. Introduction
Amaryllidaceae is a family of monocotyledon plants which are found commonly throughout the warmer regions of the world and are famous for their large ornamental flowers. An additional outstanding feature of this family is the consistent presence of various secondary metabolites with promising medicinal and agronomic potential (Fishchuk & Odintsova, 2021; Y. Wang et al., 2018). For these reasons, it is essential to establish or improve the methods of their propagation. Hippeastrum is commonly propagated in three ways: seeds, offset bulblets, and twin-scaling (Vijverberg, 1981). Due to the large variations in the characteristics of their flowers, plant shape, time of flowering etc., propagation by seeds is usually reserved for the development of new cultivars. Moreover, Hippeastrum seeds have low germination energy, which makes growing from seeds long and ineffective (Ephrath et al., 2021; Vijverberg, 1981).
To date, bulb separations or division of the mother bulb are the most efficient propagation techniques in gardening. However, such methods of manipulation are effective when cultivars produce adventitious bulbs, which is limited in many genera. As shown by studies on the bulb cutting methods for the propagation of Hippeastrum hybridum (Andrade-Rodríguez et al., 2015; Kharrazi et al., 2017), separation into twin-scales is the most effective. However, when fragments of bulbs were planted directly in the soil during experiments, a high level of infection was noted. Therefore, to obtain higher yields and healthy infection-free plants, it was essential to develop in vitro methods. Previous research mainly focused on the induction of bulblets on twin-scale explants, their acclimatization to abiotic factors, and the growth of their biomass (Amani et al., 2015; Aslam, 2012; Sultana et al., 2010). However, it is known that plants obtained from in vitro culture are more prone to adverse effects of both biotic and abiotic factors (Nawrot-Chorabik et al., 2022). The cause of this phenomenon is that growth under high humidity in culture vessels leads to morphological and physiological alterations that in many cases compromise the survival of plantlets when they are transferred to the acclimatization greenhouse (Hazarika, 2003). In such plants, poor development of the epicuticular wax layer and malfunctioning of stomata were noted (Hazarika, 2003). The chemical composition of surface wax is also found to be different in plants grown in soil (Fal et al., 1990; Hazarika, 2003; Rodrigues et al., 2014; Sumi et al., 2022). It is known that the stomatal movements play an active role in restricting pathogen invasion as part of the plant innate immune system (Melotto et al., 2008). Moreover, cuticle breakdown products, which are among the first elicitors to be generated during infection and activated developmental processes during pathogenesis, are perceived by the plant. In addition, cuticle and wax components (cutin monomers and secondary metabolites such as flavonoids and triterpenoids) of various types induce a syndrome of reactions that often results in resistance to pathogens (Serrano et al., 2014; Ye et al., 2020). Therefore, any changes in the cuticle composition can reduce resistance to pathogens.
In this case, the process of pathogen penetration into the plant is facilitated. An important issue is also the method of preparing the material before introduction to in vitro cultures. The plant material is sterilized to eliminate any microorganisms present on the surface of the organs. However, it is known that the presence of non-pathogenic microorganisms (endophytes) induces immune responses and at least partially protects against pathogens (Morelli et al., 2020; Nowak & Shulaev, 2003; Zenkteler, 2001). Therefore, micropropagation in in vitro cultures, although very efficient, leads to the production of plants that require adaptation to ex vitro conditions since those plants do not have such resistance or their resistance is low (Espinosa-Leal et al., 2018).
As has been previously described, the primed state is defined as a physiological status in which plants are capable of quicker and more effective activation of stress-protective responses (Filippou et al., 2012; Liu et al., 2022; Wiszniewska, 2021). It is a kind of “state of readiness” to another stress event, whose duration depends on numerous factors, including the type of stress stimulus, priming agent, and physiological condition of the plant organism. In vitro culture elicitation by using parts of pathogens, extracellular culture filtrates, or stress phytohormones activates the plant’s defense response and increases the synthesis of defense compounds (Fazili et al., 2022; Namdeo, 2007).
