. Introduction
The development of agriculture was a clear turning point in human history allowing for the development of settled communities increasing their safety and facilitating cultural development. However, the first domesticated plants were of relatively low quality, but thanks to selection they slowly turned into the high yield crop plants we know today. Initially, this selection process relied on the exclusion of plants with poor germination and seed dispersal, but further development prompted expansion of farming into new surroundings, where farmers began to select for new traits, such as nutritional quality or resistance to pathogens. Some good examples of this include potatoes which were gradually selected for lower glycoalkaloid concentration, rapeseed which was selected for lower glucosinolate, and flax which was selected for lower cyanogenic glycoside content. However, in several cases the improvement of one of the desired traits, like nutritional quality, acted against the improvement of others. For example, potatoes with lowered glycoalkaloid content also had reduced resistance to potato leafhopper, while reduced cyanogenesis in flax resulted in reduced pest resistance in these plants. In modern times, crop improvement approaches have been extended to various new techniques, including mutagenesis using physical (ionizing radiation, UV) and chemical agents (nitrous acid, sodium azide, alkylating agents like ethyl methanesulfonate, hydroxylamine).
However, like all organisms, plants are susceptible to both biotic and abiotic stressors, and pathogens spread with the distribution of plants and their products across different regions of the world. Thus, the use of plants in agriculture with limited genetic diversity in combination with the extensive use of agrochemicals for disease control may result in the development of novel pathogens. In addition, the growing public awareness of healthy diets and the expectation of doubling the production of high-quality food by 2050 are important signals for the development of agro-science. Therefore, it falls to plant science to improve genetic diversity while creating better plants, work which relies on understanding and exploiting plant genomes. This pressure has resulted in the development of various approaches over the last few decades all of which focus on establishing a reliable platform for diversifying the plant genome.
Recent advances in genetic engineering technology have allowed improved generation of positive traits not existing in natural circumstances with high specificity. This technology has been used in the study of plant genes and genomes around the world, with many institutes in Poland focused on the application of this technology to study gene function. However, its application in the generation of new plant prototypes remains rare, with even fewer verified by field cultivation.
As consumers’ concerns and government policies regarding GM (genetically modified) crops for food and feed production become more common in Poland and the rest of EU, there is a potential for the impediment of GM crop development, registration, and application. Despite this resistance to the development of GM crops the University of Wrocław continues to support the development of transgenic plants in the interest of improving global food security and bioproduction.
. Engineered Potato
Our research on potential DNA relaxation and binding proteins in potato led as to identification of six isoforms of 14-3-3 protein, with then unknown function. We showed for the first time its localization in the nucleus and based on research on transgenic plants with overexpression and repression of 14-3-3s proposed that those proteins are involved in regulation of secondary plant metabolism (Markiewicz et al., 1996; Wilczyński et al., 1998).
These studies revealed that flavonoids, amino acids, fatty acids, alkaloids and amines, correlate with 14-3-3 gene expression. Subsequent evaluation of the secondary metabolism in these plants resulted in discovery of various catecholamines including epinephrine, norepinephrine, and dopamine (Kulma & Szopa, 2007; Szopa & Wilczyński, 2002; Szopa et al., 2001, 2009). In addition, these studies suggest that increases in the catecholamine content of GM plants is likely a direct result of 14-3-3 protein mediated upregulation of endogenous tyrosine decarboxylase. This led to further analysis of the catecholamine biosynthesis pathway via several different approaches, including exogenous (from parsley) tyrosine decarboxylase or dopamine receptor (human) expression, which in turn revealed that these compounds are likely to serve the same biochemical functions in plant and humans, i.e., regulating glucose-starch balance and facilitating the stress response in potato plants (Kostyn et al., 2020; Skirycz et al., 2005; Świędrych et al., 2004).
