. Introduction
In the past century, the human population, global economy, and scale of trade have increased dramatically. Consequently, a concomitant boost in the demand for resources and energy has had a profound impact on the natural world (Edesi et al., 2020). Human activities have led to an unparallel increase in the rate of biodiversity loss. In the plant kingdom, this has resulted in a ca. 50% reduction in plant biomass relative to pre-human levels, and approximately 40% of plant species are currently threatened with extinction (Breman et al., 2021). Moreover, the number of crop varieties cultivated worldwide has decreased by 75% in the past century owing to advances being made in the breeding and introduction of superior cultivars, replacing local forms and landraces (Sundar, 2011). The conservation of plant diversity is urgently required to help conservationists stop these negative trends and to ensure that adequate plant genetic resources are available for both current and future generations.
In situ conservation is the primary approach for species protection; however, because their natural environment plants are continuously exposed to climate-, pollution-, and pathogen-related threats, safety backups are required (Kulus, 2019). Among the ex situ conservation strategies, cryopreservation, i.e., the process of cooling and storing plant cells, tissues, or organs in liquid nitrogen (LN) (−196 °C) or LN vapor (−160 °C), may be the most effective form of long-term biodiversity protection (Benelli, 2021). Because of its minimum space requirements and maintenance efforts, this technology has become a very important tool for conservationists. It is the sole ex situ conservation method for the long-term preservation of species that cannot be deposited in seed banks, such as clonal crops (fruit, ornamental, and vegetable plants that require clonal reproduction owing to their high heterozygosity) (Podwyszyńska et al., 2022), or species with a low seed number or recalcitrant seeds (Pence, 2014). Since the 1990s, the successful cryopreservation of plant cells and tissues has gradually increased (Panis, 2019). Although no biological sample is immortal, specimens stored in LN can have indefinite lifespans (D. Z. Li & Pritchard, 2009). Nonetheless, the global use of cryopreservation in gene banks remains limited compared with traditional low-temperature seed storage (Bettoni et al., 2021).
Currently, in Poland, there are four cryobanks implementing long-term protection programs with international cooperation (Zimnoch-Guzowska et al., 2022). Among these four units, two have specialized mainly in the long-term preservation of seeds of native and endangered species of the Polish flora. Other plant materials in LN storage include cell suspensions of members of the Gentiana genus, embryogenic lines, dormant buds and plumules of forest trees, shoot tips of potato (Solanum spp.), and gametophytes of several fern species (Table 1).
Table 1
In vitro culture-derived plant material stored in Polish cryobanks.
The Polish Academy of Sciences Botanical Garden – Center for Biological Diversity Conservation in Powsin (PAS BG-CBDC) mainly focuses on collecting and storing seeds of the most threatened and endangered plant species in Poland. There is also the world’s largest cryo-collection of tree ferns. The Kostrzyca Forest Gene Bank (Kostrzyca FGB) maintains seed collections of numerous tree and herbaceous species, representing various botanical families. A European integrated Allium Core collection was founded in 2011 as part of the European Tripartite Garlic Cryobank at the Research Institute of Horticulture – National Research Institute in Skierniewice (InHort). Finally, the collection of cryopreserved potato accessions was established at the Młochów Research Center of the Plant Breeding and Acclimatization Institute – National Research Institute (PBAI-Młochów RC). Cryobanks use various common cryopreservation procedures, such as direct freezing, slow-cooling, and vitrification-based “modern” methods (reviewed by M. R. Wang et al., 2021). To correctly use cryogenic techniques, research is focusing on optimizing cryopreservation methods in several other Polish scientific and academic units. Particularly interesting issues covered by Polish research groups are studies on the cryopreservation of geophytes, radiomutants, chimeras, and other ornamental and medicinal plants. Cryopreservation may be even more interesting if one considers the use of non-conventional supplements, such as nanomaterials or plant extracts, in cryobiology studies.
The objective of this review is to provide an overview of the scientific contributions, current status, and applications of cryogenic techniques for the conservation of in vitro culture-derived plant tissues in Poland. This article summarizes research contributing to the basic science that has been achieved using cell suspensions, and advances related to the use of nanoparticles (NPs) and plant extracts to improve cryopreservation efficiency are discussed. Then, the applications and advances in the cryopreservation of ornamental plants and crop plant species, forest trees, and ferns are presented.
