Introduction
Entomopathogenic fungi in the subphylum Entomophthoromycotina (phylum Entomophthoromycota) (Spatafora et al., 2016) are well-known virulent pathogens infecting many species of arthropod hosts. The subphylum contains over 300 species, most of which belong to the order Entomophthorales, with nearly 290 described species (Hajek et al., 2018). Most of the entomophthoralean species are obligate pathogens of insects and other arthropods (Gryganskyi et al., 2012; Hajek et al., 2018). Likewise, they are relatively host specific and, thus, pose little or no threat to non-target organisms, making these fungi ideal biological control agents against insect pests. The host specificities of different entomophthoroid genera within the Entomophthorales are often limited to a given insect order or family; however, individual entomophthoroid species may show a higher level of specificity toward host genera or species (Humber, 2016). However, species or strains selected for commercialization as biological control agents cannot have too narrow a specificity spectrum to be economically profitable.
The identification of new strains to the species level is the first step in utilizing the full potential of fungi for specific applications (Lieckfeldt et al., 1999). Entomophthoralean species are identified on the basis of their host insect species and morphological features, primarily the size and shape of the primary conidia and the number of nuclei per conidium. However, in some cases, morphometric studies are insufficient to ascertain whether they represent a single taxon or complexes of morphologically similar species (Barta & Cagáň, 2006). For this purpose, methods based on either biochemical reactions or DNA sequences are used. Various molecular techniques have been used in the systematics of fungi to assess interspecific variation and determine phylogenetic relationships. Up to date molecular phylogenetic studies of Entomophthoromycotina have used molecular markers such as rDNA (i.e., 18S, 28S, or the whole ribosomal operon) and protein coding regions (e.g., actin, β tubulin, and RPB2). In addition, other more variable regions (e.g., ITS) have been used to study closely related taxa (Jensen et al., 2007). Different combinations of these have been used in multiple gene analysis (Gryganskyi et al., 2013). Today, the vast majority of available genomic information within the Entomophthoromycotina is composed of partial gene and intron sequences developed for use in phylogenetic analyses. Most sequences deposited in the National Center for Biotechnology Information (NCBI) GenBank database are from the nuclear ribosomal DNA region, including the large (LSU) and small (SSU) subunits, 5.8S, and internal transcribed spacer (ITS) regions 1 and 2. Only a few sequences of entomophthoralean fungi from Argentina are available in GenBank (Jensen et al., 2009; Scorsetti et al., 2011), reflecting the paucity of information on the diversity of this fungal group in the region.
The present paper reports on the identification of Entomophthora planchoniana Cornu, Pandora neoaphidis (Remaud. & Hennebert) Humber, Zoophthora phalloides A. Batko, and Zoophthora radicans (Bref.) A. Batko from Argentinian insects and provides both morphological and molecular characterizations of these species. The fungal pathogens Z. phalloides and Z. radicans from both Brevicoryne brassicae L. and from an unidentified species of Chironomidae (Diptera), respectively, are recorded for first time in Argentina.
Material and Methods
Specimens and Morphological Characterization
Specimens were collected during sampling from crops of economic importance in 2007–2013 (Table 1). The isolates examined in this study, their host taxon, plant host, collection site, date, and GenBank accession numbers are listed in Table 1. Zoophthora isolates were cultivated on Sabouraud dextrose agar plus 1% yeast extract (SDAY 1%), a suitable medium used for the isolation and culture of several species of Entomophthorales (Choi et al., 2016; Feng et al., 1990; Moubasher et al., 2010; Zhou et al., 2016). Pandora and Entomophthora specimens were identified directly from infected insect hosts. Specimens were examined under a stereomicroscope and an optical microscope for the presence of rhizoids, cystidia, and/or conidia.
Fungal structures were mounted in lactophenol-aceto-orcein (LPAO) (1:1) or stained with 1% aceto-orcein plus glycerine for semipermanent mounts (as preserved material for fungarium) and measured. Fungal species were identified according to taxonomic monographs and the keys of Bałazy (1993), Keller (2007), and Humber (2012). Photographs of the primary and capilliconidia of Z. phalloides and Z. radicans from in vitro cultures were taken with an Olympus BX51 camera (Japan) at ×400 magnification. Aphid host identification to the species level was made using the keys of Blackman and Eastop (2000). Lepidopteran hosts were identified by a taxonomic specialist at the Instituto Nacional de Tecnología Agropecuaria (INTA) (see Acknowledgments).
