. Prologue
We are standing in the courtyard of the castle in Otmuchów (Opole Silesia), owned by the von Humboldt family before the war. On the left, in the place of the old western wing of the castle, there are trees in a small park, whose foundations and species composition were supervised by none other than Alexander von Humboldt, the creator of the concept of a natural monument. On the southern castle-hill slope, there is a broad-leaved forest; at the bottom, along the stream and in the moat, there is a riverside ash stand, and finally on the walls of the castle there is the thermophilic chasmophytic vegetation of Cymbalaria muralis of Mediterranean origin. The vegetation is varied and distinct, with its own specificity, characteristic features, and certain habitat requirements. It is, of course, immensely beautiful in its harmony, richness, and diversity. Alexander von Humboldt must have loved similar views. Approximately 220 years ago, he depicted the vegetation of Mount Chimborazo in Ecuador in his prominent work Essai sur la géographie des plantes [Essay on the geography of plants] (von Humboldt, 1807) as well as in letters to his brother Wilhelm sent to Otmuchów (formerly Ottmachau) (von Humboldt, 1880).
. Geobotany – Combining Botany and Geography
Despite sometimes being confused with geological sciences describing the response of plants to their geological environment (e.g., Haldar, 2013) (which can certainly be extremely important, as it is for serpentine species), geobotany is generally considered to be a science that connects vegetation and plants to space and time (Frey & Lösch, 2010). The term “geobotany” is derived from the geographical sciences and was originally applied to the study on the distribution of plants and patterns of vegetation. But in recent years its scope broadened in the contexts of both theoretical knowledge and practical applications. It includes, among others, floristic geobotany (the study of the geographical distribution of plant taxa), sociological geobotany (vegetation science, plant sociology, phytosociology, and phytocoenology), ecological geobotany (plant ecology, ecophysiology, population ecology, and synecology), and historical geobotany, which deals with the origin and development of populations and communities (Mueller-Dombois & Ellenberg, 1974). Therefore, without going into the details of many definitions and approaches (see, e.g., Grisebach, 1866; Kornaś & Medwecka-Kornaś, 2002), it can be assumed that geobotany is a modern phytogeographical discipline that deals with the composition, development, spatial distribution, and environmental relationships of plants and their communities. It focuses on how plants and vegetation function in the environment and on examining the effects of environmental factors (including anthropogenic factors) on plant populations and communities. This last role of geobotany as an applied science is the foundation for the conservation of vegetation and habitats.
. Geobotany – A Humboldtian Child
As we read the letters von Humbold wrote to his brother Wilhelm in Otmuchów (Figure 1), we recall our contemporary geobotanical expeditions and conclude that, despite the passage of 200 years, there is little difference between them. While sailing the Orinoco River by pirogue he wrote: “Regarding the woods; they are so dense on the river side, so entwined with lianas, that it is impossible to get through (October 1800).” (von Humboldt, 1880, p. 18).
Figure 1
The castle in Otmuchów (SW Poland) owned by the family of von Humboldt until 1929 (Phot. A. Nowak, July 2021).
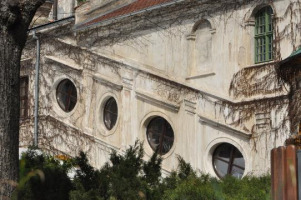
Additionally, he wrote:
A herbarium of mine has also remained in Havana with duplicates sent with the help of the monk Fr. Juan Gonzalez via North America, Spain, and La Rochelle. Moreover, I sent additional doublets with the botanist James Fraser to London and Berlin. These plants, intended for Mr. Willdenow himself, have already arrived happily in London.
(von Humboldt, 1880, p. 23)
During his trip to Colombia, he wrote:
I hope to see the great botanist Don Jose Celestino Mutis, who was a friend of Linne and lives now in Santa Fe de Bogota, and to compare our plant collections with his own. I hope to cross the immense Cordillera of the Andes, which extends from Lima towards the north to the mouth of the Atrato River (into the Gulf of Darien) and found Laurus cinnamomoides Mutis. The famous almond tree (Caryocus amygdaliferus) can also be found here. I found forests of China trees and the otoba, the true Myristica (Nutmeg).
(von Humboldt, 1880, p. 32)
These words sound remarkably similar to the those in reports of geobotanists conducting research expeditions in the present. It is not surprising that the International Association of Vegetation Science (IAVS) has funded the Alexander von Humboldt Medal for outstanding vegetation scientists (including both typical geobotanists and ecologists, such as Pierre Legendre, Stuart Chapin III, Sandra Lavorel, G. David Tilman, or J. Philip Grime).
Alexander von Humboldt was an aesthete who admired, but also carefully observed the beauty, diversity, and patterns of nature. He was an artist with a well-developed sense of technical accuracy, especially in the physical measurements of the environment. To this day, these are the characteristics of every skilled geobotanist. Indeed, following Humboldt’s example, Meyen (1836) emphasized the role that vegetation plays within Man’s aesthetic appreciation of Nature:
Baron Alexander von Humboldt’s celebrated work (“Considerations on the Physiognomy of Plants”) pointed out this highly interesting side from which botany may be viewed and how it may improve the taste of nations by increasing their sensibility to the beauty of nature and thus have an influence on the progress of the art.
In addition, Hult (the Swedish plant geographer) shared Humboldt’s standpoint that the plant geographer should cultivate an aesthetic as well as scientific link to vegetation. In his article, Hult traced the history of the study of vegetation types, from its origin in the works of Alexander von Humboldt, through the works of Grisebach and Kerner von Marilaun, to his own time (Hult, 1881).
Humboldtian plant geography was the first to reflect concern with vegetation as an autonomous object of inquiry. Unlike floristic plant geography, it was not only concerned with species, genera, and families. Vegetation types are the central research focus. Hult credited Humboldt as being the first to recognize the existence of the collective groupings of plants and creating a vegetative plant geography: “It needed Humboldt’s mighty spirit to discover these groups and to realize their immense significance in the geography and history of the Earth” (Hult, 1881). Indeed, Alexander von Humboldt was undoubtedly an outstanding geobotanist, deeply interested in the physiognomy and patterns of vegetation, as well as its relation to environmental parameters (Nicolson, 1987).
