. Introduction
Rhizoctonia solani belongs to the phylum Basidiomycota. This pathogen is widely distributed worldwide. It has a wide host range and attacks more than 250 plant species. This fungus attacks the root systems, stems, seedlings, fruits, and leaves. In the natural environment, they occur in the form of mycelial hyphae. R. solani can survive in the form of sclerotia for up to several years in soil or plant debris (Nagrodzka et al., 2016).
The mycelium produced by R. solani is colorless in the initial stage of development and ranges from light to dark brown in later stages. It consists of cells with an elongated shape and numerous branches. Each cell is tapered at the end and has a transverse septum located near the junction with the neighboring cell (Moliszewska, 2002; Stępniewska-Jarosz, 2006).
To date, 13 anastomosis groups (AG) have been identified within R. solani. These groups were further subdivided into subgroups. This subdivision is due to the great heterogeneity of R. solani and the ability of hyphae to join. AG2 and AG4 are the most frequently described groups because of their high pathogenicity in various plant species (Stępniewska-Jarosz, 2006).
This study focuses on the little-studied group AG5, which is considered to have low plant pathogenicity. It can be found on sugar beet seedlings with symptoms of seedling damping-off, among others. This group has not yet been fully characterized and described, and progeny production has not been developed under laboratory conditions. This study aimed to describe the morphological profile of the progeny obtained from basidiospores of one AG5 field strain.
. Materials and Methods
Isolates
The isolate R. solani ID23 was used in this study, as the maternal strain was isolated in 1997 from diseased sugar beet seedlings with symptoms of damping-off. Seedlings were collected from a field area close to Opole, Poland. All fungi (Table 1) used in this study belonged to the R. solani collection created by E. Moliszewska at the University of Opole, Institute of Environmental Engineering and Biotechnology, and are routinely stored on PDA slants in a refrigerator (for usual use) and on barley grains overgrown by mycelium in a deep freezer for long-term storage.
Table 1
List of tested R. solani strains (various colors indicate SBIs obtained in separate cycles of sexual fruiting).
Species determination for ID23 was performed using classical anastomosis tests, which showed that this strain belongs to AG5. This was confirmed by isolation and sequencing of the ITS1-ITS2 fragment of ribosomal DNA. The sequences were compared to those in the GenBank database (Moliszewska, 2009). Recently, this sequence was confirmed using the BLAST tool in the NCBI database.
Perfect Stage Culturing - Basidiospore Collecting
This study was based on a group of isolates obtained by culturing R. solani AG5 basidiospores under laboratory conditions. All cultured basidiospores were collected from R. solani AG5-labeled ID23 during several cycles of sexual fruiting. Initially, basidiospore production and collection were performed for a few isolates of the AG5 group using the soil-over method described by Toda and Hyakumachi (2006) (Figure 1). Finally, fruiting was obtained for only one isolate, which was coded as ID23.
Figure 1
Perfect stages of Rhizoctonia solani. Left - fruiting visible on the soil surface. Right - basidium and basidiospore of Rhizoctonia solani - dyed with safranine 0; (×400).
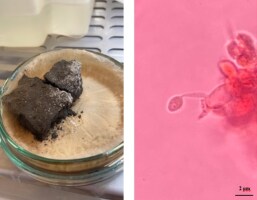
Basidiospores were trapped on water agar (WA) and cultured at approximately 20 °C. Each mycelium developed from a basidiospore was transferred to a fresh PDA medium. WA plates with no germinating basidiospores were placed in a refrigerator and incubated at approximately 10 °C for the next two months, and then newly developed mycelia were transferred to a fresh PDA medium. The letter ‘L’ has been added to the lab code for the mycelia developed in 10 °C.