Flavonoids are a widely studied class of antioxidants known to protect against disease by contributing to the total antioxidant defense system used by humans. Thus, while 14-3-3 gene manipulation offers a tangible method for improving potato plant pest resistance there are several other effective approaches for increasing flavonoid content in other types of transgenic potato. These include the use of cDNA transcripts encoding key genes from the flavonoid biosynthesis pathway (chalcone synthase, chalcone isomerase, and dihydroflavone reductase) to produce transgenic plants overexpressing single (chalcone synthase – CHS, chalcone isomerase – CHI, or dihydroflavonol-4-reductase – DFR), double (CHI and DFR), or triple (CHS, CHI, and DFR) transgenes, and thus increasing antioxidant content. These evaluations revealed that the most effective production of anthocyanins relied on the application of a single DFR cDNA construct expressed in the sense orientation (Lukaszewicz et al., 2004; Szopa et al., 2006). In fact, evaluations revealed that changes in anthocyanins production in DFR plants perfectly correlated with changes in the antioxidant capacity of their transgenic potatoes. However, in each case there was a decrease in starch level ranging from a few percent to more than 10 times the control value. This decrease was also accompanied by a significant decrease in glucose and an increase in the level of sucrose as well as a decrease in the overall tuber yield. Thus, these results suggest that a very high concentration of flavonoids may adversely affect carbohydrate synthesis.
Given this, it is important to create novel methods that decrease the negative effects of flavonoid accumulation. Therefore, we applied two novel approaches. In the first, the DFR cDNA was placed under the control of a previously isolated and characterized UV-inducible promoter from the glucosyltransferase gene, and in the second, the plant was simultaneously transformed with the DFR cDNA and a previously characterized cDNA encoding a flavonoid-specific glucosyltransferase thus reducing the reactivity of flavonoids. Both approaches resulted in increased flavonoid content and improved plant productivity (Aksamit-Stachurska et al., 2008; Korobczak et al., 2005, Lorenc-Kukuła et al., 2005). This proved that overproduction of flavonoids is an effective method for plant improvement; however, due to their high reactivity their accumulation needs to be tightly controlled to avoid undesirable side effects.
. Engineered Flax
Flax requires moderate protection while maintaining good growth making it well suited to sustainable agriculture. However, harvesting can be a major problem, especially if the cultivation starts late, or if the plants are incompletely dried or folded. Linseed is also known to suffer from severe lodging, but many plants can recover if lodging occurs at the beginning of the season. In addition, several studies and pilots have revealed that flaxseed yield can be significantly improved via classical crop breeding using cultivars with high performance characteristics, optimization of the cultivation strategy, regionalization of crops, and the introduction of GM crops, particularly drought-resistant variants, with low levels of pectin and lignin. Despite this, there are still some limitations to flax cultivation including complex issues in its growth, a lack of variants with good drought or temperatures resistance, inconsistencies in yield and quality, lack of organic farming technology, and the need for special harvesting machines. However, flax is a historically important crop known for its use in the production of oil and fiber and has long been cultivated as a dual-purpose plant with these fibers commonly applied in the textile industry, paper production, isolation materials, biodegradable packaging material, and bio-composite production. The limitations in their application are primarily linked to their inability to compete with cotton or synthetic fibers, which are thinner, more elastic, and easier to produce. Flax oil is the richest agricultural source of polyunsaturated fatty acids and is known for its various industrial applications including its use as a binder in paint and in printing inks owing to its drying and hardening properties. Several studies also suggest that ingestion of flax oil and whole seeds may provide protection against atherosclerosis and improve resistance to certain types of breast and prostate cancers. However, the use of linseed oil in the pharmaceutical, cosmetic, and food industries is limited by its high susceptibility to oxidation. This exposes consumers to extremely reactive lipid radicals and toxic and carcinogenic compounds such as aldehydes, conjugated diens, and trienes.
In addition, all plant species interacts with other living organisms, acting as the host to various symbiotic and pathogenic microorganisms. Plants are also known to produce chemicals which protect them against infection which may be toxic to both animals and humans. However, plants are sessile and rely on the environment to facilitate seed dispersal which means that their reproduction is contingent on the plants ability to attract pollinators. Thus, plants must maintain a fine balance between deterring pathogens and attracting pollinators, a process that is primarily facilitated by their production of a range of specialized secondary metabolites. For example, cyanogen glucosides from flax are poisonous but their phenylpropanoids and isoprenoids act as color and scent compounds which attract pollinators and act as efficient antioxidants.