Humboldt wrote in his Personal Narrative of Travels (von Humboldt & Bonpland, 1822):
I was passionately devoted to botany … and I flattered myself that our investigations might add some new species to those which have been already described but preferring the connection of facts which have been long observed to the knowledge of insulated facts, although they be new, the discovery of an unknown genus seemed to me far less interesting than an observation on the geographical relations of the vegetable world, or the migration of social plants, and the limit of the height which their different tribes attain on the flanks of the Cordilleras.
Figure 2
The Chimborazo Mt with the original notes of Alexander von Humboldt depicting the patterns of vegetation and highlighting both the dominant species in plant communities and environmental data (from Ideen zu einer Geographie der Pflanzen nebst einem Naturgemälde der Tropenländer by von Humboldt and Bonpland, 1807).
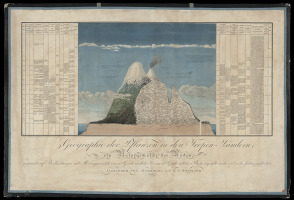
Humboldt is considered the first researcher to call, although informally, distinguishable plant communities the associations (Nicolson, 1996).
Alexander von Humboldt laid the foundation for a science that searched for vegetation patterns on a macro scale and studied the relationship between environmental characteristics and the distribution of plant communities on Earth. For Humboldt, it was obvious that vegetation can be classified into types related to topography, biogeography, and human activity. He considered vegetation geography a branch of natural history, so he introduced an evolutionary perspective to the science of vegetation (Nicolson, 1996).
As geobotany began to develop rapidly, its prominent figures, such as Grisebach, started emphasizing physiognomic aspects, finally defining the term “geobotany” (germ. Geobotanik) as a scientific discipline and introducing the traditional ending “-etum” to names of formations and associations (Grisebach, 1866). Grisebach’s great achievements include the monumental work Die Vegetation der Erde [The Earth’s vegetation according to its climatic arrangement. An outline of the comparative geography of plants], which provided the first comprehensive description and classification of the world’s vegetation (Grisebach, 1872).
The Humboldtian geobotanical approach was also developing intensively in Poland. Józef Paczoski (1864–1942) (Figure 3) is its precursor and an excellent promoter (Maycock, 1967). He is considered the founder of the name “phytosociology” (Kozłowski & Swędrzyński, 2009; Latowski, 2014). Initially, Paczoski (Pachoskiĭ, 1891) in his work “Стадии развития флоры” [Stages in the development of flora] called it “florography,” and introduced the current term “phytosociology” in the work Życie gromadne roślin [Social life of plants] (Paczoski, 1896/1930) (Figure 4). He defined plant association as a homogeneous fragment of vegetation as the basic object of research. He discussed the structure and developmental processes of plant associations and defined the scope of phytosociological research. He began the world’s first lectures on phytosociology at the Faculty of Agricultural Sciences of the Kherson University of Technology (Ukraine) and soon published the textbook Social life of plants (Paczoski, 1896/1930). Paczoski was one of the first researchers to recognize the importance of plant relationships with underground fungi and microorganisms. He understood that vegetation is highly dependent on the underground world and was convinced that phytosociology should also focus deep into the soil environment. Paczoski’s thoughts were continued by generations of Polish geobotanists and plant geographers, including prof. Jan Kornaś and prof. Anna Medwecka-Kornaś, founders of the Kraków school of phytogeography, Władysław Matuszkiewicz, author of the fundamental work on plant communities of Poland, Janusz Bogdan Faliński, an outstanding plant ecologist and phytogeographer, Janusz Matuszkiewicz, a prominent phytosociologist, or Zbigniew Dzwonko, an excellent plant ecologist, to mention just a few (Dzwonko, 2007; Faliński, 1990; Kornaś & Medwecka-Kornaś, 2002; J. M. Matuszkiewicz, 2008; W. Matuszkiewicz, 2021). It is worth mentioning that Władysław Matuszkiewicz and Janusz Faliński founded and were the directors of Białowieża Geobotanical Station, a center of long-term studies on plant communities, vegetation succession, and seasonal changes in primeval forest vegetation of European lowlands (Borkowska, 2013).
Figure 4
The reprint of the Paczoski’s work Życie gromadne roślin [Social life of plants] published originally in Wszechświat in 1896.
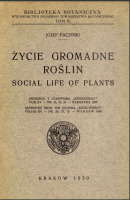
The achievements of Russian geobotanists in the development of the discipline were also not overlooked. For example, the works of Tikhon Rabotnov, who was the head of the Department of Geobotany at Moscow State University, on the regeneration, dynamics, and structure of vegetation in grasslands are still considered significant achievements (e.g., Rabotnov, 1975, 1980).
In the second half of the nineteenth century, geobotany developed rapidly in Switzerland. Carl Schröter (1855–1939) was a well-established ecological researcher, excellent phytogeographer and phytosociologist. He introduced the concept of “autecology” to explain the relationship between an individual plant and its external environment and “synecology” to express relationships between plant communities and external influences. Likewise, the Swedish botanist Rutger Sernander (1866–1944), under whom the Uppsala school was founded, had already started applying quantitative methods of vegetation studies in the mid-1880s.
Figure 5
Drawing of a commemorative plate founded on the initiative of the Polish Botanical Society on its hundredth anniversary, commemorating the figure of Josias Braun-Blanquet (prepared by Odlew-Art, Opole).