The specimens were preserved in the Mycological Collections at the Centro de Estudios Parasitológicos y de Vectores (CEPAVE, La Plata, Argentina). The isolates were deposited in the Mycological Collections at CEPAVE and the USDA-ARS Collection of Entomopathogenic Fungal Cultures (ARSEF) in Ithaca, New York.
Molecular Characterization: DNA Extraction and PCR Amplification
Fungus-infected insect cadavers were stored in 96% ethanol, and in vitro cultures were prepared as described by Jensen et al. (2001) until DNA extraction. Genomic DNA was extracted from infected insects (in vivo; for Entomophthora and Pandora specimens) or from in vitro cultures (for Zoophthora spp. specimens) via Chelex extraction (Traugott et al., 2008) or by using a DNeasy Plant Mini Kit (Qiagen, MD, USA) following the manufacturer’s protocol. PCR assays were performed on three loci, the internal transcribed spacer 1 region (ITS1), the 18S gene (SSU), and the first part of the 28S gene (LSU). Because of the low quantity of DNA obtained from infected insects, PCR assays of Entomophthora and Pandora specimens were limited to the ITS1 and LSU only. Universal fungal primers or entomophthoralean-specific primers were used to avoid amplification of insect host DNA. We used the universal fungal primers ITS5 (White et al., 1990) and the single reverse primer Nu-5.8S-3′ (Jensen & Eilenberg, 2001) the universal fungal primers nu-SSU-0021-58 (Gargas & DePriest, 1996) and nu-SSU-1780-38 (DePriest, 1993) for the SSU rDNA region, and nu-LSU-0018-5 (Jensen & Eilenberg, 2001) and LSU-0805 (Kjøller & Rosendahl, 2000) for LSU amplification.
The PCR conditions were: Initial denaturation for 30 seconds at 98 °C, followed by 38 cycles of denaturation for 10 seconds at 98 °C, annealing for 20 seconds at 55–60 °C (ITS1 60 °C, SSU 58 °C, LSU 55 °C), extension for 1 min at 72 °C, and a final extension for 10 min at 72 °C. The PCR reactions were carried out in 50 μL volumes, with 250 μM of each dNTP, 0.8 μM of each primer, 2.5 mM MgCl2, 1× buffer (10 mM Tris-HCl, pH 8.8 at 25 °C, 50 mM KCl, 0.1% Triton X-100), 1 unit of DyNazyme II (Finnzymes, Espoo, Finland), and 1 μL of extracted DNA (diluted 1:10). The size of the PCR amplification products was estimated by electrophoresis on a 1.5% agarose gel in 0.5× TBE, and the products were visualized with EZ-Vision (AMRESCO, USA). PCR products were purified using the QIAquick Purification kit (Qiagen, MD, USA) following the manufacturer’s protocol. Amplicon sizes were checked by electrophoresis, and purified PCR products were sent to Macrogen (Seoul, Korea) for sequencing in both directions. The sequences obtained were submitted to NCBI GenBank (https://www.ncbi.nlm.nih.gov/) for gene annotation. Sequences were edited using BioEdit version 7.0.9.0 (Hall, 1999) and were used to perform a phylogenetic analysis that included some sequences of related species in the genera Entomophthora, Pandora, and Zoophthora available at GenBank. Sequence data for each locus were aligned with the ClustalW tool of Mega5 (Tamura et al., 2011) and trimmed, as needed, using Mesquite version 3.0.2 (Maddison & Maddison, 2009). Aligned and trimmed sequence data were submitted to TreeBASE (http://purl.org/phylo/treebase/phylows/study/TB2:S27276?x-access-code=5af44733057f582185683187bae29cbf&format=html). Each dataset was analyzed using maximum parsimony (MP) in PAUP version 4.0a142 (Swofford, 2002) to determine the number of MP-informative sites. The phylogenetic analysis was carried out using the maximum likelihood (ML) method. The consensus tree was built using the latest 1,000 trees. Statistical support for the nodes was evaluated using 1,000 replicates. Phylogenetic analysis was limited to each locus; incomplete ITS1, LSU, and SSU sequence data from all specimens prevented presentation of a multilocus tree.
Table 1
List of species used in the study with information about insect and plant host taxa, location of collection, and GenBank accession numbers.
