. Introduction
Aboveground plant parts are normally colonized by various parasitic bacteria, yeasts, and fungi that gain access to host resources required for survival, growth, and reproduction (Agrios, 2009; Leach et al., 2014). Parasitism is typically categorized as a trophic interaction since a parasite utilizes host’s nutrients (Knudsen & Dandurand, 2014). Plant pathogens cause diseases of the leaf, stem, root, vascular system, and fruit. The damage may be moderate or very severe, sometimes destroying all the plants or resulting in the extinction of entire plant species in other cases (Agrios, 2009; Al-Ani & Furtado, 2020; Chlebicki, 2004). To avoid disease, plants must recognize the presence of potential pathogens and launch a sufficiently strong defense response restricting pathogen attack without a detrimental effect on the plant’s physiology (Clay et al., 2005; Endara et al., 2017; Leach et al., 2014). Phyllospheric microorganisms stimulate the production of plant defensive chemicals killing pathogenic organisms, i.e., phytoalexins (terpenoids, glycosteroids and alkaloids) produced rapidly at sites of pathogen infection (Chlebicki, 2004; Karki, 2019; Remus-Emsermann & Schlechter, 2018). Interdependence between plants and their enemies, referred to as a co-evolutionary arms race, is an important element of the life history of both groups. Parasitic and mutualistic associations play a key role in co-evolutionary interactions in population and community ecology. Parasites, which change constantly and are actively involved in the arms race against their hosts, somehow enforce genetic variation in the hosts. Genetic diversity is, therefore, the host’s defense against parasites (Chlebicki, 2004; Harper, 1977; Maron et al., 2019; Poisot et al., 2011; Thrall et al., 2007; Wininger & Rank, 2017).
Aerial photosynthetic tissues of plants, also known as the phyllosphere, form a large and extremely diverse habitat for terrestrial microorganisms. The phyllosphere and rhizosphere are unique and wide-ranging habitats for a variety of microbial communities influencing plant growth and health, plant population size, and the productivity of ecosystems (Al-Ani & Furtado, 2020; Colling & Matthies, 2004; Harper, 1977; Laforest-Lapointe & Whitaker, 2019; Leach et al., 2014; Lindow & Brandl, 2003; Stone et al., 2018; Thrall et al., 2007).
The greatest interest in phyllosphere microbiology has been placed on leaves, i.e., the more dominant aboveground plant structure. The surface area of the phyllosphere is suggested to be approximately twice as great as the land surface area and provides a habitat for numerous microorganisms, which colonize the surfaces (where they mostly form aggregates) and the inside of leaves (Morris et al., 2002; Vorholt, 2012). Most studies on the identity of phyllosphere organisms have focused on bacteria and, to a lesser extent, on fungi (Vorholt, 2012). As demonstrated by this author, fungal communities are extremely variable in the phyllosphere in temperate regions and exhibit even greater diversity than in the phyllosphere of tropical trees. Phyllospheric microorganisms colonize the leaf surface and first degrade the wax and cuticle layer and damage the leaf (e.g., Karki, 2019; Leach et al., 2014; Remus-Emsermann & Schlechter, 2018; Stone et al., 2018). Various environmental factors and other abiotic and biotic conditions can affect populations of pathogens and are influenced by the physical structure of the plant community (height, density). The vegetation cover has an impact on the microclimate, including light, temperature, and humidity. The most important factors include light (also UV), cold, moisture, various chemicals produced by pathogenic and other phyllospheric microorganisms on the leaf, leaf position, leaf surface, tissue damage, nutrient content per leaf area, etc. (Karki, 2019; Neumann et al., 2004; Perez et al., 2009; Vorholt, 2012).
In general, the spread of fungal pathogens is passive (Money, 2016); the movement of spores is determined by the agent of transport, e.g., wind, water, or animals. The efficiency of this “disease escape in space” (Harper, 1977) depends on distances between individual plants within the population. Thus, the main spread is possible between plants that grow close or make physical contact with each other, i.e., in crowded or sometimes overcrowded populations. Most pathogenic fungi have also dormant resting stages, and they may develop spores that await more advantageous conditions and survive for many years, e.g., in the soil, which is called “disease escape in time” (Harper, 1977). Some of them may also use many different plant species as hosts. This phenomenon, referred to as “host jumping”, occurs from typical (susceptible) hosts to “non-host” plants through asymptomatic tissue infection and subsequent exploration of new susceptible hosts (Crous et al., 2004; Verkley & Starink-Willemse, 2004; Verkley et al., 2013). For these reasons, a pathogen may not be found on a host plant species even in successive seasons.