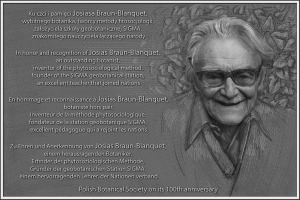
Schröter’s student Eduard Rübel (1876–1960) further developed the Zürich school of geobotany, which focused mainly on bryophytes. At the same time, Rübel collaborated with Braun-Blanquet (1884–1980) (Figure 5). In 1918, he founded the Rübel Geobotanical Research Institute. It was this geobotanical “atmosphere” that Josias Braun-Blanquet grew up in, and he was very diligent in establishing the new discipline. The foundation of a phytosociological classification system by J. Braun-Blanquet and the definition of character species, which took place in 1921, strengthened phytosociology. It was also enhanced by the definition of a differential species by W. Koch, which occurred in 1926. Later publications by Thienemann (1926) and Clements (1916, 1936) related to new ecosystems or providing theoretical foundations for analyses of changes and dynamics of vegetation, were also important for the development of phytosociology. As another exceptionally important contribution to the development of phytosociology, J. Braun-Blanquet established the SIGMA station (Station Internationale de Géobotanique Mediterranéenne et Alpine) in Montpellier, which disseminated and spread the new discipline and its methods throughout the entire botanical world. The students of Braun-Blanquet included Erwin Aichinger, Oriol de Bolòs, Frank Egler, Heinz Ellenberg, Jaromir Klika, Erich Oberdorfer, Bogumił Pawłowski, Werner Rothmaler, Reinhold Tüxen, and Maks Wraber. Josias Braun-Blanquet was the founding editor of Vegetatio (currently Plant Ecology) in 1948 (along with W. C. de Leeuw and R. Tüxen), retaining an editorial leadership role until 1974. The followers of the Zürich-Montpellier School of Phytosociology established a number of scientific journals, including the Journal of Vegetation Science, Applied Vegetation Science, Tüxenia, Castanea, Plant Biosystems, Geobotanical Studies, Acta Societatis Botanicorum Poloniae, Folia Geobotanica, and many others. The floristic-sociological approach is still very powerful in surveying and interpreting vegetation. It is also an effective tool for capturing and describing the variability and dynamics of vegetation (Mucina, 2010). Braun-Blanquet’s monographic textbook (Braun-Blanquet, 1928) is still being updated and cited in various countries (e.g., Dzwonko, 2007; Frey & Lösch, 2010).
. Geobotany – The Applied Science
From the very beginning of its existence, geobotany was characterized by a practical attitude. In the times of Humboldt, researchers were looking for answers to ethnobotanical questions about the relationships between tribes and the vegetations they inhabit. It is impossible not to mention the invaluable application of geobotany in nature conservation, vegetation diversity analyses, or the assessment of the quality of habitats and patterns of vegetation or plant distribution on a regional or global scale. The commonly used method of geobotanical mapping (see Faliński, 1990) and assessment of the quality of plant communities consistent across many countries was enabled by the European Natura 2000 network (Council of the European Communities, 1992). It was an excellent choice, if not the only one, that allowed the effective conservation of natural habitats (Directorate-General for Environment, European Commission, 2021). The same applies to summarizing works on the distribution of plants and species checklists, without which it wouldn’t be possible to protect effectively plant diversity (see Jackowiak, 1993, 1998; Mirek et al., 2020; Zając & Zając, 2001, 2019).
Leaving aside the undoubted theoretical achievements of geobotany, it is worth noting that this discipline is exceptionally effective in application studies and thus is quite similar to pharmacy (linked to more theoretical chemistry), medicine (linked to biology), forestry, ecological engineering (linked to botany and ecology), and horticulture (linked to botany). The Applied Vegetation Science (AVS) and even the Journal of Vegetation Science (JVS) are of a particularly applicative nature, with many papers dealing with the conservation, mapping, and management of the vegetation. As might be expected, “conservation” is the most common subject or aim of research, with 1,172 papers in the AVS and 1,377 in the JVS dealing with all aspects of effectiveness, methods, prioritization, restoration, or legal issues (e.g., Koyama et al., 2021; Laumonier & Nasi, 2018; Nicod & Gillet, 2021; Sitek et al., 2020; Volkova et al., 2020). In addition, more regional journals that include a geobotanical section are a forum of conservation topics (274 papers in Acta Societatis Botanicorum Poloniae; ASBP). “Management” is fairly well represented among research topics (974 in the AVS, 1,766 in the JVS, and 186 in the ASBP) (e.g., Czarnecka, 2020; Giallonardo et al., 2019). “Mapping” still remains one of the most commonly used keywords in the context of application (547 and 1,385 papers, respectively, in AVS and JVS), and in the last decade has referred mainly to low-altitude aerial photography, object-based image analysis, satellite imagery, or molecular phytogeography published also in ASBP (Andres-Mauricio et al., 2021; Kosiba et al., 2011; Ronikier et al., 2018; Uyeda et al., 2020). “Vegetation mapping” is still central to geobotanical surveys, with 535 papers in the AVS and 1,332 in the JVS (e.g., Kindt et al., 2014; Vaca et al., 2011).
When considering geobotany as an applied science, we must not forget that in a globalized world, we have probably learned about no more than 30% of the vegetation diversity at the association rank, and perhaps only 10%–15% has been mapped, while a large part of vegetation is under the serious threat of disappearance or imminent decline from human activity and climate change (rough estimate on the basis on the sizes on continents and intensity of geobotanical surveys) (Berg et al., 2014). Hence, geobotanists are faced with the tremendous task of completing the work of Humboldt and Braun-Blanquet (and other founders) and the classification, mapping, and effective preservation of the diversity of the Earth’s vegetation. There are other fascinating questions. Many interesting research topics emerge in relation to both the past and future. For example, we still do not know what kind of vegetation was the archetype of the Garden of Eden. Our last geobotanical investigation, wherein we analyzed the functional, taxonomic, and utility features of Mediterranean and Irano-Turanian vegetation, led us to the conclusion that a Pistacia khinjuk grove from the south of the Zagros Mountains in southwestern Iran was the archetype of the Garden of Eden (Nowak et al., in press). Of course, there are many other questions regarding the range or area of occupancy of a specific type of vegetation and its tendencies yet to be answered. Tropical, subtropical, and marine vegetation must be targeted. Only Europe has been comprehensively surveyed in terms of the diversity of plant communities, but other continents are still underinvestigated and awaiting comprehensive analyses (Mucina et al., 2016).
Geobotany is an applied science with a solid theoretical background in ecology, botany, geography, phytogeography, physics (e.g., remote sensing), and even molecular sciences (e.g., metabarcoding). In this sense, geobotany cannot compete with theoretical ecology and cannot be compared with it. It is difficult to imagine that any other specific discipline could answer questions about the dynamics, diversity, and distribution patterns of vegetation on a macro scale. For this reason so many phytosociologists and geobotanists are active in macroecological research. Therefore, geobotany will always be a much-needed discipline for ecologists who are particularly interested in effective applications, sensitive to the beauty of plants and vegetation, and convinced of the need for its protection. Mirkin (1989) stated that:
The basis of syntaxonomy is entirely conventional owing to the continuous nature of vegetation. As a result, the scope of the association concept varies with different national schools, and the hierarchy in syntaxonomy is pragmatic, at best of an ecological nature.