. Contribution of Polish Scientists to the Advancement of Cryopreservation Knowledge
Suspension Cultures: Preculture, Recovery, and Rejuvenation
Embryogenic suspensions allow for the handling of large populations of relatively homogeneous cells under controlled conditions of in vitro culture and offer a simplified model system for the study of cellular and molecular processes. They are useful for the large-scale production of morphologically and genetically stable plants, as well as for transformation purposes. The main difficulty in maintaining embryogenic cell suspensions is their ability to regenerate somatic embryos, which disappear over time (Breton et al., 2005; Mikuła et al., 2015). Moreover, prolonged subculturing has a high risk of contamination and is elaborate and expensive. Over 15 years ago (Mikuła, 2006), a scientific team working at the PAS BG-CBDC established a high-throughput protocol via encapsulation/dehydration for the cryopreservation of proembryogenic masses (PEMs) derived from suspension cultures of various species belonging to Gentiana (Figure 1). To improve the survival rates of cell aggregates, special attention has been paid to preculture, cryoprotection, and post-thaw treatment. Many efforts have been made to develop a rapid and reproducible method with which to assess the viability of PEMs after thawing. These studies have increased our knowledge of the possibility of routinely using cryogenic techniques for the long-term preservation of suspension cultures, as well as the benefits and risks of cryogenic treatments, as described below.
Figure 1
Cryopreservation and restoration of suspension cultures of Gentiana spp. by the encapsulation/dehydration method. (A) Embryogenic suspension cultures of G. kurroo Royle in proliferating (PS) and somatic embryo-producing (ES) phases (for more details see Mikuła et al., 2022). (B) Freshly prepared control capsule with proembryogenic masses (PEMs) of G. cruciata L. (C) Control capsule (on the top) and proliferating tissue after 14 days of post-thaw culture on agar medium (on the bottom). (D) Proliferation and release of cell aggregates of G. tibetica King. into liquid nitrogen (LN) (Day 21 of culture). (E) Numerous somatic embryos of G. cruciata developed from suspension culture recovered after storage in LN; 4 months on agar medium. (F) Plantlets of G. kurroo regenerated after cryopreservation (Mikuła, Tomiczak, et al., 2011).
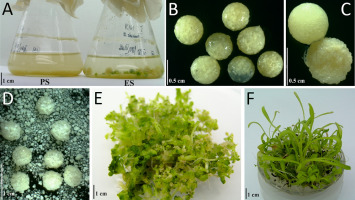
Effect of Preculture
During cryopreservation, controlled water removal plays an important role in preventing the formation of ice crystals in cells, helping to reduce and/or eliminate freeze-thaw injury, as well as maintaining high viability after cryostorage. To induce desiccation tolerance under in vitro culture conditions, a pre-culture treatment is used. In this step, cells are treated for several days (Lambardi et al., 2008) or weeks (Hitmi et al., 1999) with a medium containing one or more osmotic agents (saccharides or saccharide derivatives), amino acids (proline), or abscisic acid (ABA). In the protocol described for Gentiana spp., sucrose at an optimal concentration of 6% was used for 4 weeks. The agent was found to work well in liquid medium for a wide range of encapsulated suspension culture-derived embryogenic cell aggregates of Gentiana spp., including G. cruciata, G. tibetica (Mikuła et al., 2008), G. kurroo (Mikuła, Tomiczak, et al., 2011), and more recently, G. capitata and G. decumbens (Tomiczak & Markowski, 2021). Such treatment determines the progressive acquisition of specific endogenous substances, such as proteins (dehydrins), sugars (sucrose, glucose, fructose), proline, or hormones (ABA) (Hitmi et al., 1999), and leads to the structural reorganization of the cells (Mikuła et al., 2005). This is reflected in the strong concentration of cytosol, the appearance of numerous small vacuoles in place of large ones, an increase in starch content, and the fragmentation of the endoplasmic reticulum in the cytoplasm of G. tibetica cell aggregates that were precultured (Mikuła et al., 2005). These changes were similar to those found both under pre-culture conditions in vitro (Bachiri et al., 2000) and in vivo during plant entry into dormancy (Pomeroy & Andrews, 1978). The study showed that as a result of a 4-week-long preculture with 6% sucrose, the water content in the cells of G. tibetica PEMs was 10% lower, whereas the level of bound water was higher, than those at the start of preculture (Mikuła, 2006). The effect of preculture preceding further cryotreatment is a multiple increase in regenerative capacity after storage in LN, which has been observed in Gentiana spp. (Mikuła, Tomiczak, & Rybczyński, 2011; Mikuła, Tomiczak, et al., 2011) and Vitis vinifera (Q. Wang et al., 2002).