Results
Fungal Identification and Taxonomic Observations
Based on morphological characters, four fungal species were identified in the 17 insect specimens: E. planchoniana, P. neoaphidis, Z. phalloides, and Z. radicans (Table 1).
Entomophthora planchoniana Cornu
Bull. Soc. bot. Fr. 20: 189 (1873).
Description
Primary conidia campanulate, 14.2 ± 2.5 (12.1–16.8) × 12.1 ± 2.3 (9.8– 14.5) µm, with a small apiculus and broad, nearly flattened papilla, and multinuclear (three–five nuclei). Secondary conidia were observed emerging from the primary conidia and were slightly smaller: 11.2 ± 1.72 (9.7–13.5) × 10.5 ± 1.5 (8.7–12.2) µm (Figure 1E). Numerous thin rhizoids (Figure 1F). Cystidia and resting spores not observed. Attempts to obtain fungal isolates were not successful.
Pandora neoaphidis (Remaud. & Hennebert) Humber
Mycotaxon 34 (2): 452 (1989).
Description
Primary conidia ovoid or elongated, 23.55 ± 2.07 (19.6–27.3) × 12.08 ± 1.55 (9.6–14.3) µm, generally conical papilla connected gently with the body of the conidia Secondary conidia spherical or bell-shaped, 17.33 ± 1.36 (15.2–20.1) × 13.37 ± 1.26 (11.16–15.32) µm (Figure 1G). Capilliconidia absent. Rhizoids with discoid or irregularly branched adhesive disc. Cystidia present. No resting spores were observed. Attempts to obtain fungal isolates were not successful.
Zoophthora phalloides A. Batko
Acta Mycologica 2: 8 (1966).
Description
Primary conidia elongated cylindrical to elongated oval, 33.03 ± 4.89 (24.08–42.16) × 12.3 ± 2.52 (9.92–17.36) µm (Figure 1C). This characterization was in accordance with the original description (Batko, 1966).
The presently observed secondary conidia were capilliconidia: 18.40 ± 2.67 (12.4–22.32) × 7.04 ± 1.22 (4.96–9.92) × 94.63 ± 9.98 (79.36–114.08) µm (Figure 1D). Rhizoids were ramified and forming rhizomorphs. No resting spores were observed.
Zoophthora radicans (Bref.) A. Batko
Bull. Acad. Polon. Sci., Cl. II. sér. sci. biol. 12: 323 (1964).
Hosts
Acyrthosiphon pisum Harris, B. brassicae (Hemiptera: Aphididae), Plutella xylostella L. (Lepidoptera: Plutellidae), Tuta absoluta (Meyrick) (Lepidoptera: Gelechiidae), and an unidentified species of Chironomidae (Diptera).
Description
Elongated primary conidia uninucleate, 22.63 ± 3.40 (16.36–25.94) × 8.14 ± 0.75 (7.39–9.67), generally conical papilla demarcated with a slight protuberance from the body of the conidia (Figure 1A). Secondary conidia similar to primary ones or capilliconidia: 20.04 ± 1.83 (18.89–24.4) × 7.09 ± 0.54 (6.39–7.93) × 46.95 ± 9.70 (35.81–60.31) µm, fusiform and formed laterally on slender, capillary conidiophores arising from primary conidia (Figure 1B). Rhizoids mostly in pseudorhizomorphs, particularly abundant in the thoracical part, single or fasciculate with specialized adhesive discs. Conidiophores branched with terminal enlargement. Cystidia 3.69 µm in diameter, tapering uniformly at the base. No resting spores were observed.
Fungarium Accession Numbers
CEPHe67 (P. xylostella from Brassica napus L.), CEPHe68 (A. pisum from Medicago sativa L.), CEPHe69 (B. brassicae from B. oleracea var. botrytis), CEPHe70 (P. xylostella from B. napus), and CEPHe71 (P. xylostella from Brassica oleracea var. capitate L.).
Culture Collection Accession Numbers
CEP 30 (ARSEF 6917; T. absoluta from Solanum lycopersicum L.) and CEP 320 (ARSEF 8466; indet. Diptera from indet. host plant).
Figure 1
Zoophthora radicans from Tuta absoluta (Meyrick) primary conidium (A) and two capilliconidia each on a capilliconidiophore produced from a primary conidium (A,B). Bar: 10 µm. Zoophthora phalloides primary conidia (C) and capilliconidia on capilliconidiophore from primary conidium (D). Bar: 10 µm. Entomophthora planchoniana from Myzus persicae (Sulzer) primary and secondary conidia (E). Bar: 20 µm. Rhizoids of Entomophthora planchoniana from Myzus persicae (Sulzer) (F). Bar: 1 mm. Pandora neoaphidis from Nasonovia ribisnigri (Mosley) primary and secondary conidia (G). Bar: 10 µm.