The conventional nature of vegetation units indicates that the decision to adopt one method or another is based on conventions. Moreover, adopting formalized procedures is imperative for conventions in a consistent way.
. Geobotany – Going Below-Ground
Almost all studies have focused on the classification of vegetation, temporal, and spatial changes in vegetation cover, community assembly, and development or exploration of the influence of environmental variables on vegetation, considering only the above-ground components of easily observable plant species (e.g., Chytrý, 2013; Chytrý et al., 2015; Dengler & Boch, 2008; Wagner et al., 2017). In recent years, several cutting-edge studies have shown that, depending on the geographical location, below-ground plant richness can range from 50% to 100% higher than the above-ground plant richness (Pärtel et al., 2012; Träger et al., 2019). In many ecosystems, the majority of vegetation (e.g., 50%–90% in temperate grasslands) occurs below ground as roots, rhizomes, and shoot bases (Matesanz et al., 2019).
Whether the diversity patterns described for above-ground vegetation also hold for the large below-ground component has yet to be fully explored (Pärtel et al., 2012). Despite significant progress in the exploration of below-ground diversity, most ecosystems have not yet been studied. Most studies have focused on single types of vegetation, with relatively scarce sampling, small size of soil samples, and molecular analysis performed only for the below-ground part of the phytocoenoses with the use of a single barcode, mostly the P6 loop of the trnL region of cpDNA (Hiiesalu et al., 2012; Li et al., 2015; Matesanz et al., 2019; Träger et al., 2019). This situation is similar to writing a poem with only half of the available letters. Getting better compositional data during standard surveys is hampered by many factors (Gaston & O’Neill, 2004), some important factors are: (i) limited access to taxonomic competence (Gaston & O’Neill, 2004; Janzen, 2010) especially regarding diagnosis of roots; (ii) the seasonality of vegetation cover along with an often limited time window available to sample individuals that can be identified using morphological characteristics, for example floral characteristics of plants (Dexter et al., 2010); (iii) the underdevelopment of species in a highly competitive neighborhood – for example, decayed green part of the plant despite the presence of a small seedling and roots; (iv) destruction of species by animals and humans; (v) problems with identification of cryptic species or hybrids; (vi) periods of dormancy that prevent species from being found despite thorough investigations undertaken even for several years. Shoots may also be missing in some years but present in others (Reintal et al., 2010; Shefferson, 2009), resulting in >30% variation in above-ground richness between years in natural vegetation (Pärtel & Zobel, 1995; Price et al., 2012; Wilson, 2007; Wilson & Tilman, 2002).
DNA metabarcoding of environmental samples has already been suggested as an alternative or complementary method to traditional approaches in fields related to biodiversity estimation (De Barba et al., 2014; Parducci et al., 2013). Owing to considerable cost reduction and improved bioinformatic analyses, the recent development of DNA metabarcoding has improved the efficiency and reliability of mixed-species samples (Herben et al., 2017; Hiiesalu et al., 2012; Li et al., 2015). Methodological limitations have been identified and both field data collection and laboratory analysis have improved, which has made it possible to increase the accuracy of estimates of the proportion of the above-ground and below-ground parts of phytocoenoses (Rucińska et al., 2021, 2022). This provides a great opportunity to discover or refine patterns and relationships in below-ground communities. Considering how heterogeneous, species-rich, and interdependent below-ground habitats and communities are, this opens up a wide frontier of research not only for vascular plants, but also for fungi, animals, and microorganisms that interact with plants in various ways. These studies have great potential to increase our understanding of the ecology of plant communities and help to answer important questions such as: (i) What is the difference in species richness and diversity between the below- and above-ground components of plant communities? (ii) What are the most important environmental drivers of the differences in the diversity of the below- and above-ground parts of the plant community? (iii) What is the influence of fungi–plant interactions on the difference between below- and above-ground diversity? (iv) How does anthropogenic disturbance affect the above-to-below-ground ratio of a given plant community? (v) What is the relationship between the above/below richness and diversity ratio in different habitats and biogeographical regions? How does below-ground diversity affect the species–area relationship? (vi) How can the difference in species composition of the above- and below-ground parts of phytocoenoses influence the classification of vegetation types? (vii) What is the extinction credit in particular habitats when examined with below-ground evidence of species composition? (viii) What is the diversity and richness of the soil seed bank in relation to the above/below-ground ratio of species richness and diversity of the vegetation? (ix) How do management intensity or harsh environmental conditions affect the above/below ratio in different plant communities? A few of these issues have been partially answered for Central European grasslands, but the majority are still waiting to be addressed (Rucińska et al., 2021, 2022).
An interesting perspective in the study of below-ground biodiversity is provided by the use of physicochemical methods to visualize the architecture of plant roots below the ground. These hot methods of rhizosphere exploration include magnetic resonance imaging (MRI). It allows precise measurements of the root system architecture without the need for expensive experimental tools such as hydroponics, rhizotrons, or destructive soil coring (van Dusschoten et al., 2016). An integrated imaging system called growth and luminescence observatory for roots (GLO-Roots) is an equally promising method. It uses luminescence-based reporters to enable studies of root architecture in a soil profile (Rellán-Álvarez et al., 2015). Additionally, the use of X-ray micro-computed tomography with 3D imaging provides highly accurate images of the root distribution in the soil at a resolution that allows single species identification (Flavel et al., 2017; Teramoto et al., 2020). Gregory et al. (2003) captured 90% of a 7-day-old Triticum aestivum crop, and Perret et al. (2007) retrieved approximately 87% of the total root segments and 78% of the total root length in Cicer arietinum.