One of the biotic factors that limit the growth, size, and reproductive abilities of the Senecio umbrosus Waldst. et Kit. population is the exceptionally quick spread of microscopic fungi, first of all living in the phyllosphere. Fungal pathogens have been observed on this ragwort species within the White Mt. population since the early 2000s, and the preliminary collection was made in 2008 (Czarnecka, 2009). In some growing seasons, the plant species even did not produce valuable propagules in natural conditions and an experimental garden due to the massive appearance of microfungi (B. Czarnecka, unpbl. data). The present study aimed to determine: (i) the spectrum of microfungi inhabiting the leaf surface of the ragwort S. umbrosus; (ii) the degree of leaf infection in different periods of growing season; (iii) the repeatability of the occurrence of microfungal species in successive growing seasons.
. Material and methods
Study site
The studies were conducted on Biała Góra (White Mountain) near Tomaszów Lubelski, Central Roztocze Highlands (Figure 1). White Mt. (50°28′N, 23°29′E; 349 m a.s.l.) is a vast parabolic hill built of Cretaceous marls and loesses. Shallow rendzinas were formed on this substratum with the alkaline soil reaction (pH = 7.4–7.8). The southwestern, western, and northwestern slopes with a varied gradient (10–45°) are overgrown with mosaic vegetation (Czarnecka, 1995, 2009, 2010, 2020). Open areas are inhabited by xerothermic grasslands of the alliance Cirsio-Brachypodion pinnati, the class Festuco-Brometea, which represent patches of both low and loose, and tall and dense grasslands. They are surrounded by xerothermic bushes of the class Rhamno-Prunetea, accompanied by communities of tall herbs belonging to the class Trifolio-Geranietea sanquinei. The top parts of the hill are covered by beech-hornbeam forests of the class Querco-Fagetea with an admixture of Pinus sylvestris L. (the classification of plant syntaxa is given after Matuszkiewicz, 2008, and the names of plants follow Mirek et al., 2002).
Study plant species
One of the most valuable elements of the flora occurring on White Mt., often subjected to strict or partial protection in Poland, is the ragwort species S. umbrosus (Calvo & Aedo, 2015), Asteraceae. The species (Figure 2) was documented in the study site in the early 1980s (Karczmarz & Sałata, 1984). It was formerly known as S. doria subsp. umbrosus (Chater & Walters, 1976), S. umbrosus (Czarnecka, 1995; Karczmarz & Sałata, 1984), and S. macrophyllus (Czarnecka, 2006, 2009, 2010; Czarnecka & Denisow, 2014; Czarnecka & Kucharczyk, 2014; Fijałkowski, 1980; Grulich & Hodálova, 1994; and others). The ragwort has a rather narrow ecological niche occurring in xerothermic grasslands, thermophilous shrubs, and loose forests or small islets within calcareous meadows and fens. A common feature of the substrate, regardless of its composition and humidity, is the high CaCO3 content and neutral or alkaline reaction. S. umbrosus is provided the best conditions for growth and development in well-lit places with temporary or periodic shade (Zarzycki et al., 2002). Because of the small number of localities and small areas of occurrence, it was included into the Polish red list and red data book of plants with vulnerable category of threat (Czarnecka & Kucharczyk, 2014; Kaźmierczakowa, 2016).
Figure 2
Senecio umbrosus in xerothermic grassland on White Mt.: (A) vegetative phase; (B) generative phase. Photography by A. Wołczańska (A) and B. Czarnecka (B).