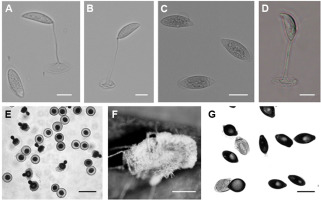
Molecular Characterization: DNA Sequencing and Molecular Analyses
We successfully amplified DNA samples and obtained sequences of the ITS1 (337 bp for Z. radicans, 259 bp for E. planchoniana, and 308 bp for P. neoaphidis), LSU (755 bp for Z. radicans, 855 bp for P. neoaphidis, and 820 bp for E. planchoniana), and SSU (1,720 bp for Z. radicans and 1,739 bp for Z. phalloides). All sequences were deposited in GenBank under the accession numbers given in Table 1.
Combined with the GenBank sequences of other entomophthoralean fungi, PAUP analysis showed: ITS1 sequence data (total 733 bp) with 141 variable parsimony-uninformative and 541 parsimony-informative characters, LSU sequence data (total 1,024 bp) with 165 variable parsimony-uninformative and 341 parsimony-informative characters, and SSU sequence data (total 1,863 bp) with 207 variable parsimony-uninformative and 54 parsimony-informative characters.
The best tree from the ML analysis of the ITS1 sequences showed that our isolates of E. planchoniana and P. neoaphidis clustered with the same respective entomophthoralean species, with a 67% bootstrap value (Figure 2). All E. planchoniana specimens had the same ITS1 sequence. The ITS1 sequence of P. neoaphidis CEPHe73 clustered with P. neoaphidis ARSEF 835 in another monophyletic group supported by a 99% bootstrap value. Finally, the Z. radicans CEPHe67, CEPHe68, CEPHe69, CEPHe70, CEPHe71, and CEP 30 sequences clustered with Z. radicans NW386 in another monophyletic group supported by a 100% bootstrap value. The ITS sequences of the Z. radicans specimens did not reveal differences between different hosts.
Figure 2
Phylogenetic relationships of Zoophthora, Pandora, and Entomophthora and related species inferred from maximum likelihood analysis of ITS1 sequences. The sequences corresponding to this work are marked with asterisks. Bootstrap values are noted above the internodes. The bar at the bottom indicates the number of substitutions per site.
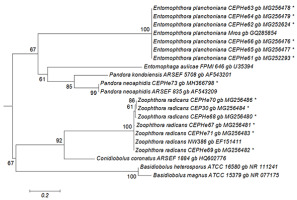
In the phylogenetic analyses of the LSU rRNA, P. neoaphidis and Z. radicans were clustered in a monophyletic group with sequences from related entomophthoralean species. This grouping was supported by a 100% bootstrap value. The LSU sequences of six Z. radicans specimens from Diptera, Hemiptera, and Lepidoptera were identical to that of ARSEF 388, which was isolated from a dipteran in Switzerland. Zoophthora radicans specimen 320 had 99% similarity with the group (Figure 3). Entomophthora planchoniana specimen CEPHe64 clustered with E. planchoniana ARSEF 6252 in another monophyletic group, which was supported by a 100% bootstrap value (Figure 3).
Figure 3
Phylogenetic relationships of Zoophthora, Pandora, and Entomophthora and related species inferred from maximum likelihood analysis of LSU rDNA sequences. The sequences corresponding to this work are marked with asterisks. Bootstrap values are noted above the internodes. The bar at the bottom indicates the number of substitutions per site.
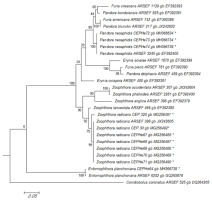
In the ML tree from the phylogenetic analysis of the SSU rRNA sequences, Z. radicans CEPHe67, CEPHe68, CEPHe69, and CEPHe71 were clustered in a monophyletic group supported by a 65% bootstrap value, which includes representatives of Z. radicans (Z. radicans ARSEF 853) and Z. lanceolata (Z. lanceolata ARSEF 469) (Figure 4). Based on the SSU rRNA sequences, Z. phalloides CEP 687 clustered with representatives of Z. anglica, Z. phalloides, and Z. occidentalis in another monophyletic group supported by a 99% bootstrap value. Likewise, the sequence of Z. phalloides CEP 687 characterized in this work was clustered with Z. phalloides ARSEF 2281 in a same clade supported by a 100% bootstrap value (Figure 4).