In the case of Hippeastrum hybridum, the fungal pathogen Phoma narcissi (=Peyronellaea cutrisii) is responsible for diseases, a decrease in the effectiveness of the formation of daughter bulbs, and even the death of individuals. Following contact with the pathogen or mechanical damage, Hippeastrum cells produce phytoalexin, which is a mixture composed of an orange-colored chalcone (3,2′4′-trihydroxy-4-methoxychalcone) and 3 flavans (7,4′-dihydroxy-8-methylflavan, 7,3′-dihydroxy-4′-methoxyflavan, and 7-hydroxy-3′-4′-methylenedioxyflavan) (Wink & Lehmann, 1996). When plants have enough time and are able to produce phytoalexin in sufficient quantities, they become more resistant to subsequent infections (Hrynkiewicz et al., 2016).
In the present work, the proper technique of micropropagation of H. hybridum from selected plant organs, i.e. floral elements and twin-scales, was selected while comparing the effectiveness of bulb induction. The research focused on the regeneration system and the ability of the obtained plants to biosynthesize phytoalexins. Finally, an attempt was made to check whether the supplementation of the medium with a Phoma narcissi fungal elicitor or stress phytohormones (JA, MeJA, and SA) would yield healthy plants with potentially better adaptation to further cultivation.
. Material and methods
Plant material and its preparation
The bulbs of Hippeastrum hybridum (PolPest, Poland) and young flower buds were used as experimental materials. The bulbs (4–6 cm in diameter) were cleaned of the dry leaves covering them, rinsed in tap water for 1 h, and sterilized in 70% (v/v) ethanol for 30 s and in 50% (v/v) commercial bleach with sodium hypochloride for 60 min. Subsequently, the plant materials were rinsed four times in sterile distilled water (each for about 5 min). The apical halves were cut and the basal section twin-scale explants (5 mm × 5 mm with the basal plate) were isolated. For the latter material source, the apical part of an immature inflorescence stem (the stem length was not longer than 2 cm) was isolated and translation sterilized similarly as described above. Afterwards, the bracts were removed and fragments of peduncles (3–4 mm in length), receptacles (3–4 mm in length), ovaries (2–3 mm), and perianth (5 mm × 5 mm) were isolated.
Establishment of the culture
All types of explants were transferred onto MS medium (Duchefa) containing 18.0 µM benzylaminopurine (BAP) and 0.54 µM naphthaleneacetic acid (NAA) (Sigma-Aldrich) as in Aslam (2012), 3.0% sucrose, solidified with 0.7% agar with the pH adjusted to 5.8 before autoclaving. Sets of eight explants were cultivated in 300 ml jars, each containing 50 ml of the prepared medium. They were cultivated for 12 weeks under continuous white fluorescent light (70 µmol ⋅ m−2 ⋅ s−1) at 26 ± 1 °C in a growth chamber. The explants were subcultured at 4-week intervals. After the first period of culture, the receptacles were cut longitudinally into four parts. The multiplication rate and percentage of explants producing shoots were analyzed after 12 weeks of culture. The obtained shoots were rooted on MS medium without plant growth regulators (PGR). The shoots were cultured in the conditions described above. After four weeks, the percentage of rooting and the number and length of roots were analyzed. The roots were then gently removed (shortened to 2–3 mm) and the microcuttings were transferred to the elicited medium.
Phoma narcissi cultivation and production of the fungal elicitor
In this study, we used a strain of Phoma narcissi (=Peyronellaea cutrisii) marked as P2. This strain was isolated from infected bulbs of the ‘Jan’ variety of Knight’s star lily (Hippeastrum hybridum hort. cv. ‘Jan’.), which was obtained from the National Institute of Horticultural Research in Skierniewice, Poland. Biotic elicitors and elicitation medium were prepared according to the method described by Hrynkiewicz et al. (2016) with some modifications. The strain was first cultivated on solid medium (Potato Dextrose Agar) in Petri dishes at 26 °C ± 2 for 7 days of incubation. For the liquid culture of P. narcissi, 100 ml of Potato Dextrose Broth (PDB) medium contained in 300 ml Erlenmeyer flasks were used. The medium was inoculated with 1 plug (10 mm diameter) from agar plates containing P. narcissi and kept at 26 °C on a rotary shaker (70 rpm) for 7 days of incubation. The mycelium free fungal extracellular culture filtrate (F) was obtained by stepwise purification of the culture. First, the mycelia were separated from the culture medium by filtration through Millipore nylon membrane filters with a pore size of 0.45 µm. Then, F was sterilized using a 0.2 µm syringe filter (Millipore) and ultimately used as a biotic elicitor.