Heterokaryon Formation
Single basidiospore isolates (SBIs) served as progenies and parental strains to produce potential heterokaryons (Hs). Potential Hs were obtained through the cultural pairing of SBIs (parental strains) and their cleavage from the contact sites of the two paired strains. Strains were inoculated on the opposite sides of the Petri dish on a PDA medium with 2% activated carbon. After the distinctive tuft was created, potential Hs were transplanted onto a new PDA medium.
Morphology
The progenies of two generations (SBIs and Hs) were analyzed in terms of their morphological diversity. For the purpose of this study, all strains were inoculated onto a PDA medium and incubated at 10 °C, 20 °C, and 30 °C in the dark. The daily mycelial growth rate was observed for each strain. After two weeks of incubation, mycelia were morphologically characterized (color, concentric zonation, mycelial height and fluffiness, compactness, and presence of sclerotia). The experiment was performed with four replicates.
Morphological features of the analyzed fungi were compared by creating a similarity/dissimilarity tree using NTSYSpc 2.21w software and the UPGMA method.
Fungicides
In the next phase of the research, PDA supplemented with fungicides, azoxystrobin, thiophanate-methyl, and thiuram, used at concentrations of 10 and 100 ppm, allowed for evaluation of the resistance of the mycelium to these fungicides. Plates with PDA without supplements served as controls. In both experiments, the growth of mycelia [mm] was measured every 24 h along two vertical lines on the bottom of the Petri dishes for all tested samples until the mycelium covered the entire dish (Φ90 mm). The fungicide test was performed at 21 ± 2 °C. All experiments were performed in triplicate.
The results of measuring mycelial growth are expressed as the range of mycelial increases per day (mm/d).
Based on the data obtained from the test with fungicides, the coefficient of growth inhibition was calculated according to Abbot’s formula (Abbott, 1925):
where ΔH is the coefficient of linear growth inhibition of the fungus [%], K0 is the diameter of the colony on the control dish [mm], and F is the colony diameter on the dish with fungicide.
In the final stage of the study, microscopic measurements of the samples incubated at 20 °C were performed. The average hyphae diameter, as well as the minimum and maximum diameters [µm], were measured at a magnification of ×400.
. Results
Isolates – Culturing, Identification, Heterokaryons, and Monokaryons
The basic classification of the ID23 isolate, and DNA isolation was performed in a previous study by Moliszewska (2009). Maternal isolate ID23 was confirmed to be R. solani AG5. The sequence, showing 99.86% identity with the R. solani AG5 strain (accession No. JQ946291) and 100% identity with Thanathephorus cucumeris strain GZ-5, was determined to be AG5 (accession No. KR006028), both of which were deposited in GenBank. The ID23 sequence was submitted to the NCBI GenBank database under accession No. OP601638.
Strains were incubated at room temperature for two weeks after which morphological characteristics were macroscopically evaluated, starting with the parental isolate. Based on this evaluation, the progeny was further assessed. Maternal R. solani ID23 was characterized by a light brown fluffy structure. Irregularly shaped and brown sclerotia were visible on the surface of the mycelium. Zonation of mycelial growth was not observed on the surface of the maternal ID23 isolate.
The color of the tested strains ranged from light beige to light or dark brown. Individual isolates also differed in mycelial fluffiness, height, and hyphae density. In a few cases, concentric zonation and sclerotia have also been observed. Unfortunately, the distinction of the progenies by color to distinguish individual basidiospore-born isolates and Hs is not possible. However, they were almost grouped separately, in clusters A or B, in a dendrogram of similarity. Cluster A contains only SBIs and maternal ID23, while Cluster B contains Hs and six SBIs (ID23II, III, V, VI, IX, X) (Figure 2).
Figure 2
Similarity tree based on morphological features of maternal strain ID23 and its progenies. Strains with the letter “H” in a code are heterokaryons (A – Cluster A; B – Cluster B).