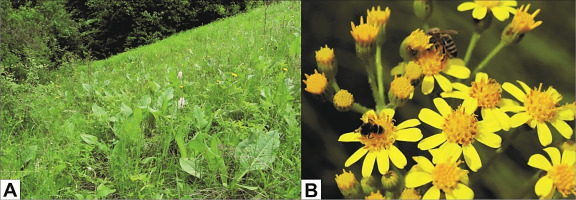
The species is characterized by dominance of generative reproduction, a very slow rate of individual development, and a long life span of the genet. Depending on the growing season, the ragwort begins the vegetation phase in the 2nd or 3rd ten days of April. In full season, the leaf rosette of vegetative individuals has from 2 to 10 leaves, which are 20–50 cm long or exceptionally even 60–70 cm; their width is in the range of 5.5–20 cm or sometimes slightly more. Only ca. 2/3 of plants in the generative phase form a rosette of 1–3 leaves (max. 5–6) with a similar size to those in vegetative or plants resting between successive generative episodes. The plant flowers in July–August and produces an erect flowering stem, mostly 80–120 cm or sometimes up to 150–190 cm high, and an inflorescence (subcorymb) consisting of numerous heads. The phase of dispersal of earliest mature diaspores, i.e., achenes equipped with pappus, begins at the end of flowering, sometimes around mid-August, and lasts long until October–November, depending on the microhabitat and weather conditions. Usually, at the end of fruiting and the beginning of diaspore dispersal, the stem and basal leaves dry up in generative individuals, while the leaves of vegetative rosettes dry up gradually until the end of the growing season (Czarnecka, 1995, 2006, 2009, 2010; Czarnecka & Denisow, 2014; B. Czarnecka, unpbl. data).
Microfungal data collection
Eight permanent plots, i.e., 1 m2 patches (measurement centers) were established in June 2014 on the xerothermic grassland inhabited by S. umbrosus in the belt of 265–315 m a.s.l. Among these patches varying in light conditions (exposed more toward the south or west), species composition, and herb layer density, there were five patches of low, loose, and sun-exposed grassland dominated by Aster amellus L., Carex flacca Schreb., Linum flavum L., Teucrium chamaedrys L., and Salvia verticillata L. and three patches of higher and denser grassland with Brachypodium pinnatum (L.) P. Beauv. and Peucedanum cervaria (L.) Lapeyr. as dominants, situated on the edge and moderately shaded by brushwood and forest. The study was conducted during three vegetation seasons (2014–2016) in order to note the sequence of occurrence of phytopathogens: in late spring (first ten days of June), summer (last ten days of July, first ten days of August), early autumn (second ten days of September), and autumn (first ten days of October); six investigation series were performed altogether.
Leaves of S. umbrosus plants exhibiting disease symptoms or signs of microfungal infections (necrosis, distortions, visible discolorations or deformations, and etiological signs, i.e., heaps of spores and/or sclerotia blackish, brown, and rusty in color, greyish-white tint, etc.) were collected within a radius of 2 m from the central point of each permanent 1 m2 plot, transferred to the laboratory, and dried. The infected plants were examined by means of a standard light microscope Olympus CH30 and a scanning electron microscope (SEM) VEGA3 Tescan. Observations and measurements of spores and other fungal structures were made in slide preparations stained with cotton blue in lactic acid and heated. The identifications of fungi and their nomenclature are consistent with those proposed by various authors (Brandenburger, 1985; Braun, 1995, 1998; Crous & Braun, 2003; Ellis & Ellis, 1987; Heuchert et al., 2005; Majewski, 1977, 1979; Wołczańska, 2005, 2013). All plant samples were deposited in the Herbarium of the Department of Botany, Mycology and Ecology, Faculty of Biology and Biotechnology, Maria Curie-Skłodowska University in Lublin (LBL).
The frequency of particular species of microfungi was calculated as a percentage of leaves collected in a particular investigation series. Additionally, the degree of leaf infection was analyzed in leaves collected during three investigation series conducted in 2014, both on the abaxial (lower) side and adaxial (upper) side. The percentage share of fungal infection was calculated using the ArcMap 10.1 program. Seasonal occurrences of microfungi on S. umbrosus in 2014–2016 were presented versus the meteorological conditions prevailing in the vegetation period of the ragwort (April–October) in relation to long-term means (1951–2016). The data were provided by the Tomaszów Lubelski station of the Institute of Meteorology and Water Management (IMWM, 2019). The following data were used: the mean month temperature and precipitation (in mm) with the deviation of these values from the long-term mean, the number of days with precipitation, and the number of days with temperature higher than 5 °C in a given month.
. Results
Microfungal numbers and diversity
During the three growing seasons (six collection series between May and October), 15 species of phytopathogenic microfungi and three hyperparasites were collected on S. umbrosus leaves in total (1–9 species per one collection date, 11–12 species per season). It was, respectively, nine and two species in 2014, nine and three species in 2015, and nine and two species in 2016. The collection comprised 14 Ascomycota and only one Basidiomycota species (Table 1).