Ultrastructural changes occurring in plant cells are a response to osmotic stress and the beginning of further biochemical and physiological alterations. The effects of these changes were largely dependent on the duration of the preculture. Ultimately, by increasing cell survival, prolonged preculture contributes to more efficient recovery of suspension cultures.
Viability and Rapid Efficient Recovery of Cryopreserved Cell Suspension Cultures
In the cryopreservation of cell suspensions, it is important to have a tool that allows for the early, rapid, and reliable prediction of the post-thawing viability of cells. Comparing the effectiveness of different cryopreservation techniques is also desirable, especially because they require the use of different recovery culture conditions and affect suspension culture restoration (Mikuła, 2006; Mikuła et al., 2008). Although regrowth measurement of cells is the most sensitive test for viability, the method is time-consuming because it can take several weeks. A rapid (1–2 days) method was developed to replace regrowth-based assessments is the tetrazolium (2,3,5-triphenyl tetrazolium chloride) (TTC) test (Mikuła et al., 2006). This test was not widely used to determine PEM viability until attempts were made to standardize it, as cell survival tended to be overestimated. Formazan levels can increase many times in osmotically stressed cells (Duncan & Widholm, 2004), and dehydrogenase activity can persist for some time despite the inability of cells to continue life functions (Pelah et al., 2003; Smith et al., 1982). Studies using cell suspensions of Gentiana spp. have revealed that dehydrogenase activity in lethally damaged cells decreases gradually within approximately 1 hr, and formazan production sometimes persists for up to 48 hr (Mikuła et al., 2006). This may be due to the presence of living, weakened, and dead cells in the thawed explant, and the result depends on the ability of the cells to tolerate and repair the stress-induced damage (Verleysen et al., 2004). Based on our knowledge, the TTC test can be described as reproducible and reliable if it is performed within the first 48 hr after thawing the plant material (Mikuła et al., 2006). It is now routinely used to estimate the cryopreservation efficiency of cell suspensions from various plant species (Nausch & Buyel, 2021), including gentians.
Retaining the high growth activity of embryogenic suspension cultures is a critical factor affecting the success of their recovery after cryopreservation. The rapid recovery of cryopreserved suspension cultures depends on the morphogenetic status of the tissue and pre- and post-thaw manipulations. Embryogenic cells are supported by a higher respiratory capacity than are non-embryogenic cells. They respond more efficiently to oxidative stress and changes in glycolytic and respiratory pathways (Benson et al., 1992). This has important implications for the survival of cells and their ability to perform vital activities after cryopreservation (Bachiri et al., 2000; Škrlep et al., 2008). However, the rapid and reproducible recovery of suspension cultures from thawed cell aggregates has been associated with tissue treatment after thawing. Detailed information on this topic is provided in studies on G. tibetica (Mikuła, 2006), G. cruciata (Mikuła et al., 2008), and G. kurroo (Mikuła, Tomiczak, et al., 2011). Tissue frozen by slow cooling or by the vitrification technique is most often cultured on agar medium for approximately 3 weeks (Mikuła, 2006; Sadia et al., 2003; Škrlep et al., 2008), and less often for a shorter time (e.g., 7 days) (Menges & Murray, 2004). After this step, tissue proliferation can be used to recover liquid culture. Using Gentiana spp., this two-step pathway was demonstrated to favor the emergence of populations of elongated, highly vacuolated, and non-embryogenic cells (Mikuła, 2006; Mikuła et al., 2005). The study showed that it takes approximately 4 months to obtain fully functional gentian suspension cultures that were frozen in LN using the vitrification method (Mikuła, 2006). Only the encapsulation-dehydration method was confirmed to be highly effective in recovering the suspension culture. This allows thawed tissue to be placed directly into the liquid medium (Figure 1). This method of recovering suspension cultures has been successful for embryogenic tissues of G. cruciata (Mikuła et al., 2005) and G. tibetica (Mikuła, 2006), as well as for non-embryogenic suspension cultures of tobacco BY-2 (Kobayashi et al., 2005).