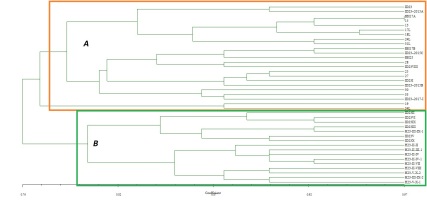
In each offspring group, mycelia took on different hues. Some strains developed a light beige to almost white mycelium, whereas others had a dark brown color. However, most isolates were light brown in color. Concentric zonation within the cultures was observed in strains whose mycelium grew flat and did not form sclerotia on their surfaces. In the observed isolates, all tested Hs were found to have the ability to form sclerotia as compact mycelial structures. They appear in the form of brown- and creamy-colored densely packed structures. These structures were also characterized by irregular or almost spherical shapes (Table 2).
Table 2
Morphological characteristics of R. solani AG5 isolates.
R. solani AG5 isolates are characterized by their very diverse mycelial appearance. Depending on the strain, they can vary in fluffiness and height. The study showed that high and fluffy mycelia were formed by all potentially heterokaryotic isolates and a few single basidiospore isolates. The remaining R. solani strains obtained from single basidiospores grew flat and formed compact structures (Table 2).
Based on the calculations, the daily growth at 10 °C ranged from 2.5 to 15.2 mm/d, with the maternal strain being 4.6 mm/d (Figure 3). All other isolates, except a few strains signed with the letter “L,” had a similar daily growth, oscillating between 4.5–6.5 mm/d values. The SBI isolates labeled “L” were characterized by the following growth values: 18L - 13.5, 17L - 14.8, 34L - 12.5, 31L - 15.2, and 24L - 14.7 mm/d, indicating that these samples grew most favorably under these temperature conditions and their growth rate was much higher than that of the maternal strain ID23. When Hs were compared with their ID23 parent isolates, their growth rate was found to be inconsiderably different from that of the parent, ranging from 3.2–6.4 mm/d.
Figure 3
Influence of temperature on the average daily growth (mm/d) of tested mycelia AG5 group of R. solani.
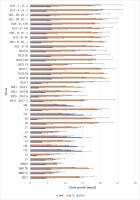
At a temperature of 20 °C, the daily growth rate for the mother strain ID23 was 14.2 mm/d and the maximum value for the isolates studied was 21.4 mm/d (isolate ID23 II), and the minimum value was 5.7 mm/d (isolate 15) (Figure 3).
Increasing the incubation temperature to 30 °C did not drastically affect the modification of mycelial growth rates when the temperature was increased from 10 °C to 20 °C. The daily growth of the maternal strain ID23 under these conditions was 14.1 mm/d. The highest recorded value was 21 mm/d (isolate H23-V-X-2), whereas the lowest was six mm/d (isolate 18L), which clearly confirmed the tendency of the isolates to have different optimal growth temperatures. At higher temperatures, the growth rate did not change over a wide range, and it was similar in most of the tested strains. The lowest values of daily growth at 30 °C were observed for “L” labeled isolates. Hs at this temperature showed slightly higher growth rates than the parental strains. Only R. solani H23-II-VIII grew like one of its parent strains ID23 VIII (17.8 mm/d). For the other Hs, the growth rates were 0.2–2.9 mm/d higher at 30 °C than at lower temperatures (Figure 3).
The graph in Figure 3 shows a comparison of the strains tested at all incubation temperatures. Based on our observations, the most favorable temperature for rapid mycelium growth was concluded to be 30 °C. However, the values observed in the tests conducted at 20 °C suggest that a temperature range of 20 °C–30 °C may be optimal for the growth of R. solani. At a low temperature (10 °C), the growth rates of the strains tested were less effective, as these isolates required a much more extended incubation period. However, “L” labeled-isolates (obtained after the basidiospore incubation in the refrigerator) were the only ones that showed more favorable growth at 10 °C. This confirms that these isolates did not spontaneously activate their growth at room temperature, which was too high for them, and transferring the isolation WA plates to the cold room allowed them to grow at the beginning of this study.