Table 1
List of microfungal species collected on Senecio umbrosus leaves in 2014–2016; +/− – presence or absence of species.
Only C. tussilaginis (Figure 3) was present in all six study. The other species were collected in five (Boeremia exigua, Leptosphaeria ogilviensis), four (Alternaria sp.), or three investigation series (Ascochyta compositarum, Periconia cookei), while the other taxa were found twice (Asteromella senecionis-nemorensis, Epicoccum nigrum, Golovinomyces cichoracearum) or only once (Acremonium sp., Alternaria tenuissima, Cladosporium herbarum, Cladosporium sp., Didymella senecionicola, Phyllosticta sp.). Hyperparasites on C. tussilaginis were represented by Cladosporium uredinicola, Ramularia coleosporii, and Alternaria sp. (Table 1).
Figure 3
Coleosporium tussilaginis on Senecio umbrosus leaves: (A) general view of infected plant; (B) abaxial (lower) side of leaf. Photography by A. Wołczańska.
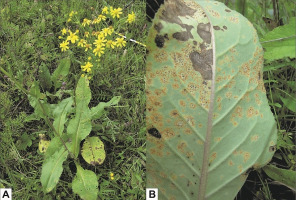
The majority of fungal taxa identified on S. umbrosus are common species in Poland, but some of them deserve special attention, particularly a new species for Poland – A. compositarum. Its leaf spots are roundish or elliptical, brownish, sized 5–10 × 4–7 mm. The pycnidia are epiphyllous, scattered, pale brown, up to 130 µm diam., with a circular ostiolum, 40–44 µm diam. The pycnidial wall is thin. Cylindrical and usually uniseptate conidia, sometimes with an eccentric septum, very rarely have two septa: 14–20(–22) × 4–5 µm (Figure 4). In turn, the C. uredinicola (Figure 5) and R. coleosporii (Figure 6) hyperparasites represent a group of rare species in Poland.
Seasonal dynamics of microfungal occurrence
The dominant pathogen in all years, regardless of the collection date, was C. tussilaginis (Figure 3). The share of this species in the number of records of the microfungi (elaborated on the basis of 180 collected leaves) ranged from ca. 30 to 100%. In turn, the next most frequent species i.e., B. exigua and L. ogilviensis, represented 21–30% of the occurrences only in some collection series (Table 2). The massive occurrence of C. tussilaginis was partially limited by the hyperparasites growing in summer, in particular, R. coleosporii. The rate of leaf infection increased during the growing season and was much higher in the case of the abaxial (lower) side than the adaxial (upper) side (Table 3, Figure 3, Figure 7).
Table 2
Frequency of microfungal species on Senecio umbrosus (%) in the total number of records at subsequent collection series: ∙≤ 10%; ● 11–20%; ⬤ 21–30%; 31–40%;
41–50%;
> 50%.
Table 3
Degree of leaf infection by the most abundant microfungal species during vegetation period of Senecio umbrosus in 2014.
Occurrence of microfungi vs. weather conditions
The number of species of phytopathogenic microfungi on S. umbrosus leaves also increased in the successive growth phases reached by the host plant: flowering, fructification, and dissemination. The largest numbers of pathogen species were found in the summer of 2016 and in the early autumn of 2014 (nine each time). Slightly higher numbers of fungal species were found in open microhabitats with western exposure (Table 1, Table 2, Table 4).
During the study period, the mean annual air temperature and the annual precipitation sum clearly differed from the long-term means for 1951–2016 (7.2 °C and 636.8 mm, respectively).
The year 2014, when nine species of parasites and two species of hyperparasites were collected on three dates, was characterized by relatively the lowest deviations from the long-term means (air temperature 8.5 °C, precipitation sum 750.5 mm). In turn, 2015 was the warmest year since 1951 (9.3 °C) and drier than usual (precipitation sum 601.5 mm). In 2016, both the mean annual air temperature and the precipitation sum were slightly higher than in 2014 (8.7 °C and 780.6 mm, respectively). During the two last study seasons, nine parasites and three species of hyperparasites (two collection series), as well as nine parasites and two hyperparasite species (only one collection series), respectively, were found (Figure 8).