. Geobotany – Focus on the Absence
The concept of dark diversity is a promising approach for prioritizing conservation efforts, as it focuses on species that are present in the regional pool but locally absent, even though environmental requirements are met (Moeslund et al., 2017; Pärtel et al., 2011). The notion of dark diversity can provide an effective tool for biodiversity management measures (Lewis et al., 2017; Pärtel et al., 2011). In fact, species suspected to be a part of dark diversity are rare or retreating, and their biological and ecological traits make their recruitment and persistence in a given location unsure. There is still no comprehensive data on the traits in which habitats cause plants to be missing locally (despite being a part of a regional species pool), but the most important are seed dispersal, seed germination, seedling emergence, establishment, metapopulation and metacommunity dynamics, mycorrhizal relationships, allopathic activity, pollinator dependence, competitive potential, etc. Employing the concept of dark diversity, it is possible to estimate the community completeness index, which is undoubtedly a useful tool for assessing the composition and conservation value of a plant community (Pärtel et al., 2013). Could it be more important for nature conservation to answer the question of why a certain species is missing in a given place? The idea of dark diversity is still expanding its influence, but much remains to be discovered, both theoretically and practically. For example, we do not know the relationship between dark diversity, soil seed banks, and hidden diversity (below-ground parts of plant communities). The vast majority of world regions and habitat types have not been studied for the relationship between regional species pools and local diversity; hence, new projects and great efforts in this area are urgently needed.
. Geobotany – The Science of Numbers
Numerical competence is a cognitive ability that is widespread across the animal kingdom and is especially developed in primates and humans. The ability to discriminate the number of elements in a set, called numerosity, is indispensable in discerning differences, sizes, and dynamics (Nieder, 2020). Therefore, modern geobotany and vegetation science employ a massive number of numerical methods to compare vegetation features, estimate their changes, analyze the population sizes, model species ranges, calculate the richness and diversity in a sample, manage huge databases, and analyze the functional attributes. First, in order to work digitally, we must convert the cover of species to a percentage or some other number based on the applied scale (e.g., Braun-Blanquetian). Later, this value is most often computationally transformed to obtain the best fit and decrease the effect of the dominant species (Tichý et al., 2020). Numerical classification methods are essential data analytical tools in vegetation ecology (De Cáceres et al., 2015). Plants and vegetation, in all their complexity, are certainly not easy objects for mathematical description. However, the latest tools enable many types of analyses on large datasets. This applies, inter alia, to distribution modeling, phytogeography, phylogeny, phylogeography, life-history traits, taxonomic and functional diversity, species co-occurrence, and environmental data in studies on vegetation. Presenting vegetation is particularly crucial when dealing with different indices, such as alpha- and beta-diversity, evenness, similarity, dominance, frequency, relative frequency, and the statistical significance of the result (Morris et al., 2014; Pitkänen, 1997).
The IAVS has established a special working group for ecoinformatics to facilitate access to big data, as well as its analysis. In particular, its members are active in establishing standards for data exchange, providing tools for data identification, access, integration, storage, and analysis. The numerical approach has dominated the classification and ordination procedures in multidimensional space over the last few decades and is supported by a number of software (Mucina, 1997). The often expressed need for a common language and formalization of classification procedures calls for the adoption and standardization of computer-aided algorithms (Mucina, 1997). One of the applications of mathematics in phytosociology is fuzzy logic, which is useful for coding and describing overlapping clusters in a nondeterministic approach to clustering (Moraczewski, 1993a, 1993b). Despite being considered a promising research method for analyzing vague vegetation units, its use is still not widespread enough. Currently, the analysis of large databases, consistent classification, and a formalized approach to defining vegetation types and establishing expert-based clustering require a numerical approach and a formal protocol.
A multitude of software and applications for computing vegetation data have been released, for example, the JUICE program (Tichý, 2002) designed for the editing, classification, and analysis of large phytosociological tables and databases. This software includes many functions for classification using the COCKTAIL, ISOPAM, and TWINSPAN methods. MULVA-5 was designed to apply multivariate statistical methods to vegetation and site data and derive simple models from relevé and site data (Wildi & Orlóci, 1996). The TURBOVEG software was developed by Hennekens and Schaminée (2001) for processing and managing big datasets of phytosociological relevés. Additionally, the VEGANA (VEGetation edition ANAlysis tools) program, written in Java, was proposed for editing and analyzing community data (De Cáceres et al., 2003). A numerical approach was also used to ensure the possibility of automatic identification of vegetation type (De Cáceres, Font, & Oliva, 2010; De Cáceres, Legendre, & Moretti, 2010). At the beginning of the twenty-first century, a number of R packages were written, with a particular focus on numerical analyses of vegetation. One of the most important is the vegan package, which contains ordination methods, diversity analysis, and other functions (Oksanen et al., 2008). The vegclust package provides functions for maintaining plot-based community classifications using fuzzy clustering methods with three available options: fuzzy C-means, noise clustering, and possibilistic C-means. Additionally, the mclust has been developed to find the best correspondence between classifications and calculate the adjusted R and index (Scrucca et al., 2016).
In the last decade, many vegetation scientists have implemented a large set of new statistical and numerical methods and techniques using different software. Further developments and adjustments to the R environment, related to assigning a vegetation plot to a given vegetation type by the Expert System, have been proposed (Bruelheide et al., 2021). This formal approach in R language is particularly applicable to large vegetation-plot databases because it effectively handles logical vector-wise operations across all plots, allowing for the evaluation of membership expressions and formulas. These formulas can be produced as a machine-written result, for example, as the output of classification results (Bruelheide et al., 2021). Machine learning algorithms have already been applied to understand the patterns and potential drivers of forest diversity (Luo et al., 2021). Additionally, a new semiautomatic machine-learning method called GRoup IMProvement (GRIMP) was developed to optimize groups of species used for discriminating between vegetation types in expert systems (Tichý et al., 2019). Vegetation scientists have focused on the continued improvement of cluster homogeneity in vegetation classification. The quality of the partitioning can be assured by reallocation-based methods of optimization of the value of a particular index of goodness by iteratively changing the initial clustering. A new effective tool for calculating silhouette widths, which measures the goodness-of-fit of plots to their cluster using the iterative reallocation clustering method (REMOS), has been proposed recently (Lengyel et al., 2021). The number of assigned clusters was also examined to obtain optimal clustering in relation to the abundance transformation power exponent, thus rendering the classification effective and stable (Lengyel et al., 2018).
The conspecific-encounter index and Moran’s index were tested to distinguish between overlapping and aggregate distribution at the community level. This new numerical simulation can be related to the aggregative rules, distribution, community stability, extinction of species, and influence on species–area relationships in plant communities (Chen et al., 2021). To identify the best functional predictors of community assembly, we can reveal the underlying environmental and biotic factors, even if they are hidden, unmeasured, or not accessible at the spatial or temporal scale (e.g., the leaf economic spectrum). This method compares two matrices of fuzzy-weighted trait similarities of species and the matrix derived by Beals smoothing using the probabilities of species co-occurrences (Pillar et al., 2021).