Thus, large sections of flax, and other plant research, is dedicated to understanding these pathways and then exploiting metabolic engineering to improve pathogen resistance and increase their industrial and biomedical value. In fact, we developed a novel flax plant (Figure 1) enriched with antioxidants from the phenylpropanoid and isoprenoid pathways, which also accumulates polyamines which makes the plant more resistant to environmental changes including Fusarium infection (Lorenc-Kukuła et al., 2007; Wróbel-Kwiatkowska et al., 2005; Żuk & Szopa-Skórkowski, 2014) while improving its antioxidant production. Similarly, we reported that changes in the lignin and pectin or bioplastic synthesis pathway helped to improve flax fiber quality (Szopa & Wróbel-Kwiatkowska, 2011; Szopa-Skórkowski & Wróbel, 2008; Wróbel et al., 2004; Wróbel-Kwiatkowska et al., 2007), while manipulation of terpenoids resulted in improvements in flax oil composition and stability and increased resistance to pathogens (Boba et al., 2011, 2020).
Modified flax fibers have also been shown to accumulate β-hydroxybutyrate and its polymer both of which are beneficial for composite material and medical product production, with these fibers demonstrating clear benefits in a wound healing assay (Kulma et al., 2015; Skórkowska-Telichowska et al., 2021; Szopa-Skórkowski et al., 2013, 2015; Wróbel-Kwiatkowska et al., 2009). The oil from this engineered flax was also shown to be beneficial for humans providing some protection against atherosclerosis. In addition, bio-refined preparations from both seed residues after oil pressure (seedcake) and stem wastes (e.g., shives) were shown to protect human cells against cancer and inflammatory processes, combat pathogenic microbes, activate skin regeneration, and promote chronic wound healing (Czemplik et al., 2012, 2017). Later, detailed analysis of these fiber extracts uncovered a novel cannabinoid-like compound (Styrczewska et al., 2012, 2015) which was then evaluated by UV, IR and MS which in turn identified this compound as cannabidiol (CBD). Further confirmation of the nature of the compound was based on a biological activity assay which revealed that it activates the specific peripheral cannabinoid receptor 2 (CB2) gene and affects the expression of genes involved in both mouse and human inflammatory processes. In addition, the modified industrial process of fabric production did not affect its CBD activity suggesting for the first time that flax products may be a source of biologically active cannabinoid-like compounds which might influence the immune response. These findings could open up many new uses for linen products, especially fabric as a dressing material with anti-inflammatory and analgesic properties.
In summary, most of the new uses of flax based raw materials were derived from new types of transgenic flax plants. These applications include the use of modified linseed oil to boost human nutrition and skin cell regeneration, the use of seed and shive extract to protect humans against infectious diseases, the application of engineered flax fibers as bandages for the healing of chronic wounds, and as a biocomposite for biodegradable packaging material, scaffolding for tissue engineering, and micronized fibers for drug delivery, amongst others. The most important result of our research into the potential use of linseed products was the development of a novel wound dressing made from flax fibers activated with oil emulsion and refined seed extract. These products were tested in external authorized laboratories and were shown to be microbiologically clean, non-toxic, and non-inflammatory and to promote the regeneration of skin cells making it appropriate for use in humans. The resulting certified product (CE 1435) has been used to treat venous ulcers in humans (with this study being approved by independent bioethics committee). These pilot experiments strongly supported the earlier suggestion that these wound dressings promote the healing of various types of chronic wounds (Czemplik et al., 2012; Skórkowska-Telichowska et al., 2010, 2013). This product was almost commercialized, but the process was stopped because current legislation means that GM plants cannot be used in food or feed and cannot be released into the environment, meaning that these products cannot be registered in Poland or elsewhere in Europe.
Despite this, evaluations of the literature suggest that we still have the largest collection of transgenic flax plants in the world with applications across several industries, including medicine (Hall et al., 2016). However, GM plants are still primarily used as a model to study the integration of different metabolic pathways in response to environmental influences, evaluate their capacity to produce and accumulate a wide variety of metabolites of different natures, and study their potential to help fight climate change.