. Discussion
Changes in the growth, abundance, and generative reproduction in a plant population are caused by both abiotic and biotic factors that influence plants in different ways. Among the biotic factors, a specific role is played by a variety of parasitic microorganisms living in the phyllosphere, i.e., a more dominant aerial plant structure (Agrios, 2009; Al-Ani & Furtado, 2020; Knudsen & Dandurand, 2014; Leach et al., 2014; Vorholt, 2012). They exploit host plant resources, thereby influencing plant growth and health, reproduction ability, population size, and, finally, the productivity of the ecosystems (Al-Ani & Furtado, 2020; Colling & Matthies, 2004; Harper, 1977; Lindow & Brandl, 2003; Stone et al., 2018; Thrall et al., 2007). Phyllosphere traits (liverworts, mosses, lichens, bacteria, fungi, and other microorganisms colonizing leaves) are suggested to be fundamental traits linked with such aboveground trait axes as the photosynthetic strategy, hydraulics, resource acquisition, leaf temperature, and reproduction (Rosado et al., 2018 and literature cited therein).
Table 4
Occurrence of microfungi on Senecio umbrosus in 2014 according to its phenology and microhabitats: v – vegetation (late spring); f/f – flowering/fructification (summer); d/w – dissemination/withering (early autumn); +/− – presence or absence of species. Species noted on: ∙ 1–2 plots; ● 3–4 plots; ⬤ 5–6 plots; 7–8 plots.
The fungal biota detected in the phyllosphere of S. umbrosus, a species with a rather narrow ecological amplitude (Calvo & Aedo, 2015; Czarnecka, 1995; Czarnecka & Kucharczyk, 2014; Grulich & Hodálová, 1994), was represented by 15 species (even nine per one collection series) and three hyperparasites. It can be regarded as rich, especially in comparison with data for other plant species living in xerothermic communities, which may be related to the very large leaf area in S. umbrosus, compared to leaves of other species, and the relatively long durability of leaves, especially in vegetative rosettes. For example, Romaszewska-Sałata and Mułenko (1983) and Ruszkiewicz-Michalska (2006) found a maximum of 7 fungal species on one host, i.e., on Taraxacum officinale F. H. Wigg. in xerothermic communities near Drohiczyn and Mielnik on the Bug River and on Vincetoxicum hirundinaria Medik. in the designed “Olsztyńskie Skały” reserve (Kraków-Częstochowa Upland), respectively. A greater number of fungal parasites on one host plant were only noted during multiyear investigations in a 1-ha plot established in the linden-hornbeam forest Tilio-Carpinetum in the Białowieża National Park; these were 22 species on Stellaria holostea L. (Mułenko, 1998). Most probably, the antimicrobial effect of UV light in xerothermic grasslands directly exposed to sunlight is involved in the relative reduction of the number of microorganisms compared to a situation when the leaf surface is shaded, e.g., in forests (Karki, 2019). In the case of S. umbrosus, there were fewer species of microfungi in the phyllosphere of plants growing in open microhabitats (sunlit, low, and loose grassland), particularly in the south-exposed plants, than in the patches of grassland dominated by tall grass B. pinnatum and/or shaded by bushes. However, in general, the degree of leaf infection tended to increase during the growing season, likewise the diversity of the fungal biota. The highest numbers of pathogens were recorded in the summer of 2016 and in early autumn of 2015 (nine parasites and two species of hyperparasites, and nine parasites and three hyperparasites, respectively). A similar tendency, i.e., the increasing richness of fungi in the phyllosphere during the summer season, has been observed, e.g., in the Mediterranean oak Quercus ilex (Peñuelas et al., 2012).
The dominant microfungus on S. umbrosus is the polyphagous species C. tussilaginis (Majewski, 1977). In the Roztocze region, the species was observed on 22 hosts from diverse taxa (Kozłowska et al., 2019), among them, also on several plants growing in xerothermic grasslands, brush outskirts, and loose forests on White Mt. (comp. Czarnecka, 1995, 2010): Campanula rapunculoides L., C. trachelium L., C. persicifolia L., Melampyrum arvense L., M. nemorosum L., Euphrasia stricta D. Wollf. ex J. F. Lehm., Tussilago farfara L., and P. sylvestris L. The rust C. tussilaginis was also collected in xerothermic and sandy grasslands, on edges of young mixed and deciduous forests, roadsides, etc., in other physic-geographical regions of Poland (Ruszkiewicz-Michalska, 2006; Ruszkiewicz-Michalska et al., 2012, 2015). This species is present on S. umbrosus throughout the growing season with changeable abundance and spore type. The richness of C. tussilaginis (infection degree) may increase as the ragwort leaves age (cf. e.g., Osono, 2008). This rust fungus forms uredinial aecia on the study host plant observed from the beginning of the season and, additionally, teliospores developing at the end of the season. These spores are thick-walled and, therefore, less responsive to such abiotic factors as temperature and humidity.