Phytoalexin biosynthesis
In the experiment, the fungal culture filtrate (F) was used as a biotic elicitor, while jasmonic acid (JA), jasmonic acid methyl ester (MeJA), and salicylic acid (SA) were used as signaling molecules involved in stress responses in plant cells. The F was added to get the final concentration of the filtrate 1, 10, 25, and 50% solution. JA, MeJA, and SA (all Sigma-Aldrich) were dissolved in ethanol (50%) and filtered using PEP-syringe filters with a pore size of 0.2 µm to a 100 mM concentration. Then, the solutions were added to the autoclaved MS medium without PGR to reach the final concentrations of 1, 10, and 100 µM. Equal volumes of ethanol were added to the controls. After four weeks of growth, microcuttings were collected.
Phytoalexin isolation from Hippeastrum hybridum microcuttings
The elicited plants (bulbs) obtained from the in vitro culture were cut into small pieces (4 × 4 mm) and kept on Petri dishes at 20–22 °C in continuous light (70 µmol ⋅ m−2 ⋅ s−1 cool white Polam fluorescent tubes) at high humidity (Hrynkiewicz et al., 2016). The microcuttings cultivated on medium without any elicitors constituted the control. Materials for the study were collected immediately (0 h) and subsequently at 24, 48, 72, and 96 h after the cut. The collected samples were quickly frozen in liquid nitrogen. The frozen tissues (100 mg) were crushed in liquid nitrogen using a mortar and pestle and extracted with 10 ml of 90% (v/v) methanol by shaking at 200 rpm for 10 min. The macerates were centrifuged for 20 min at 10,000 × g. The liquid fraction was collected and a UV absorption spectrum was obtained on the Shimadzu spectrophotometer (λ = 495 nm) (Hrynkiewicz et al., 2016; Saniewska et al., 2005; Wilmowicz et al., 2014).
Statistical analysis
During the establishment of the culture for each experiment, 3 bulbs and 5 immature inflorescences were used as donor materials. From these materials, at least the following amounts were isolated: 12 twin-scales from the bulbs; 3–4 fragments of peduncles, receptacles, and ovaries from the inflorescence; and 12 explants from the perianth. All experiments were carried out three times. The results were statistically analyzed by means of ANOVA and the mean values were evaluated using the Kruskal–Wallis test for varied numbers of collections (shoot organogenesis) and by Tukey’s multiple range test for equal numbers of collections (rooting stage) at p < 0.05. Biochemical analyses were carried out in three replicates with each containing materials from 3 independent microcuttings (9 plantlets were analyzed for each variant) and repeated twice. Statistically significant differences were assessed using Tukey’s multiple range test at p ≤ 0.05.
. Results
Micropropagation of Hippeastrum hybridum
All types of H. hybridum explants were able to regenerate bulblets. However, the twin-scales, peduncles, and receptacles (Figure 1A–C) had significantly higher regeneration potential than the ovaries and perianths (Figure 1D). The percentage of explants capable of developing shoots oscillated around 76–82%, compared to 22.2% and 16.5% for the ovaries and perianths, respectively (Table 1). The receptacles had the highest rate of proliferation at 5 shoots per explant (Figure 1C). However, the differences in the number of shoots obtained on the twin-scales, peduncles, and perianths (2.3–2.7) were not statistically significant (Table 1).
Figure 1
Micropropagation of Hippeastrum hybridum. (A–D) shoot organogenesis after 4 weeks of culture on MS supplemented with 18.0 µM benzylaminopurine (BAP) and 0.54 µM naphthaleneacetic acid (NAA): (A) on twin-scale, (B) on basal part of peduncle, (C) on receptacle, and (D) on basal part of perianth; (E) microcuttings used for elicitation experiments on MS without PGR; bar = 1 cm.
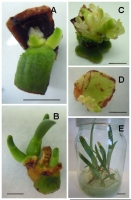
Table 1
Effects of the explant source on shoot organogenesis in Hippeastrum hybridum after 4 weeks of culture on MS supplemented with 18.0 µM benzylaminopurine (BAP) and 0.54 µM naphthaleneacetic acid (NAA). Different letters indicate significant differences at p ≤ 0.05.