. Novel Flax
In the course of creating improved crops, the use of transgenesis for biological effect has been strongly outlined as a new concept in biology, especially in plant genome diversification and the development of next generation plants. Flax was among the first crop species to be genetically engineered with researchers inserting genes of potential agronomic value. Herbicide resistant traits have been expressed in flax including chlorsulfuron and metsulfuron methyl resistance, glufosinate-ammonium, and glyphosate resistance (Hall et al., 2016). In the first decades of the twenty-first century, transgenic flax was intensively studied by scientists from the University of Wrocław in Poland, but none of these innovative plants has been registered in Poland or the rest of Europe. The first and only GM flax variety, CDC Triffid (sulfonylurea herbicide resistant variety), was registered in Canada and has been approved for release into food, feed, and the environment. However, before it was released to the market, the variety was deregistered (in 2001) because it was not accepted by key markets such as the EU and Brazil.
One specific transgenesis technology with the potential to revolutionize the use of genetic engineering in agriculture is genome editing. Modern genome editing techniques use enzymes to cut DNA at precise locations, using sequence matching to produce a “double-stranded break” that is then repaired by the cell, leading to a change in the genome. Of the three major genome editing techniques, zinc finger nucleases (ZFN), transcription activator like effector nucleases (TALEN), the very recently developed, clustered regularly interspaced palindromic repeats (CRISPR), and CRISPR elements linked to retrons called CRISPEY, the last category appears to work most effectively and are dominant in the global genome engineering space (Lin et al., 2020; Pennisi, 2020; Simon et al., 2019) However, despite their dominance in the research space no CRISPR edited crop has yet been brought to market in Europe, with this unlikely to change in the near future. CRISPR edited plants have the same challenges as GMOs and restricted market access for these crops is likely to remain in place for some time to come. Despite this CRISPR has some important advantages including the ability to easily target and bind to or cut out desired regions of the genome. However, CRISPR mediated insertion remains a significant challenge in plants. In addition, virtually all gene editing techniques fail to avoid the regulatory hurdles that GMOs face with all modified plants still subject to EU control in terms of GMOs.
All these improvement approaches, rapidly accelerated in recent years, have allowed us to capture a significant portion of DNA sequence variation and thus allowed us to investigate other approaches to improving crop plant production and quality. These evaluations have all revealed that an epigenetic approach to plant manipulation may be a valuable alternative to more classical transgenics, with this being widely supported by comparisons of ancient and modern plant DNAs which show that most of their nucleotide substitutions can be described as C to T and G to A transitions, with these kinds of substitutions commonly associated with the deamination of 5-methyl cytosine to thymine, which is typical of epigenetic changes (Springer, 2013). We therefore suggest that the epigenetic regulatory mechanism may be involved in both domestication and plant evolution and is likely to be of great benefit to plant improvement and commercialization, especially since these modifications may circumvent certain GMO regulations.
It was previously discovered that short antisense oligodeoxynucleotides introduced into mammalian cells were able to reduce the level of their complementary mRNAs likely via RNAi. Several technologies (OLIGO, ODN) based on these finding are being developed as therapeutics for various diseases (Quemener et al., 2020) and recent reports describe the use of these kinds of oligonucleotides to control gene expression at the level of transcription and to modify and/or cleave long DNA fragments at single sites (for a review see Gilbert et al., 2008). Oligodeoxynucleotide mediated transcriptional regulation has also been extensively studied in tobacco models (C. Sun et al., 2005) with OLIGOs reported to readily penetrate plant cells and their nuclei. Complementary to mRNA, OLIGOs lower their target gene expression level and have been used to validate specific observations in mammals. Very recently, we revealed that OLIGO affects genes and genome methylation status, and thus their activity in plants (Wojtasik et al., 2014, 2019). Given this, we postulated that OLIGO could be a valuable approach in plant modification via epigenetic mechanisms.
Epigenetic control is mediated by changes in chromatin structure which are in turn primarily regulated via DNA methylation, histone variation, and histone modification. Environmental stress can cause changes in the DNA methylation, which can then be inherited. This is likely because many stressors such as chilling, metal ions, salt, water stress, and pathogen infection produce reactive oxygen species (ROS) that may contribute to changes in the DNA (strand breaks, modification) and thus pathophysiology.
Figure 2 illustrates the connections between several pure genetic and epigenetic factors like: MET1, CMT1, CMT3, DME, ROS1, AGO1, AGO4, RDR2, histone methyltransferases and acetyltransferases (all leading to chromatin alterations) that are thought to be entirely independent of each other. It is believed that common genetic variants associated with increased resistance to specific stressors including single nucleotide variants and copy number variants together with factors that are acquired during the lifetime of the individual have a significant impact on plant evolutionary improvement. Thus, the development of novel epi-alleles via epigenetic regulation of genes could be useful in crop plant breeding. Indeed, the existence of naturally occurring epi-alleles that affect traits in several plant species is already widely accepted (Richards, 2006) with many epigenetic variants known to exhibit relatively stable behavior over hundreds of years, with occasional reversions (Cubas et al., 1999).