Statistical analysis (p = 0.05) showed that the growth of the tested groups of fungi (maternal isolate, SBI, and Hs) did not differ inconsiderably at 10 °C. However, considerable differences were observed between SBI and Hs at 20 °C and 30 °C. Maternal isolates did not differ notably in either group of progeny.
Microscopic Evaluation of the Examined Strains of R. solani AG5
Microscopic evaluation of the mycelia was performed to determine the hyphal diameter (µm), considering the minimum, maximum, and average values. Maternal strain ID23, five exemplary potential Hs, and 12 single basidiospore isolates were considered in this test. Regarding anatomical structure, the hyphae of the progeny of R. solani AG5 were found to have diameters ranging from 2.93 µm to 15.60 µm. The mean size of the hyphae in potential Hs ranged from 3.90–13.65 µm. In the parent strain ID23, the mean value was 6.14 µm, the minimum was 3.90 µm, and the maximum was 7.80 µm. For SBI, these values were 2.93–15.60 µm. This could mean that single basidiospore isolates present more significant differences in the diameter of the hyphae (Figure 4).
Analysis of the similarity between the Hs and their parent strains revealed that the maximum size of hyphae of isolate H23-II-III was the same as that of its parent strains (9.75 µm); however, the mean value of the measurements was higher than that of isolate ID23 II, but identical to that of ID23 III. Isolate H23-II-VIII had a larger diameter than its parent strain ID23 II; however, it was similar to that of the second parental strain ID23 VIII (Figure 4). Another potential heterokaryon, H23-III-IX-2, resembled its parental isolate ID23 IX in the minimum and maximum diameter of hyphae. In contrast, in this case, the overall average size was smaller than that of both parental strains. The maximum diameter of the R. solani H23-V-X-1 hyphae was identical to that of ID23 V and ID23 X, and the minimum diameter was the same as that of ID23 V, whereas a more remarkable similarity was observed in the mean value of this parameter with R. solani ID23 X. General analysis of the relationship based on the diameter of the young hyphae of R. solani AG5 showed that the Hs did differ inconsiderable from the parental strains in their morphological structure. In the analysis, in most cases, the progeny was observed to adopt the characteristics of one of the parents or have the features of both, on average.
Fungicides
Two fungicide concentrations were used in this study: 10 ppm of active substance, which refers to the possible field concentration of the preparations, and 100 ppm of active substance, which is extremely high; therefore, sensitive isolates should be extracted. On the medium containing 10 ppm of azoxystrobin (Amistar), the growth of the mycelium was found to be inhibited by 29% (isolate ID23 VI); however, in the case of the maternal strain ID23 and isolates SBI 17A, 24L, and ID23 VIII, it did not contribute to the slowing down of mycelial growth (Figure 5).
Figure 5
Influence of selected fungicides (10 ppm) on the growth of individual isolates of R. solani AG5 showed as an inhibition coefficient (ΔH%) (PDA+A - azoxystrobin, PDA+M - thiophanate-methyl, and PDA+T - thiuram).
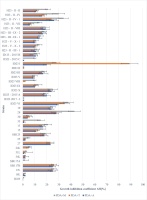
On the 10 ppm medium with thiuram, some isolates grew unchanged, which means that the fungicide did not affect mycelium growth (isolates ID23, SBI17A, and ID23 2017-I), and the remaining strains slowed down their growth to a greater or lesser extent. The calculated growth inhibition coefficient (ΔH) varied between 0%–35%, and in one strain, the value was as high as 89% (ID23 I) (Figure 5).
A slight slowdown in mycelial growth was observed on a PDA medium containing 10 ppm thiophanate-methyl. The calculated growth inhibition coefficients (ΔH) varied between 1%–43%, with low values for isolates ID23, ID23 II, and ID23 2017-I and the highest value for R. solani isolate 28 (Figure 5).