Rejuvenation of Embryogenic Capacity of Suspension Cultures
The cells in suspension cultures may experience partial or total rejuvenation. This process is largely owing to the removal of constraints imposed by neighboring cells and their metabolic products (Aderkas & Bonga, 2000). Several studies have reported that the difficulty associated with the aging of suspension cultures can be overcome by cryopreservation (Table 2). These studies show that the embryogenic capacity of PEMs increases under stressful conditions of preculture treatment, especially when a high concentration of sucrose is used. Treatment of cell aggregates for just 1 hr with 0.6–1 M sucrose solution enhanced somatic embryo production (Aguilar et al., 1993; Engelmann et al., 1997; Lardet et al., 2007). The embryogenic potential of suspension cultures can be increased by a factor of 20 when PEMs are cryopreserved using the encapsulation-dehydration method. The reasons for the increase in embryogenic capacity during cryopreservation should be investigated during pretreatment (Mikuła, Tomiczak, & Rybczyński, 2011; Q. Wang et al., 2002). The high osmotic values of the pretreatment media lead to the dehydration of the cells and positively influences their freezing tolerance. In contrast, severe osmotic stress disrupts intercellular communication and promotes cell isolation (Bachiri et al., 2000). Moreover, studies on the suspension cultures of Gentiana spp. have revealed that prolonged sucrose treatment leads to an increased population of cells with embryogenic characteristics (Mikuła et al., 2005). The stimulation of the embryogenic potential of G. cruciata and G. kurroo occurred after osmotic dehydration of the encapsulated tissue, carried out by gradually increasing sucrose solutions over 7 days. Further steps of the cryopreservation procedure, that is, air dehydration and LN treatment, did not alter the regenerative potential of the suspensions studied. Osmotic dehydration leads to the elimination of potentially non-embryogenic cells from the cultures. This phenomenon is referred to as cryoselectivity and is considered to be a potential cause for increasing the efficiency of somatic embryogenesis in conifers (Häggman et al., 1998), Citrus deliciosa (Aguilar et al., 1993), or gentians (Mikuła et al., 2005).
Table 2
Samples of rejuvenation of embryogenic suspension cultures after cryopreservation in order of increasing embryonic capacity of proembryogenic masses (PEMs).
Species | Plant material | Pretreatment (time) | Cryopreservation technique | Embryogenic capacity | References |
---|---|---|---|---|---|
Hevea brasiliensis Muli. Arg. | Nine NE and 30 E callus lines | 1.0 M sucrose (1 hr) | Controlled-rate cooling | Five NE lines acquired, three E lines lost | Lardet et al. (2007) |
Citrus deliciosa Tan. | Suspension culture | 0.3–0.6 M sucrose (1 hr) | Controlled-rate cooling | enhance | Aguilar et al. (1993) |
Hevea brasiliensis Muli. Arg. | Two callus lines | 1.0 M sucrose (1 hr) | Controlled-rate cooling | 1.2× higher | Engelmann et al. (1997) |
Festuca spp. Lolium spp. | Suspension culture | 0.33 M sorbitol (3–4 days) | Controlled-rate cooling | 2× higher | Z. Y. Wang et al. (1994) |
Cyclamen persicum Mill. | Suspension culture | 0.6 M sucrose (2 days) | Freezing in two steps | 4× higher | Winkelmann et al. (2004) |
Vitis vinifera L. | Suspension culture | 1 M sucrose (3 days) | Encapsulation-dehydration | 7× higher | Q. Wang et al. (2002) |
V. vinifera ‘Tempranillo’ and ‘Riesling’ | Suspension cultures | 0.25 M, 0.5 M, 0.75 M, and 1 M sucrose (for 1 day each) | Encapsulation-dehydration | Enhance | Ben-Amara et al. (2013) |
Gentiana cruciata L. | Suspension culture | 0.3–1 M sucrose (7 days) | Encapsulation-dehydration | 9× higher | Mikuła, Tomiczak, and Rybczyński (2011) |
Gentiana kurroo Royle (Figure 1A) | Suspension culture in E phase | 0.3–1 M sucrose (7 days) | Encapsulation-dehydration | 9× higher | Mikuła, Tomiczak, et al. (2011) |
Suspension culture in P phase | 0.3–1 M sucrose (7 days) | Encapsulation-dehydration | 20× higher | Mikuła, Tomiczak, et al. (2011) |
[i] P phase and E phase – suspension cultures in the proliferation and embryogenic phases, respectively (Mikuła, Tomiczak, et al., 2011); NE – non-embryogenic line; E – embryogenic line.