The occurrences of the other species were characterized by varied repeatability in the successive collection series and seasons. For example, B. exigua and L. ogilviensis were found five times, Alternaria sp. – four times, A. compositarum and P. cookie – three times, and the majority of pathogenic microfungi were collected twice or only once, which might indicate the so-called “disease escape in time” (Harper, 1977). For example, G. cichoracearum (syn. Erysiphe cichoracearum), i.e., a species that still finds new hosts from the Asteraceae family, was found only in 2014. In Poland, it has been detected on 41 plant species, including species common to xerothermic grasslands: Achillea millefolium L., Carlina acaulis L., Centaurea jacea L., Cichorium intybus L., Hieracium pilosella L., Leontodon autumnalis L., Tragopogon pratensis L., and others (Moliszewska, 2008; Mułenko et al., 2010; Paulech & Paulech, 1996; Ruszkiewicz-Michalska, 2006; Ruszkiewicz-Michalska & Michalski, 2005). G. cichoracearum was collected repeatedly many times in Roztocze in habitats of beech forests, pine forests, mixed coniferous forests, thickets along roads, and roadsides (Kozłowska et al., 2019; Mułenko et al., 2010). It was noted on many species of the genus Senecio in Germany (Jage et al., 2010) and since 1992 on S. rivularis (W. & K.) DC, both in natural conditions, i.e., in a habitat of dried bog alder forest in the Roztocze National Park and in a greenhouse (leg. B. Czarnecka, cit. in Mułenko, 1993). The discussed species G. cichoracearum is a good example of the so-called “host jumping” phenomenon (Crous et al., 2004; Verkley & Starink-Willemse, 2004; Verkley et al., 2013) when a given pathogen “searches” for new susceptible hosts among the plants of the given community where it occurs.
Erysiphales fungi vary in their temperature and humidity requirements. Dry and warm weather usually promotes their spread, whereas low temperatures and precipitation inhibit their growth. In 2014, the mean annual air temperature was 8.5 °C, and the annual precipitation sum was 750.5 mm. In turn, such an extremely dry and very hot period as 2015, which was the warmest since 1951 (9.3 °C) and drier than usual (precipitation sum 601.5 mm), did not favor the germination of conidial spores, which probably explains why G. cichoracearum did not infect S. umbrosus in this and the subsequent growing season. Previously, the fungus was recorded on White Mt. in 2008 (Czarnecka, 2009). The year 2008 was very warm (annual air temperature 8.5 °C) and extremely wet (precipitation sum 834.4 mm, i.e., 131% of the long-term mean). In addition to the mass appearance of G. cichoracearum and C. tussilaginis, other microfungi were abundant on S. umbrosus, e.g., B. exigua (formerly Phyllosticta senecionicola Petr.), Ph. jacobaeae, and Septoria sp. (Czarnecka, 2009). Additionally, the ragwort population was then much more abundant (Czarnecka, 2020), what increased the efficiency of so-called “disease escape in space” (Harper, 1977). In 2004, G. cichoracearum was collected from the same host plant in the nature reserve “Brzeźno” (Pagóry Chełmskie/Chełmskie Hills, eastern Poland), where it grows on islets within calcareous meadows and fens (leg. B. Czarnecka & K. Kowalska; cit. in Świderska-Burek, 2015).
Although a majority of the fungal taxa identified on S. umbrosus are common species in Poland, we also found A. compositarum, a new species for Poland, which was described by Davis (1919a, 1919b) in North America on leaves of Aster drummondii Lindley, recently Symphyotrichum drummondii (Lindl.) G.L. Nesom, on Helianthus strumosus L., and Eupatorium urticaefolium Reichard [syn., e.g. Ageratina altissima (L.) King & H. E. Robins]. It usually forms 14–24 × 4–6 µm conidia. The specimens collected on White Mt. fit the original description, whereas Mel’nik et al. (2000) reported smaller spore sizes: 10–16 × 3.5–5 µm. In North America, this species occurs commonly on representatives of the family Asteraceae. In Europe, since 1873, the species was noted as Coleosporium senecionis J. Kickx fil. on taxa from the Senecio doria group, i.e., S. umbrosus, S. macrophyllus or S. doria in Transylvania (Romania), in botanical gardens of Gottingen, Muenchen, and Berlin (Germany), and in Copenhagen (Denmark). In 2015, it was found on S. doria in the Danube Valley, Lower Austria (Farr & Rossman, 2022). A. compositarum has been also reported from a few localities on Helianthus annuus L. in Europe and China (Farr & Rossman, 2022; Sałata, 2002).