The rooting process was translation effective, and more than 90% of the H. hybridum shoots regenerated from the twin-scales and peduncles produced roots, while almost 80% of the receptacles also created roots on the MS medium without PGR. The number of roots per shoot was about 3, and the differences were not statistically significant (Table 2). The root length was not affected by the origin of the shoots, and the average root length ranged from 24.0 to 30.4 mm. All the microcuttings were subsequently used for further research on the induction of immunity (Figure 1E).
Table 2
Effect of the origin of Hippeastrum hybridum shoots on rooting after 4 weeks of in vitro culture on MS without plant growth regulators (PGR). Different letters indicate significant differences at p ≤ 0.05.
Elicitation and phytoalexin biosynthesis
Elicitation of the medium used in the cultivation of the H. hybridum microcuttings with F at the concentrations of 1% and 10% significantly stimulated phytoalexin accumulation when the elicitor directly affected the plant tissue. In the following hours, a weakening effect of the elicitation was found in the case of culture supplemented with the 1% filtrate. On the other hand, the effect of the 10% filtrate lasted up to 48 h after the mechanical damage to the bulbs, and this impact was statistically significant. The highest level of phytoalexin in the microcuttings was recorded in the elicitation variant with the 1% filtrate immediately after the end of the culture (Figure 2).
Figure 2
Post-wounding phytoalexin accumulation in Hippeastrum bulbs grown for four weeks on MS medium without plant growth regulators (PGR) enriched with a fungal culture filtrate (F) in concentrations of 1% (F1), 10% (F2), 25% (F3), and 50% (F50). Medium without the elicitor was used as a control (C). The bars represent the mean concentration of phytoalexin ± standard deviation (n = 3). For each source material, bars with different letters are significantly different (p = 0.05); from 0 to 96 h - time lapse from injury.
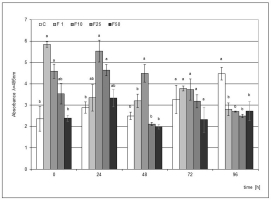
In the case of jasmonic acid, the stimulating effect of the elicitation was visible from 48 h after the mechanical damage to the microseedlings, and the concentrations of 10 and 100 µM JA showed a statistically significant effect. In the following hours, an increasing accumulation of phytoalexin was noted, and this trend was maintained until 96 h. The elicitation of the medium with 1 µM JA during the culture of microcuttings also turned out to be effective, but a significantly higher level of phytoalexin compared to the control was observed only after 72 h. The highest level of phytoalexin was found after the elicitation with 100 µM JA at 96 h after the mechanical damage (Figure 3).
Figure 3
Post-wounding phytoalexin accumulation in Hippeastrum bulbs grown for four weeks on MS medium without plant growth regulators (PGR) enriched with jasmonic acid (JA) in concentrations of 1, 10, and 100 µM. Medium without the elicitor was used as a control (C). The bars represent the mean concentration of phytoalexin ± standard deviation (n = 3). For each source material, bars with different letters are significantly different (p = 0.05); from 0 to 96 h - time lapse from injury.
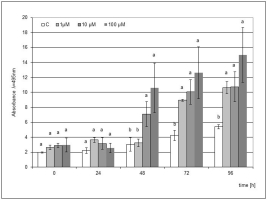
The use of MeJA as an elicitor was not as effective as JA. However, a similar trend was found, where a significantly higher accumulation of phytoalexin was visible from the 48 h point of the experiment after the elicitation of the medium with 100 µM MeJA (Figure 4). In the following hours, an increase in phytoalexin synthesis was noted up to 96 h after the mechanical damage to the bulb. However, this level of phytoalexin synthesis was 1.8 times lower than that after the JA elicitation.
Figure 4
Post-wounding phytoalexin accumulation in Hippeastrum bulbs grown for four weeks on MS medium without plant growth regulators (PGR) enriched with jasmonic acid methyl ester (MeJA) in concentrations of 1, 10, and 100 µM. Medium without the elicitor was used as a control (C). The bars represent the mean concentration of phytoalexin ± standard deviation (n = 3). For each source material, bars with different letters are significantly different (p = 0.05); from 0 to 96 h - time lapse from injury.
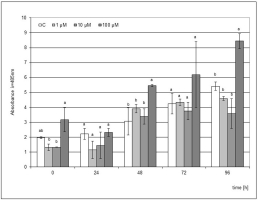
The use of SA as an elicitor was ineffective because a significant stimulating effect on the accumulation of phytoalexin was only observed when the plant material was in direct contact with the elicited medium supplemented with 1 µM SA. The differences compared to the control in this case were statistically significant (Figure 5). However, the level of accumulation was 1.6 times lower than after the use of the post-culture filtrate, where as in the case of supplementation of the SA medium, the strongest effect was noted directly after the end of the culture.