Increasing the concentration of the active substance to 100 ppm resulted in increased growth inhibition of R. solani AG5 isolates (Figure 6). On the PDA medium with azoxystrobin, the growth rate of the tested fungi was inhibited by 0%–66%. Therefore, in most cases, it was inhibited almost twice as effectively as at a lower fungicide concentration. The lowest value of growth inhibition was obtained for the single basidiospore isolate 24L and the highest value for a potential Hs isolate H23-II-VIII (Figure 5). The presence of thiuram contributed to an increase in growth inhibition compared to previous formulations, which in this case was 23%–80%. Thiuram had the least effect on the single basidiospore isolate SBI17A, while the most significant effect was observed on potential Hs, both strains H23-III–IX-1 and H23-III–IX-2 (Figure 6).
Figure 6
Influence of selected fungicides (100 ppm) on the growth of individual isolates of R. solani AG5 showed as an inhibition coefficient (ΔH%) (PDA+A - azoxystrobin, PDA+M - thiophanate-methyl, and PDA+T - thiuram).
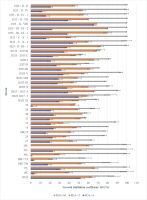
Thiophanate-methyl (100 ppm) affected tested strains the most effectively. In this case, the inhibition of the growth rate varied between 3.5 mm/d (isolate SBI 17A) and 19.0 mm/d (isolate ID23 V) (Figure 5). For “cool” strains, 17L, 18L, 24L, 31L, 34L, and all potential Hs, 100% growth inhibition by thiophanate-methyl was observed (Figure 5). Statistical analysis (p = 0.05) showed that significant differences among the tested groups of fungi (maternal isolate, SBI, and Hs) did not exist with the use of 10 ppm fungicides. In the case of the 100 ppm fungicide concentration, differences were significant between SBI and Hs, and the maternal isolate did not differ significantly from either group of strains.
. Discussion
Isolates of R. solani AG 5 derived from one maternal strain, ID23, presented very different morphological features. The color of the mycelia ranged from almost white to creamy and light to dark brown. The mycelial structure varied from compact, visible zonation, to fluffy, with distinct sclerotia on the colony surface. They appeared only on the edge of Petri dishes during the initial growth phase or developed on the surface of the entire colony. Carling and Sumner (1992) did not provide a morphological description of AG5; however, they detailed basic information on its pathogenicity, concluding that AG5 is far less virulent than AG3 and that it is thiamine auxotrophic.
The morphology of Rhizoctonia mycelia is usually given in general descriptions of subsequent anastomosis groups. For example, Carling et al. (2002) showed that the mycelia of Rhizoctonia solani AG13 were characterized by a light to dark brown color. After a few days of incubation, they noticed zonation in all incubated samples, which disappeared over time. Initially, dark brown sclerotia were observed only near the edge of the Petri dishes, but after a more extended cultivation period, they also appeared randomly scattered over the entire surface of the mycelium. Moliszewska et al. (2020) described two AG11 isolates that formed white-beige- to creamy-colored mycelia with broad concentric zonation, and one of them formed light-colored sclerotia. This confirmed the morphological differences in the anastomosis group. Morphological differences among AG5 isolates isolated from carrots in Sweden were also observed by Marcou et al. (2021). Singh et al. (2018) observed the characteristics of R. solani isolated from seven agroecological zones adapted to maize cultivation in 2018. All 62 strains that were incubated were light to dark brown in color, and their structures varied from fluffy to compact. The studies on the growth of R. solani mycelia presented in this paper have shown that all isolates may grow more or less effectively depending on the ambient temperature. At 10 °C, mycelia’s average daily growth rate was the lowest, ranging from 1.9–15.2 mm. At 20 °C, the growth increase for the tested isolates varied between 6.4–21.4 mm/d. Increasing the temperature to 30 °C did not radically affect the growth activity of the fungal isolates compared to that at 20 °C; in this case, the average growth of the mycelia per day varied between 6.0–21.0 mm. Moliszewska et al. (2020) showed that for two Polish AG11 isolates, the average daily rate of hyphal growth on PDA at 21 °C was 22.8 mm and 22.6 mm, respectively.