Figure 4
Phylogenetic relationships of Zoophthora and related species inferred from maximum likelihood analysis of SSU rDNA sequences. The sequences corresponding to this work are marked with asterisks. Bootstrap values are noted above the internodes. The bar at the bottom indicates the number of substitutions per site.
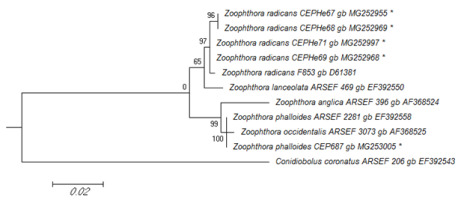
Discussion
In the present study, the initial identification of fungi from various insect hosts was based mainly on the shape and size of the primary and secondary conidia and according to host affinity. These identifications were confirmed by use of molecular data, specifically the DNA sequences of the ITS1, LSU, and SSU. Based on the results obtained in this study, the morphology of the secondary conidia of P. neoaphidis was consistent with the original descriptions reported by Humber (1989). Our results were also consistent with observations made by Scorsetti et al. (2006). These authors previously reported P. neoaphidis from aphids in Argentina, with measurements of both primary and secondary conidia very similar to those observed in this study. With regards to its insect hosts, P. neoaphidis is a common aphid pathogen with a cosmopolitan distribution. In Argentina, it was previously recorded in 17 aphid species (López Lastra & Scorsetti, 2006; López Lastra et al., 2019; Manfrino, Hatting, et al., 2014; Manfrino et al., 2013; Scorsetti et al., 2006) and was reported by Scorsetti et al. (2006) as the most predominant pathogen of aphids in Argentina.
We identified six specimens of Entomophthora from aphids as E. planchoniana. The identity of the fungal species was determined based on insect host, number/size of conidial nuclei, and conidial size (Humber, 2012). Compared to the original description of E. planchoniana (Keller, 1991), the materials observed in this study had slightly smaller conidia than those originally described. The results obtained in this study, however, were consistent with those made by López Lastra and Scorsetti (2006) and Scorsetti et al. (2006). These authors previously recorded E. planchoniana as a pathogen of N. ribisnigri, Aphis fabae, Aphis gossypii, Myzus sp., and M. persicae in Argentina and observed that the primary conidia from their specimens were slightly smaller than those described by Keller (1991). For many years, E. chromaphidis was considered to be a synonym for E. planchoniana (Gustafsson, 1965; MacLeod et al., 1976; Waterhouse & Brady, 1982) until these two species were definitely separated and justified (Humber & Feng, 1991). Entomophthora chromaphidis occurs in North America and Australia and is characterized as having smaller primary conidia and larger nuclei, whereas E. planchoniana is more widely distributed (primarily in Europe) and has larger primary conidia and smaller nuclei. In Argentina, E. planchoniana has been recorded as an aphid pathogen on horticultural crops (López Lastra & Scorsetti, 2006; López Lastra et al., 2019; Scorsetti et al., 2006). It has also been recorded causing epizootics in M. persicae on pepper and eggplant crops (Manfrino et al., 2016).
Regarding its host affinity, previous studies have already covered Entomophthora species. A study elucidated the host-driven divergence of species within the E. muscae (Cohn) Fresen. species complex and E. planchoniana Cornu (Jensen et al., 2009). Thomsen and Jensen (2002) showed a separation of resting spore isolates of E. muscae species complex at the species level, which is not possible using only morphological characters (i.e., diameter). Freimoser et al. (2001) and Jensen et al. (2009) showed that isolates originating from different specimens of the same host taxa appeared to be strongly clonal, even when they were sampled at different localities in different years. In agreement with these observations, our study showed only minor differences in E. planchoniana specimens from several different aphid species based on ITS1 sequences.
