2,4-D (Valizadeh & Valizadeh, 2009). Similarly, the highest calli induction rates were obtained when 2,4-D (2 or 3 mg/L) and Kin (3 or 6 mg/L) were used combinations in different Citrus species (Hosseini et al., 2015). It is thought that the addition of auxin to the media during both the callus induction and proliferation phase positively affects the texture and color of the callus. Previous studies have reported that adding varying amounts of 2,4-D to the medium leads to the formation of friable, greenish, or yellowish callus (Najhah et al., 2021; Thiruvengadam et al., 2010; Zhang et al., 2011), which is also consistent with our results.
In our study, callus tissues were proliferated and developed through subcultures. It was found that the MS basal medium, containing PGRs in the same concentrations and combinations as the callus induction media was suitable for callus proliferation. Upon evaluating the results of the subculture, we observed an increase in callus diameter and growth index in all nutrient media; however, we determined that the greatest increase in terms of diameter and growth index occurred in the medium containing 2 mg/L Kin (15.73 ± 4.39 and 9.51, respectively). These results indicate the importance of subcultures for callus proliferation. In a study on Catharanthus roseus, callus biomass increased between 139% and 168% by subculture (Kaya & Aki, 2013). Similarly, in a study on Chlorophytum borivilianum, subculture was found to effectively increase callus biomass (Nakasha et al., 2016).
When comparing the metabolite amounts of leaf samples of the collected plants, leaf samples of in vitro growing plants, and calli samples, it was found that the calli in MS medium containing 2 mg/L Kin resulted in a higher verbascoside ratio, while the ratio of luteloin and aucubin increased more in the medium containing Kin + 2,4-D (respectively, 2 mg/L Kin + 0.5 mg/L 2,4-D and 0.5 mg/L Kin + 0.5 mg/L 2,4-D). When comparing the amounts of secondary metabolites, we found verbascoside content increased by 196% (Medium no 1), luteolin content increased by 77% (Medium no 3), and aucubin content increased by 312% (Medium no 2), in the callus culture compared to the leaf samples of the collected plants. We also found that the amounts of compounds in in vitro growing plant leaf samples were lower than in the leaf samples of the collected plants and calli samples. In our study, when comparing media containing PGRs, it was found that the MS basal medium with an addition of 2 mg/L Kin (Medium no 1) was the most suitable medium to produce verbascoside. We also determined that the MS basal medium with an addition of 2 mg/L Kin + 0.5 mg/L 2,4-D (Medium no 3) was the most suitable to produce luteolin, and the medium containing 0.5 mg/L Kin + 0.5 mg/L 2,4-D (Medium no 2) was found to be convenient to produce aucubin. Based on the results, it is thought that the concentrations of Kin and 2,4-D added to media impacted the amounts of secondary metabolites in the callus samples. According to Smetanska (2008), secondary metabolite production may be related to the differentiation processes of calli depending on PGRs, which led to the conclusion that the callus culture created in our study could be utilized for secondary metabolite production. Furthermore, it is thought that the proliferated calli of different textures and colors in these media influenced secondary metabolite accumulation.
Previous studies on the production of secondary metabolites from callus cultures have reported verbascoside amounts of 26.27–47.27 mg/g in callus extracts from V. thapsus species (Temporiti et al., 2020). In another study on the Verbena officinalis, verbascoside content of calli grown under different light spectra ranged from 2277 to 6716 mg/100 g dry weight (Kubica et al., 2020). The luteolin content in calli obtained from Capsicum chinense species was found to be 2.65 µg/g dry weight (Sherova et al., 2019). In another study, callus extracts from Globularia trichosantha subsp. trichosantha species were found to contain aucubin between 1.538–3.061 mg/kg and verbascoside between 0.018–0.742 mg/kg (Çölgeçen et al., 2018).
The verbascoside content in the leaf of collected plants was 7.03 mg/g, luteolin was 0.66 mg/g, and aucubin was 2.99 mg/g in V. scamandri. When secondary metabolite analyses of aerial parts, leaves of plants growing in the natural populations contained 13.1 ± 0.3 mg/g verbascoside in Verbascum sinuatum (Garcia-Oliveira et al., 2022); 73.85 mg/g verbascoside in Verbascum niveum (Grigorov et al., 2022); 16.77–48.46 mg/g verbascoside in V. thapsus (Temporiti et al., 2020). Luteolin was obtained 118.60 mg/g in Verbascum nigrum, 50.03 mg/g in V. phlomoides, and 63.36 mg/g in V. thapsus (Mihailović et al., 2016). In another study, the amount of aucubin was 15.88 ± 0.54 mg/g in the aerial parts of V. ovalifolium species (Luca et al., 2019). Comparing the results of our study with previous ones, it has been determined that the contents of verbascoside, luteolin, and aucubin obtained from the leaf samples of the collected plants were lower than those of other Verbascum species. Additionally, it was observed that the amounts of all three metabolites were lower in leaf samples of in vitro growing plants than in leaf samples of the collected plants, and calli samples.
This is the first report of in vitro callus cultures of V. scamandri and the determination and comparison of the amounts of verbascoside, luteolin, and aucubin metabolites in calli samples, leaf samples of the collected plants, and leaf samples of in vitro growing plants. An effective in vitrocallus protocol for secondary metabolites of V. scamandri was established as an output of our study. However, if additional research is done on the production these metabolites, elicitors might be used to enhance the production of secondary metabolites in V. scamandri.