Figure 2
A schematic illustration of the complex genetic and putative epigenetic factors that contribute to environment resistance causation; epi-genes: the genes coding for enzymes and factors leading to chromatin alterations.
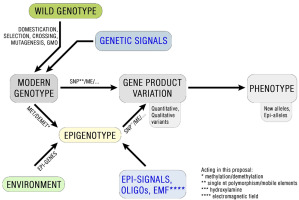
Taken together, this information clearly suggest that epigenetic regulation contributes to plant genome evolution and perhaps might help genome diversification within plant species (Dzialo et al., 2017, 2019; Peng & Zhang, 2009; Sahu et al., 2013).
OLIGO activity is likely mediated via an epimutagenesis signal triggered in response to its binding of the genomic double-stranded DNA and generation of triple DNA. Triple helices have been known to form in vitro and were first observed in homopolyribonucleotides, then polydeoxyribonucleotides, and finally in hybrids (Felsenfeld et al., 1957; Morgan & Wells, 1968). The binding of short oligodeoxynucleotides to sequences of double-helical DNA has also been previously described (Arnott & Selsing, 1974). Furthermore, we suggest that this triple DNA signal triggers the recruitment of DNA methyltransferase, which was then supported by our discovery that 3-hydroxybutyrate activates Met3 methyltransferase (Mierziak et al., 2020). Therefore, co-administration of 3-hydroxybutyrate with a targeted OLIGO is likely to accelerate its action. In addition, the action of OLIGOs can be further accelerated by activating the transport between the cytoplasm and the nucleus, with this being achieved by complexing OLIGOs with a seven amino acid highly positive nuclear localization signal (PKKKRKV large T antigen SV40) and introduction into the plant under neutral pH and weak ionic strength conditions by vacuum infiltration. This method called OLIGO technology has recently been patented (Żuk et al., 2021) and applied to flax plant modification. CHS silencing and β-glucanase overproduction protect flax against Fusarium infection and valorized plant raw products. The addition of OLIGO enabled us to suppress or activate the transcription of specific genes in this process, with these changes being accompanied by more permanent changes in the gene and genome methylation pattern (Dzialo et al., 2017; Wojtasik et al., 2014, 2019).
Taken together, oligodeoxynucleotides (OLIGO, ODN) seem to be the most promising technique for genome diversification, producing plant variants with increased productivity and product quality, and more importantly, increased commercialization potential without the limitations associated with more classically modified GMO plants. Very recently OLIGO technology was used to create novel flax variety, Silesia (Zuk et al., 2021). The ratio of ω-6 to ω-3 in oil from Silesia seeds was about 1.5:1, which is quite close to that recommended for the human diet (Simopoulos, 2008). Additionally, these changes also significantly improved oil stability likely due to increased presence of various flavone and carotenoid antioxidants. The conjugated diene formation in oil oxidized at 140 °C was very low when compared to other commercially available flax oils. Its peroxide value, which indicates the level of primary lipid oxidation, dropped significantly (3.5-fold decrease) after oil oxidation when compared to those available on the market and these products were also shown to be enriched for ornithine, citrulline and 3-hydroxybutyrate. These products have been shown to have some beneficial effects on the cardiovascular health of both adults and children (J. Sun et al., 2021). In addition, nutritional rescue of the levels of beta-hydroxybutyrate inhibited the renal Nlrp3 inflammasome, resulting in improved renal function and decreased blood pressure (Chakraborty et al., 2018). To the best of our knowledge, this was the first report on the epigenetic manipulation of the fatty acid pathway in plants. The flax generated using these manipulations appears to be a remarkable source of health-promoting oil and is now available through the Linum Foundation (Polish: Fundacja Linum; http://www.leczenielnem.pl/). Taken together, this data suggests that while there are still hurdles to the production of novel plant variants both the application of classic transgenesis and epigenetic manipulation are likely to be key factors in crop plant evolution in the future.