The analysis of optimal growth temperature showed that R. solani AG5 grew the most favorably at both temperatures (20 °C and 30 °C), except for the group of isolates, which showed the highest growth activity at 10 °C. This group of isolates was derived from single basidiospores incubated on WA in a refrigerator, which shows how great possibilities are coded in the R. solani AG5 genome regarding optimal growth temperatures. This flexibility proves that it is always possible for some offspring to survive, independent of the environmental conditions.
Bełka and Mańka (2014) conducted tests with different strains of R. solani (including AG5) and showed that AG5 isolates reached the fastest growth among other anastomosis groups (13.9 mm/d). Furthermore, Carling et al. (2002) showed that the average daily mycelial growth for R. solani (AG13) was approximately 28.3 mm at a temperature of 25 °C. This was much higher than the growth rates of our tested AG5 isolates and those observed by Bełka and Mańka (2014) and Moliszewska et al. (2020). Tomaso-Peterson and Trevathan (2007) identified pathogenic fungi in agronomic crops and turfgrasses, among which 12 R. solani isolates were identified. One of them belonged to AG5, and its optimal growth temperature was found to be 21 °C–29 °C.
Harikrishnan and Yang (2004) showed a relationship between temperature and the growth rate of R. solani. Mycelial growth was observed in different anastomosis groups, including 15 isolates of AG5. Although there were differences in growth rates among the tested isolates, in most cases, the fungi showed more effective growth with increasing temperature. None of the tested isolates showed the ability to grow below 5 °C. The most rapid growth was recorded at 20 °C, 25 °C, and 30 °C, which agrees with our observations.
Papavizas and Davey (1961) found that the optimum temperature that affects the saprotrophic activity of R. solani depends on the soil type. For example, in a greenhouse where loamy sand was used, the optimum temperature for mycelial activity was 20 °C, and at 30 °C, the efficiency of the saprotrophic mycelial development decreased. They also demonstrated that R. solani showed the highest saprobic activity in fine sand at 26 °C–30 °C. Similar observations have been reported by Martinson (1963). Their research showed that the activity of R. solani mycelia increased with increasing temperature. They also determined the optimal growth temperature in the range of 15 °C–25 °C and found no differences between 25 °C and 30 °C. The last observation was similar to that observed for our tested isolates. Our group of “cold-grown” isolates refers to their lowest growth temperature (15 °C). However, they were seen to grow the most intensively at 10 °C. The growth of R. solani depends not only on temperature but also on the natural features of the isolates; the medium or soil type is also very important. R. solani is commonly known as a fast-growing species. The importance of preferred growth temperature for R. solani was shown by Minier and Hanson (2021), who reported that below 15 °C, a risk of Rhizoctonia damping-off was observed on sugar beets.
Other factors that may influence the R. solani growth rate in agricultural soils are fungicides. This study investigated the following groups: strobilurin derivative — azoxystrobin, dithiocarbamate — thiuram, and benzimidazole — thiophanate-methyl. These compounds were found to inhibit the growth of R. solani mycelia, with thiophanate-methyl showing the highest antifungal activity. Thiophanate-methyl at a dose of 100 ppm contributed to almost total growth inhibition in many of the tested isolates. Two concentrations of fungicides were investigated in the study: lower (10 ppm) as an appropriate dose adequate for field applications to the soil and higher (100 ppm), which was expected to inhibit the growth of fungi completely. Kucharska et al. (2018) tested such compounds as propiconazole + cyproconazole, penthiopyrad, prochloraz, tebuconazole, and azoxystrobin against R. solani AG2-2B and AG4. All of these could notably reduce or inhibit the growth of R. solani. The least effective fungicide was azoxystrobin (efficacy of 47.30%–91.14% inhibition), whereas the most effective compounds were propiconazole and cyproconazole. Moliszewska et al. (2020) showed that hymexazole, specific to Oomycetes, could increase the growth of both tested fungi from 13.5% to 28%. However, thiuram inhibited them by 35.8%–74.7% depending on the dose of the preparation. They concluded that both tested strains differed in response to both fungicides, similar to our observations for AG5 isolates. The differences observed for our isolates may result from the individual features inherited from the maternal isolate ID23. This also shows that some level of potential resistance to fungicides could be acquired in the field by the maternal isolate or by natural genetic changes that may occur during long-term storage.