In this study, the primary and secondary conidia of Zoophthora radicans specimens were slightly bigger than the original description reported by Keller (1991). Our results are consistent with reports by López Lastra and Scorsetti (2006) regarding the measurements of the primary conidia of Z. radicans from T. absoluta, although we observed bigger capilliconidia. Scorsetti et al. (2006) recorded Z. radicans from six aphid species, and our measurements of the primary and secondary conidia of this species are consistent with their data. Additionally, these authors recorded Zoophthora sp. from only one aphid species and found that its morphological characteristics clearly differed from those of Z. radicans. In this study, measurements of Zoophthora from B. brassicae were different from those of Z. radicans, with primary and secondary conidia of greater values. Based on the original description by Batko (1966), this species corresponds to Z. phalloides. Glare et al. (1987) studied different criteria based on morphological, physiological, and biochemical characters in order to reliably differentiate Z. phalloides from Z. radicans. This allowed the division of isolates broadly defined as Z. radicans and Z. phalloides using shape and spore dimensions, host specificity, growth in vitro, and analyses of isoenzymes and fatty acid composition (Glare et al., 1987). Balazy (1993) conducted thorough studies on the morphology, biology, and particularly host specificity of the genus Zoophthora, which enabled him to distinguish and describe seven new species morphologically similar to Z. radicans. Zoophthora phalloides is very similar morphologically to other aphid pathogenic members of the Zoophthora spp. complex. Zoophthora phalloides was first described as a pathogen of aphids (Hemiptera) in Poland by Batko (1966) and has since been recorded in North America, Britain, Denmark, France, Switzerland, Israel, Korea, New Zealand, and occasionally in Australia (e.g., Barta & Cagáň, 2005; Nielsen et al., 2001; Papierok et al., 2016; Yoon et al., 1998), thus indicating a worldwide distribution.
Zoophthora radicans has the broadest host range reported for any species of the Entomophthoraceae. This observation has led some authors to suggest that it is a complex of several species differing in their pathogenicity to different hosts (Bałazy, 1986; Glare, 1988; Humber, 1983; McGuire et al., 1987). It has been collected worldwide from hosts in the insect orders Lepidoptera, Diptera, Coleoptera, Orthoptera, Thysanoptera, Hemiptera, and Hymenoptera (Glare & Milner, 1991; Keller, 1991; Milner & Soper, 1981; Papierok et al., 1984; Soper & Ward, 1981). In Argentina, Z. radicans has been reported as a pathogen of several insect species (Manfrino, Hatting, et al., 2014; Manfrino et al.,2013; Manfrino, Zumoffen, et al., 2014; Scorsetti et al., 2006), but there were no records of dipteran hosts until this study. Milner and Mahon (1985) identified Z. radicans in other species of Diptera (Damaromyia sp., Sciaridae, Psychodidae) in Australia. Our results revealed that the ITS1, LSU, and SSU sequences of Z. radicans specimens from Diptera, Lepidoptera, and Hemiptera show few differences. In analyses of LSU sequences, all specimens clustered as Z. radicans, with a slight difference for the dipteran isolate, in agreement with reports of its wide host range.
For species included within a species complex, molecular characters are critical data supplementing analysis at different taxa. In this study, species of Zoophthora in Argentina were characterized by molecular tools for the first time. Molecular analyses based on SSU rDNA sequences confirmed the identity of Z. phalloides, with the Argentinean strain grouped with Z. phalloides strain ARSEF 2281 (gb EF392558) with a 100% bootstrap value. Likewise, with regards to host affinity, the Zoophthora aphid-pathogenic isolate CEP 687 was clustered with isolate ARSEF 2281 (Z. phalloides), which was originally isolated from B. brassicae, in accordance with the host aphid species of our native strain (Figure 4).
This study reports the first record of Z. phalloides as a pathogen of B. brassicae and Z. radicans as a pathogen of an unidentified species of Chironomidae (Diptera) in Argentina. The identification and establishment of in vitro cultures of these fungal species would allow further studies determining their potential as microbial biological control agents for the management of insect pests of agricultural crops. Of the four fungi, Zoophthora spp. and Pandora neoaphidis can be grown on SDAY, but Zoophthora spp. are easier to cultivate than P. neoaphidis on this medium. Other entomophthoralean species included in the genus Entomophthora require complex and highly nutritious culture media, which is considered a sign of high specialization and close adaptation to the host (Humber, 1994). Based on these observations, Zoophthora strains would have greater potential to be used as targeted microbial control agents.
Handling Editor
Malgorzata Ruszkiewicz-Michalska; Institute for Agricultural and Forest Environment, Polish Academy of Sciences; University of Łódź; https://orcid.org/0000-0001-8901-0552