Figure 5
Post-wounding phytoalexin accumulation in Hippeastrum bulbs grown for four weeks on MS medium without plant growth regulators (PGR) enriched with salicylic acid (SA) in concentrations of 1, 10, and 100 µM. Medium without the elicitor was used as a control (C). The bars represent the mean concentration of phytoalexin ± standard deviation (n = 3). For each source material, bars with different letters are significantly different (p = 0.05); from 0 to 96 h - time lapse from injury.
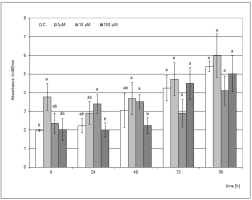
. Discussion
Micropropagation is most often associated with rare plant cultivars, the mass production of plant materials, or the demand for plant parts as medicinal remedies. These are all objectives sought in the case of Hippeastrum, thus encouraging the development of an efficient method of producing their microbulbs. As the industrial demand for Hippeastrum bulbs increases, there is a growing concern about the sustainable supply and conservation of the wild population. Problems with the demand for large quantities of bulbs can be resolved using plant tissue culture approaches. In vitro propagation via organogenesis has been reported for numerous Amaryllidaceae species. Twin-scales are the most commonly used type of explants for shoot regeneration. This technique has been successfully used for Cyrtanthus sp. (Angulo et al., 2003), Galanthus sp. (Resetár et al., 2014, 2017; Staikidou et al., 2006), Leucojum aestivum (El Tahchy et al., 2011), Narcissus sp. (Abdel-Rahman et al., 2017; Santos et al., 2015), Sternbergia fischeriana (Kizil et al., 2014), and Zephyranthes rosea (Mujib et al., 2014). However, regeneration of shoots has also been possible from leaf explants of Cyrtanthus mackenii (Kumari et al., 2017) and Lycoris chejuensis (Oh et al., 2007) and from the peduncles of Clivia miniata (Ran & Simpson, 2005) and Heamanthus coccineus (Ziv & Lilien-Kipnis, 2000).
Several reports about the regeneration of species from the genus Hippeastrum have already been published. De Bruyn (1997) was the first to provide data on in vitro propagation of Hippeastrum by using twin-scales of H. hybridum and a medium supplemented with BAP and NAA. Sultana et al. (2010) also regenerated H. hybridum and they observed that the maximum shoot organogenesis occurred on medium supplemented with 4.0 mg/L BAP. Aslam (2012) found that a medium with 4 mg/l BAP and 0.1 mg/l NAA achieved 80% frequency of multiple shoot and bulblet formation. However, they provided no information on the number of shoots per explants. In the present study, a modified version of the bulb cutting method published by Andrade-Rodríguez et al. (2015) was used to multiply in vitro bulblets.
To the best of our knowledge, regeneration of H. hybridum from inflorescence fragments has not been the focus of previous research, and such results are described and discussed here for the first time. Shoots were formed with about 80% frequency at the base peduncle and the receptacle in close proximity to the petal. The ability of species from the Amarylidacae family to regenerate shoots from petiole fragments was first described for Allium ampeloprasum, Eucrosi radiata, Heamanthus occinus, and Narcissus tazetta (Ziv & Lilien-Kipnis, 2000). Regeneration from young petals by indirect organogenesis for Clivia miniata (Q. M. Wang et al., 2012) and from ovary explants by somatic embryogenesis for a Narcissus cultivar (Malik, 2008) has also been reported. However, using the same types of explants for Hippeastrum shoot organogenesis was found to be much less effective. This may be due to the specific properties of the species, resulting from different levels of endogenous cytokinin (Yucesan et al., 2007) or differences in tissue sensitivity to exogenous growth regulators present in proliferation medium (Lisowska & Wysokinska, 2000).