The enhanced embryogenic potential of 3.5-year-old G. cruciata (Mikuła, Tomiczak, & Rybczyński, 2011) and 6-year-old G. kurroo (Mikuła, Tomiczak, et al., 2011) suspensions, that is, cultures threatened with loss of regenerative capacity because of their age, is direct proof of the possibility of using cryopreservation to rejuvenate cell suspensions and restore their regenerative potential. Regenerants obtained from these cryostored PEMs were also observed to maintain genetic uniformity. Thus, cryopreservation opens up important avenues for its practical use, compared to the long-term conservation of plant tissues, which was first mentioned by Engelmann (2004).
Plant Extracts and Nanoparticles (NPs) in Improving Cryopreservation Methods
The efficiency of cryopreservation can be improved by the application of non-conventional additives, such as natural plant extracts or NPs, during various steps of the cryoprocedure. However, they are not yet widely used, and few research centers worldwide have undertaken studies on their use to improve cryogenic procedures.
Plant extracts are cheap and natural sources of phytohormones, vitamins, nutrients, phenols, and proteins that are beneficial for plants (Gnasekaran et al., 2010). Over the years, extracts of various origins have been added to in vitro propagation media (Molnár et al., 2011; Venkatachalam et al., 2015), but little attention has been paid to their use in cryopreservation. In a study by Kulus and Miler (2021), fresh aquatic extracts obtained from coconut shreds, oat, rice, and sesame seeds were added into the preculture medium during cryopreservation via the encapsulation-vitrification of two bleeding heart cultivars. It was found that coconut extract, abundant in endogenous cytokinins and simple sugars, stimulated more intensive shoot proliferation and development after the rewarming of explants than the extract-free control did. A similar phenomenon was reported when 10% coconut water was added to Murashige and Skoog (MS) medium during the micropropagation of Pogostemon cablin Benth. (Swamy et al., 2014). Conversely, oat and sesame extracts had a deleterious effect on cryopreservation efficiency in the bleeding heart cultivars. The diverse effects of plant supplements most likely results from their different compositions, which affect the physicochemical properties of the extracts and, thus, the uptake of nutrients and cryoprotectants by the explants (Meï et al., 2015).
Nanomaterials can be highly effective tools for safeguarding plant genetic resources. One of the unique properties of metal NPs is their high thermal conductivity (M. Li et al., 2005), which is crucial for the success of cryopreservation at the tissue cooling and rewarming stages. However, very little information is available on the use of NPs in the cryopreservation of shoot tips or meristems (Roque-Borda et al., 2021), which are the most frequently used explants in plant cryopreservation (Kulus & Zalewska, 2014). In a study on Lamprocapnos spectabilis ‘Valentine,’ gold nanoparticles (AuNPs) at various concentrations (10–30 ppm) were added to the preculture medium, alginate bead matrix, or recovery medium during the encapsulation-vitrification protocol (Kulus & Tymoszuk, 2021). The presence of AuNPs at the lowest concentration (10 ppm) increased the viability of explants stored in LN by approximately 20%, without affecting the genetic stability of the recovered plants. In contrast, the presence of NPs in the recovery medium had a deleterious effect on the survival of the shoot tips by stimulating the production of reactive oxygen species and the disruption of cell membranes. Nonetheless, these results confirm the usefulness of NPs in cryopreservation.