. Geobotany – Mapping From Above
Mapping vegetation is crucial for managers, conservationists, and scientists because it provides the basis for natural resource management, ecological modeling, and climate change studies (Faliński, 1990; Su et al., 2016). The typology of vegetation is still not sufficiently advanced over vast areas of the globe. Airborne LIDAR devices may be considered a useful method for the exploration of extant and distant areas. Recently, a semiautomatic approach to vegetation mapping with LIDAR using hierarchical object-based classification with a rule-based expert system has been developed (Uyeda et al., 2020). Light detection and ranging foster the acquisition of structural and compositional data from forest stands and efficiently help in vegetation mapping at large spatial scales. It is possible to determine vegetation types and determine their distribution patterns using the Bayesian information criterion algorithm and unsupervised classification techniques (Su et al., 2016). With the use of multispectral and hyperspectral data, owing to the strong penetration capability of a narrow laser beam, it is possible to examine the internal structure and, to some extent, the species composition of a particular plant community and produce accurate maps at fine or coarse scales (Adam et al., 2010). LIDAR surveys are not only a very fast and accurate tool for understanding the distribution of vegetation types (see Beamish et al., 2020), but also serve to measure biomass, the range of the habitat of a selected plant species, or to assess the degree of degeneration or quality of habitats. It can also be used to measure selected individual features such as tree height or diameter (Huang et al., 2011; Su et al., 2016) or leaf area index, leaf life-span, leaf mass per area, photosynthetic capacity, dark respiration rate, and nitrogen and phosphorus content (Kattenborn & Schmidtlein, 2019). It surveys the canopy cover (Korhonen et al., 2011), stand volume (Naesset, 1997), phenology phases of phytocoenosis (Salas, 2020), and successional stages on industrial lands (Woźniak et al., 2021) with high accuracy. Airborne LIDAR systems have recently been used to monitor biodiversity in different ecosystems. They can detect wildfires, timber harvesting, and biases in community structure (deviation from standard height, density, and cover) at a spatial resolution of 30 m (Guo et al., 2017). Multispectral and LIDAR data can reveal fine textural and structural features, and can be an effective tool for detecting the target individual species. It has been successfully used to monitor and assess the population size and occupation area of invasive species such as Pueraria montana (Liang, et al., 2020). The still-increasing accuracy of air-born LIDAR technology, decreasing device prices, and a wide range of applications in life sciences, including geobotany, make it one of the most promising vegetation mapping tools in global surveys.
. Geobotany – Looking to the Past and Future Patterns of Vegetation
Modern geobotany can also use palynological diagrams and archaebotanical data to provide insight into past vegetation cover. Szafer (1935) was the first to develop a pollen-derived analytical map of Poland’s vegetation based on 152 sites (so-called iso-pollen maps). A more general and prominent work, an Atlas of Past and Present Pollen Maps for Europe, was published by Huntley and Birks (1983) and is still a valuable source for understanding vegetation change in the Holocene at the subcontinental scale. The European Pollen Database (created in 1992), North American Pollen Database (NAPD), and other sources serve as an effective tool for reconstructing the Quaternary vegetation of the Northern Hemisphere and its changes (Giesecke et al., 2014). It enables the assessment of the intensity of human land-use in Europe and man’s impact on medieval forest clearance, the migration period, the Iron Age, the Bronze Age, and the Neolithic period. Very successful attempts have been made to map the ancient vegetation of North America over the past 2,500 years, with the use of fossil pollen in sediments and applying the Bayesian approach to understand spatiotemporal trends in forest composition (Paciorek & McLachlan, 2009).
After ca. 100 years of palynological and archaeobotanical studies in Europe and North America, their vegetation history, particularly for certain regions, is well known (see Brewer et al., 2017; Latałowa & van der Knaap, 2006; Ralska-Jasiewiczowa et al., 2004). However, there are a number of severely underinvestigated regions in the Northern Hemisphere, and particularly in the Southern Hemisphere.
Reconstruction of past vegetation distribution derived from pollen records provides an important baseline for understanding long-term ecosystem dynamics and modeling future environmental changes (Bunting et al., 2013). It is also possible to examine important features of the vegetation, such as composition, richness, life form spectrum, and even diversity (Birks et al., 2016; Matthias et al., 2015; Papadopoulou et al., 2022).
Palynological studies and predictions of vegetation history and its dynamics are still developing and involve modern ecological concepts. They help to interpret past pollen assemblage richness, estimate taxa richness from plant macrofossils, partition diversity, and estimate beta diversity, and apply the concepts of the species pool, pollen pool, hidden diversity, dark diversity, and dark richness, as well as functional and phylogenetic diversity (Birks et al., 2016).
The perspective of research using palynological and archaeobotanical methods in regions outside Europe and North America is still intriguing. New techniques of radiometric dating, new sites and profiles, and molecular methods for studying sedimentation profiles open up wide fields of research and may enable important discoveries in the field of mapping past vegetation.
Standard geobotanical sampling can be used to predict future vegetation change scenarios. The modeling of future ranges of plant communities can be based on species distribution, plant traits or lists (including standard relevés), or vegetation types. Modeling a niche on the basis of empirical data with the use of climate databases such as WorldClim or CHELSA allows for a relatively precise prediction of changes in vegetation type ranges depending on key climatic or environmental variables (Yang et al., 2019).
In recent years, due to climate warming in most areas of the world, there has been increased interest in researching treeline dynamics and shifting ranges towards the poles. Basic geological data make a valuable contribution to in-depth analyses of global changes in vegetation and species richness, such as accelerated growth in the number of species on mountain peaks (Steinbauer et al., 2018). High-resolution information on climatic conditions (e.g., WorldClim, CHELSA, CRU, GPCC, and PRISM) is currently available for research and can be used in species distribution models (SDMs) (Karger et al., 2017). Environmental niche modeling is widely used to predict the spatial distribution and shifts of species and vegetation types due to environmental changes (e.g., Elith & Leathwick, 2009). Extrapolations of potential invasions and expansions of alien species are particularly effective, especially if the new range is flat terrain. The precise modeling of range changes in areas with very high altitude amplitudes and relief complexity remains a challenge. These vertical variations cause microclimatic differences and thus influence the distribution of species (Bramer et al., 2018). Nevertheless, we obtain a number of accurate predictions of invasive species, hybrids, or plant communities present in recent years, which considerably helps in effective conservation and management (e.g., Jaźwa et al., 2018; Konowalik et al., 2017; Szumańska et al., 2021).