Rooting is an important phase of micropropagation, and successful commercial micropropagation systems often depend on both the ability of microcuttings to induce rooting and the quality of the root system. The difference in shoot origin can often lead to different rooting response (Lyubomirova & Iliev, 2013; Trejgell et al., 2013). However, in the case of Hippeastrum shoots regenerated from various types of explants (twin-scales, peduncles, and receptacles), no significant differences were observed in the number of shoots capable of rooting, the number of roots per shoot, or in their length in the specified rooting conditions. The process of micropropagation does not only serve to improve the quality of plant production but should also lead to an increase in plant vigor and resistance to both biotic and abiotic environmental factors. The environmental factors, microorganisms and their parts, or post-culture media can pre-sensitize or ‘prime’ the cellular metabolism of plants in order to enable them to respond more quickly and to a higher degree than non-primed ones upon exposure to stress factors (Nowak & Shulaev, 2003; Wiszniewska, 2021). Currently, elicitation is known to be one of the most effective techniques used to improve the biotechnological production of secondary metabolites due to the demand for phytochemicals from the pharmaceutical, food, and agriculture industries. Work involving the use of elicitation through micropropagation techniques leading to improvement in the health of plants has been carried out to a lesser extent. It has been documented that some microorganisms or cell wall and extracted elicitors can be co-cultured in vitro with plant explants to colonize the emerging plantlets (Nowak & Shulaev, 2003; Smetanska, 2008). Plantlets that have contact with pathogens or elicitors establish efficient defenses leading to plantlet resistance. They appear more vigorous and can withstand transplant stress better than non-inoculated controls. Not so long ago, it was noted that specific endophytes isolated from various plant organs conveyed improved growth and development to plantlets of potato, tomato, sweet pepper, cucumber, and grapes among others (Alam et al., 2021) and that these plants also exhibited improved performance under stress, including resistance to pathogens.
The response of plants to biotic stress factors is influenced by signaling pathways regulated by plant hormones such as JA and regulators like SA. These factors coordinate defense induction in plants depending on the type of pathogen. The exogenous application of SA, JA, or its derivatives such as MeJA, is commonly used to enhance the production of economically useful and specialized metabolites (Jeyasri et al., 2023; Sohn et al., 2022). One of the mechanisms triggered by changes in the concentration of stress hormones is the synthesis of phytoalexins. The accumulation of phytoalexins has been reported to be related to the resistance of plants to pathogens. Such a group of secondary metabolites is present in Hippeastrum × hybridum and has been shown to have anti-fungal activity (Hrynkiewicz et al., 2016; Saniewska et al., 2005). In the present research, the ability of plants obtained by in vitro techniques and grown in such conditions to synthesize the proper amount of phytoalexin was analyzed. The elicitation of the media with the post-culture filtrate (P. narcissi) stimulated the accumulation of phytoalexins when the filtrate directly affected the H. hybridum tissue. When this contact was stopped, even the signal amplification through the mechanical damage to the bulbs did not increase the level of phytoalexins in the tissues, compared to the microcuttings which were not treated with the fungal metabolites. Concerning the use of stress phytohormones, the reaction of plant tissues was observed whereby the signal was strengthened only by the mechanical damage and, in the following hours, the level of phytoalexins was found to significantly increase. Moreover, our results confirm the findings reported by Saniewska et al. (2005), which indicate that SA and acetylsalicylic acids (ASA) partially inhibit the formation of phytoalexin in wounded scales, beginning within the first two days after treatment. The inhibitory effect of SA and ASA on the formation of phytoalexin in wounded scales of Hippeastrum may be caused by lowered biosynthesis and accumulation of jasmonates (Wilmowicz et al., 2014).
Studies have confirmed the role of elicitation induced by extracellular culture filtrate (F) and jasmonates in inducing the biosynthesis of phytoalexin in microbulbs obtained by in vitro techniques. It can be used to mitigate the stress effects and regulate physio-biochemical processes in plants.
. Conclusions
The present study revealed that biotechnology gives a unique opportunity to carry out reproduction, proliferation, and phytochemical investigations of Hippeastrum hybridum without collecting them from their natural localities. The establishment of a successful H. hybridum regeneration protocol using twin-scale, peduncle, and receptacle explants provides an opportunity for their successful utilization in commercial propagation. Moreover, the conducted research shows the potential of using elicitation as a tool to obtain initial immunity of H. hybridum to the pathogen Phoma narcissi, which can be used as a priming procedure. Considering all these points, such cooperative applications of both micropropagation techniques and elicitation process may be the beginning of research that will facilitate the elicitation of plants from in vitro cultures to obtain more resistant propagation materials.