Our research shows that R. solani AG5 is represented by isolates differing in morphological features and some physiological and genetic properties. A more detailed study of this group is currently needed because R. solani AG5 has been isolated from different hosts. Isolates have been found among other AGs in soil, cucumbers, potatoes, tomatoes, peppers, red cabbage, wheat, turfgrasses, cereals, beans, soybeans, sugar beets, pine seedlings, and apple plants (Ajayi-Oyetunde & Bradley, 2017; Amaradasa et al., 2013; Bełka & Mańka, 2017; El-Sharouny, 2015; Erper et al., 2021; Erper & Yildirim, 2017; Fiers et al., 2011; Gondal et al., 2019; Melzer et al., 2016; Woodhall et al., 2012). Recently, Hassan and Chang (2021) described the first case of damping-off in ovate-leaf Atractylodes ovata caused by AG5. They may exist as single pathogens, as in the Japanese monkshood (Aconitum japonicum subsp. subcuneatum) (Mori et al., 2020), wheat in the United Kingdom or Turkey, as well as barley and potato, or in mixed groups of different Rhizoctonia-complexes in which they usually play a minor role (Erper et al., 2011; Erper & Yildirim, 2017; Gonzalez et al., 2001; Moliszewska, 2009; Woodhall et al., 2012). According to the results of Moliszewska (2002), R. solani AG5 may comprise approximately 60% of the total amount of R. solani strains isolated from sugar beet seedlings in Poland (Moliszewska, 2009). R. solani AG5 can cause seedling damping-off and root rot in sugar beets and decrease plant size by slowing and stunting development (Moliszewska, 2002); however, these observations require a more detailed inspection. Recently, similar results were reported by Erper et al. (2021) for red cabbage. Some data suggest that members of AG5 may also act as severe pathogens, for example, on chickpeas in Turkey (Basbagci & Dolar, 2021). Hence, this strain seems to have become more dangerous than previously thought. They also should not be considered a homogenous group; as Lübeck (2004) suggested, there are three possible groups based on RFLP patterns. This was partially confirmed by the groups formed in the phylogenetic tree (Figure 2). Therefore, they should be studied to understand their evolution in nature and how they use plants as nutrient sources. Knowledge gained from this type of research will prevent their potentially harmful influence on crops in the future.
. Conclusions
Research has shown that sexual reproduction leads to the segregation of features among progenies of the maternal isolate. In the tested isolates, several types of morphological features were observed, which were divided into two groups, one of the heterokaryons and the second of the homokaryons derived from basidiospores grouped with their maternal isolate ID23. This study is the first to show that SBI progenies mostly carry a morphology similar to the maternal isolate, but after crossing them, the next generation of heterokaryotic progenies creates a separate group of morphological characteristics (Figure 2). Other attributes of new generations, such as the optimal temperature of growth or sensitivity to fungicides, also provide distinct information. A group of cool-preferring isolates that were sensitive to some fungicides were identified. Higher sensitivity to fungicides has also been observed in heterokaryons than in mono/homokaryons.
This study shows the changes in the next generation of R. solani. Our observations of changes in progenies may contribute to our understanding of how R. solani AG5 modifies its aggressiveness to plants and resistance to fungicides. In our opinion, further research is needed to obtain fully described changes in the group AG5, to understand the details of its sexual reproduction, and to evaluate its notable in crops.