However, there is still an urgent need to develop this discipline, including new areas and testing new species for potential invasiveness, niche divergence, or compositional changes in vegetation, as the number of alien species continues to grow and the mixing of flora is constantly progressing (van Kleunen et al., 2019). It is also important to integrate all databases that include taxonomic, functional, ecological, and distribution data of nonnative species (Ruiz-Benito et al., 2020).
. Geobotany With Functions and Traits
Trait-based approaches are a rapidly developing section of plant ecology and vegetation science, as functional diversity is regarded as a major component of biodiversity, and plant functional traits are sensitive to environmental conditions and directly linked to growth, reproduction, and spread (Dzwonko & Loster, 2011; Schneider et al., 2017). There is a growing number of studies on the distribution of particular functional traits, such as leaf dry matter content (Ali et al., 2019), specific leaf area, nitrogen and phosphorus mass (Butler et al., 2017), plant strategy types (Schmidtlein et al., 2012), pollination types (Feilhauer et al., 2016), and many others. Additionally, many studies on the analysis of functional traits (e.g., vertical distance between the canopy top and the ground, projected plant area per horizontal ground area, and leaf chlorophyll) with the use of remote sensing have been successfully completed and depict the ecosystem structure, canopy architecture, specific leaf area, and photosynthetic performance (e.g., Schneider et al., 2017; Zheng et al., 2021).
This emergence of functional biogeography (Kattge et al., 2020) is strongly related to classical geobotany and its main questions raised by Humboldt. The application of new methods, such as remote sensing or global databases, should enhance the mapping of functional diversity instead of the fairly well-known taxonomic diversity. Despite the important results of the above-mentioned studies, the mapping of the distribution of plant and vegetation traits and their partitioning into diversity components still remains very promising in terms of research and is extremely important from the point of view of conservation and ecosystem management.
. Geobotany – The Metabarcoding Challenge of Fungi (Geo-Mycology?)
Similar to the below-ground parts of plant communities, fungal communities can be analyzed on the basis of DNA barcodes. More than 500 recently published papers have used ITS barcoding (Xu, 2016). Mushrooms are found in a wide variety of habitats; however, their global distribution and diversity remain poorly understood (Martorelli et al., 2020). The first studies, based on ITS2 rDNA sequencing, gave promising results in unraveling the distribution of red-listed fungi in different soil profiles in the Netherlands (Geml et al., 2014). In Poland, 36 species (47 of all the listed fungal taxa) were identified by ITS DNA barcoding during interesting research on wild edible mushrooms collected and stored in the countryside of the Masovia region (Kotowski et al., 2019). Recently, the Myco Diversity Database was established for comparative analyses of the barcodes of fungi from environmental samples to enhance the worldwide study of the biodiversity patterns of fungi (Martorelli et al., 2020).
Despite these obvious achievements, fungal metabarcoding still has many unresolved issues and is quite a challenge. It requires the identification of new barcodes to distinguish sister taxa as well as the development of a common set of primers. It would also be important to develop a reference database for known fungal taxa (approximately 100,000 out of 2–10 million). To analyze the distribution of fungi at the species level, it is indispensable to establish unambiguous rules for the recognition of species based on DNA barcode readings (Xu, 2016). A wide range of analyses of distributional patterns of fungi based on environmental and specimen samples would contribute significantly to the global knowledge of fungal geography.
. Geobotany – A Global Summons
As geobotanists, we have inherited a deep need for peregrination from Alexander von Humboldt; we carry out research expeditions, regardless of the scale. These can be trips to a neighboring forest, to a clearing, to the nearest mountains, to an adjoining country, to a neighboring continent, and finally around the world. A global perspective is needed to explain the global patterns of plant and vegetation diversity. sPlot 3.0, which reflects this need, has the largest repository for plant communities’ data in the world and contains almost 2 million records with full lists of plant species co-occurring in standard plots (Bruelheide et al., 2019). Almost all samples were geo-referenced and contained data from 161 databases, 140 countries, and seven continents. This unusual tool makes a great difference in exploring global vegetation and provides exceptional prospects for examining the taxonomic, functional, and phylogenetic diversity of plant communities. It also serves as a good application for understanding the role of temporal and spatial scaling in plant diversity. Within the last few years, the sPlot database has enabled us to unravel the global patterns of alpine plant species richness and the functional variation of alpine vegetation (Testolin, Attorre, et al., 2021; Testolin, Carmona, et al., 2021) as well as global fern and lycophyte richness (Weigand et al., 2020), and also helped explain the abundance and distribution patterns of European plants at different spatial scales from a functional perspective (Sporbert et al., 2021). Additionally, the GrassPlot database has been established as a tool for exploring multi-scale patterns of grassland diversity (Dengler et al., 2018). The GrassPlot database includes high-quality phytodiversity data sampled on plots of the following standard areas: 0.0001 m2, 0.001 or 0.0009 m2, 0.01 m2, 0.1 or 0.09 m2, 1 m2, 10 or 9 m2, 100 m2, and 1,000 or 900 or 1,024 m2 (Biurrun et al., 2019). There are currently about 50 research projects that use data from only these two databases. This shows the scale of the demand and research potential of databases containing high-quality vegetation data from around the world. New databases for vegetation also emerge at the regional scale, with the number of relevés still growing (Kącki & Śliwiński, 2012), while some research focuses on country-scale studies of plant communities. A global perspective is increasingly present in many studies dealing with the classification, distribution patterns, and visualization of vegetation types (e.g., Fleri et al., 2021; Hunter et al., 2021).