Additionally, we found new fungus/host plant combinations for C. tussilaginis, which is a very common species in the world (Farr & Rossman, 2022) and in Poland (Majewski, 1977). The author provides only a short note on the occurrence of C. tussilaginis on plants from the S. doria group in the botanical gardens in Krakow and Wroclaw; the data originate from the late 19th and early 20th centuries. Recently, it has been noted on S. macrophyllus only in the former Soviet Union (Farr & Rossman, 2022).
The present study provides equally interesting data on hyperparasitism. New fungus/mycohost/plant host combinations were found for C. uredinicola and R. coleosporii. Both these hyperparasites did not appear before summer. C. uredinicola is very common on rust fungi, downy mildews, and powdery mildew fungi in Asia, Australia, the Caribbean, Europe, New Zealand, and North and South America (Heuchert et al., 2005). This species was noted earlier in several localities in Poland on rust fungi, powdery mildew fungi, and on cave walls (Dolińska et al., 2011; Dolińska & Schollenberger, 2012; Moliszewska, 2008 – as C. aecidiicola Thüm.; Mułenko et al., 2008; Pusz et al., 2015; Ruszkiewicz-Michalska, 2010). To date, only C. aecidiicola has been noted on C. tussilaginis (Bensch et al., 2012; Heuchert et al., 2005). This species forms verruculose conidia, but the specimens collected on C. tussilaginis on S. umbrosus have almost smooth spores. Based on the morphology of the conidiophores and conidia, the collections on C. tussilaginis from White Mt. seem to be conspecific with C. uredinicola. Other fungicolous species, i.e., C. gallicola B. Sutton and C. gerwasiae Heuchert, U. Braun & K. Schub., are quite different. C. gallicola has strongly branched conidiophores, densely covered with numerous conidiogenous loci, while C. gerwasiae forms thicker fasciculated conidiophores, often with two-layered walls (Bensch et al., 2012). R. coleosporii occurring commonly on rust fungi in Europe, Asia, and North America (Braun, 1998) was noted in ca. 20 localities on C. tussilaginis on different host plants in Poland (e.g., Bartkowska, 2007; Ruszkiewicz-Michalska et al., 2012; Wołczańska, 2005). The specimens collected on C. tussilaginis on S. umbrosus are a new fungus/mycohost/host combination worldwide.
Many researchers emphasize the key role of co-evolutionary interdependence between pathogenic microorganisms living in the phyllosphere and their hosts and on trade-offs between the host range and other life-history parameters (Maron et al., 2019; Poisot et al., 2011; Thrall et al., 2007; Wininger & Rank, 2017). They also underline the importance of both pathogenic and symbiotic interactions at different levels of plant organization: growth and health of individual plants, plant population abundance, and the productivity of the ecosystems, especially in the era of rapid environmental changes. They often lead to fragmentation and isolation of community patches in the landscape, simplification of the trophic structure of ecosystems, and even monotypization or appearance of alien species (Colling & Matthies, 2004; Leach et al., 2014; Lindow & Brandl, 2003; Remus-Emsermann & Schlechter, 2018; Stone et al., 2018). As a rule, it may be predicted that as biotic complexity increases, pathogen specificity tends to decline, whereas mutualistic specificity will increase and vice versa (Thrall et al., 2007).
The numbers and diversity of microfungi in the phyllosphere of S. umbrosus may be recognized as rich in comparison with data for other plant species living in xerothermic communities. However, the authors are aware that the species richness of phytopathogenic microscopic fungi on the study host plant, which occurs in different vegetation types (xerothermic grasslands, bushes, forests, and calcareous meadows and fens), may be substantially greater. The investigations should be continued to complement the spectrum of fungal pathogens both on the “flag” species, e.g., the ragwort S. umbrosus, which is vulnerable in Poland, and on entire plant communities where it occurs. The rationale is the need to study the potential colonization of its phyllosphere by species of pathogenic microfungi living on other host plants.