. Geobotany – Recreational and Psychological Issues (Ecosystem Services in Spatial and Functional Scale)
Vegetation has a significant influence on the quality of human life. Particularly in cultural or anthropogenic landscapes, the remnants of natural vegetation have restorative or stress-reducing effects on psychological functioning (Hernández & Hidalgo, 2005). This may suggest intrinsic biophilia or provide a clue to the reconstruction of the primeval vegetation landscape of early Homo sapiens. There is still little research on how humans react, rest, and feel in different types of plant communities. It has recently been shown, on a fairly rough sample, that we prefer medium-dense stands and like to stay inside them (the highest stress reduction), although we are the most concentrated (attentional functioning) when we are at the forest border in the ecotone zone.
Vegetation science and geobotany have a deep understanding of the diversity and quality of ecosystems and thus can provide an integrative perspective as well as analytical tools for studying human health in relation to the quality of the environment. With knowledge of the distribution, structure, and composition of vegetation types, features of vegetation that affect people’s well-being, their diseases, levels of stress, or their feelings of rest and happiness can be easily determined (Chiang et al., 2017). The concept of aesthetic ecology and a socioecological approach in human sciences have led to a deeper understanding of the role of vegetation in human well-being (Gobster et al., 2007). Vegetation, traditional species composition, and the share of fragrant, edible, or medicinal species, as well as species with other kinds of usage, combined with the simultaneous absence of toxic, venomous, and virulent species, have made certain phytocoenoses attractive for thousands of years. Since the beginning of humankind, we have preferred bright, species-rich, lush groves that shelter, protect, and feed people. Additionally, they enhance emotional and mental health, reduce anxiety and stress, increase attention deficit recovery, decrease depression, enhance memory retention, improve life satisfaction, support the feeling of happiness, mitigate post-traumatic stress disorder (PTSD), increase creativity, enhance productivity, reduce the effects of dementia, and improve self-esteem. This translates into the well-being of the inhabitants of such ecosystems. With the growing interest in therapy in botanical gardens (e.g., aromatherapy) and the constantly increasing rate of mental illness in the human population, is certainly an interesting research perspective. Vegetation therapy is possibly waiting for its discovery and wide application (see Capra et al., 2019; Górska-Kłęk et al., 2009 for plant and horticultural therapy).
. Geobotany – The Citizen and Social Science Perspective
Geobotany is a privileged discipline with an important relationship to and potential engagement with society. Vegetation was the first home of ancient Man and remains an important resource of natural goods, a space for recreation and relaxation, and an interesting space for research and education to the present day. In almost every civilized country, there is a long tradition of geobotanical research and a number of scientific or educational associations that aim to establish a tight relationship between vegetation science and society. An example is the Polish Botanical Society, with its 100-year tradition of geobotany and nature conservation. It is no surprise that flora and vegetation are the subject of much research on citizen science.
An example here is the analysis of heterostyly in European primrose populations (Primula veris, P. officinalis). This project, led by scientists from the University of Tartu, received a wide response in European societies and was instrumental in improving the understanding of the environmental causes of proportions and prepolyps of prepollination, which already troubled Darwin (Aavik et al., 2020). In healthy cowslip populations, there should be equal numbers of both morphs of flowers to enable successful breeding. Their imbalance indicates population decline, loss of habitat, or a change in management. In 2019, the research team obtained data on the morph type of >240,696 cowslip individuals from >2,641 locations across Estonia (“Looking for Cowslips,” 2021).
Recently, vegetation has also become a subject of increasing public interest. This is related to the recreational value of plant communities (e.g., forests, heaths, and meadows) or the relationship between the occurrence of certain harvested mushrooms and the type of tree stand. A new online platform was created to identify plant communities in Central Europe (Tichý & Chytrý, 2019). It allows for the quick identification of vegetation types in the field, based on species composition. The method is based on calculating the probability that a few species observed in the field would co-occur in a priori defined vegetation types, using the existing information on the species occurrence frequency in these types of vegetation. The Android software Probabilistic Vegetation Key can be easily used on smartphones, including offline.
Other citizen science projects focus on phenology and seasonal changes in flowering. It helps substantially to understand global changes in plant and vegetation phenology and correlate them with data obtained by remote sensing (e.g., Sentinel-2 survey; https://ritmenatura.cat/projects/phenotandem/index-eng.htm). The importance of citizen science will inevitably increase, and projects in relation to plants and vegetation will continue to grow. One can imagine many ideas involving amateur botanists in vegetation studies, for example, using private drones to map the vegetation in their place of residence. Currently, projects related to the distribution of edible mushrooms, segetal species, and invasive species are being carried out in Central Europe (https://www.univie.ac.at/oemykges/pilzfinder-at/ and https://easin.jrc.ec.europa.eu/easin/) (Tsiamis et al., 2017). It is certainly worth considering the potential topics of cooperation with society.
. Summary
The dreams of Humboldt and many of his successors have not yet come true. Despite the great achievements of the last century, we still do not know the total number of vegetation types or the differences in the patterns of the distribution of diversity above and below the ground, and we cannot predict all the effects of climate change on vegetation at the regional and global scales. Additionally, we do not know the hidden and dark parts of species diversity in most ecosystems, nor are we able to make historical vegetation maps for many areas of the Earth. We also do not know the cultural contribution of many plant communities to the development of human populations and civilization, nor do we know the future recreational and therapeutic potential of vegetation (e.g., aromatherapy, ecotherapy). Geobotanical methods can effectively contribute to finding answers to burning questions in current ecology, for example, among many others: (i) What will the consequences of global homogenization, extinction, and climate change be at the community level? (ii) How important are dynamic extinction–recolonization equilibria to the persistence of species assemblages in fragmented landscapes? (iii) Which mechanisms allow the long-term coexistence of grass and woody plants over a wide range of ecosystems? (iv) What do we predict the responses of ecosystems to environmental changes based on the traits of species? (v) How do natural communities respond to the increased frequency of extreme weather events predicted under global climate change scenarios? (vi) What determines whether species adapt, shift their ranges, or go extinct? (vii) What is the impact of the re-emergence of ancient species as a result of permafrost melting in Siberia?
Since there are so many gaps in our geobotanical knowledge, many young researchers are still speechless with delight when looking at endless steppes, lush rainforests, tall-herbs, or colorful meadows, there is no doubt that geobotany will be a thriving and developing discipline in the future. Its driving force is the passion of researchers and admiration for the beauty of various types of vegetation, their dynamics, and the awareness of the need to protect them for generations to come.