In conclusion, the use of non-conventional supplements during various stages of cryopreservation may greatly affect its efficacy. Coconut extract and AuNPs added at low concentrations either to the preculture medium or alginate bead matrix, respectively, seem to be particularly promising, and more attention should be focused on their potential in cryobiology studies.
. Applications and Advances in the Cryopreservation of Plant Tissues
To meet the growing needs of consumers, countless new cultivars of ornamental and crop plants are being produced to replace the current assortment. Floricultural abundance creates problems for breeders in securing diversity as a potential source of traits. Therefore, there is an urgent need to develop efficient methods for the long-term storage of genetic resources for future breeding, especially in view of rapidly changing consumer preferences.
Rosa spp.
Roses are the most popular ornamental plant species in the world. Every year, breeders introduce many new cultivars to the market. However, rose breeding is based on a narrow gene pool, which can lead to genetic erosion. Therefore, there is a tendency for breeding to return to the original gene pool and reach wild species (Leus et al., 2004). The development of efficient cryopreservation protocols may be useful in supporting future rose biotechnology programs, as they offer a stable and safe method for the long-term storage of plant tissues while safeguarding genetic stability. The first reports in the literature on rose cryopreservation discussed pollen preservation (Marchant et al., 1993; Rajasekharan & Ganeshan, 1994). In subsequent studies, the shoot tips derived from in vitro grown plantlets were used for encapsulation (Lynch et al., 1996) or droplet vitrification (Halmagyi & Pinker, 2006a, 2006b). The small number of studies is owing to difficulties in the reproduction and in vitro regeneration of various species and cultivars of Rosa. One of the most important factors determining regeneration in vitro is the properties of the genotype (Kucharska et al., 2006; Pawłowska, 2011). This group of woody plants requires constant research on the composition of the media and external conditions of in vitro cultures. Advanced studies on cryopreservation require protocols for the efficient micropropagation of roses by stimulating lateral buds, which guarantees the highest stability during in vitro propagation.
Since 2008, the University of Agriculture in Krakow has refined methods for preparing plant materials for long-term storage in LN. Studies on the cryopreservation of plant explants originally focused on roses. The survival rate of the initial method of the dehydration-encapsulation of apical buds of Rosa ‘New Dawn’ collected from an in vitro culture was only 20% (Pawłowska & Bach, 2011). When the method was changed to droplet vitrification, the survival rate increased several times. The development of in vitro cultures of four Polish wild rose species, namely Rosa agrestis Savi, R. canina L., R. dumalis Bechst. them. Boulenger, and R. rubiginosa L., were necessary to produce a large number of explants for cryopreservation and offered the possibility of explant regeneration after removal from LN. The survival rate increased to 78%–95%, and the regeneration rate was always above 50%. Rose shoot tips intended for cryopreservation required the maximal exposure of the meristem before applying a plant vitrification solution. The survival rate was also improved by the preculture of donor plants in a medium with 0.25 M sucrose (Pawłowska, 2012; Pawłowska & Szewczyk-Taranek, 2015).
Plant samples collected from the natural environment can also be conserved in LN, with only regeneration conducted in vitro following cryopreservation. A breakthrough discovery for the cryopreservation of roses was the use of shoot tips isolated in winter from dormant buds growing on the ground. In this case, the survival and regeneration rates were close to 100% (Pawłowska & Szewczyk-Taranek, 2014). Several years of research have expanded our knowledge on the cryopreservation of roses and culminated in developing a procedure that may be an excellent method for preserving numerous species and cultivars of this genus that cannot be stored as seeds (achenes) in gene banks. The effectiveness of the procedure was verified for an economically important genotype, Rosa pomifera ‘Karpatia,’ where a survival rate of over 80% has been achieved (Kwaśniewska et al., 2017). In the method developed at the University of Agriculture in Krakow, the cryopreservation efficiency was 30% higher than that of Rosa chinensis. The old blush was grown in a greenhouse and frozen by a French team using drip vitrification and encapsulation-dehydration methods (Le Bras et al., 2014). A great advantage of this method is that it eliminates the costly maintenance of in vitro culture collection and reduces the risk of somaclonal variation during prefreezing culture. After cryopreservation, the explants developed shoots that were multiplied and rooted in vitro, acclimatized, and finally planted in the field. Several years of monitoring of morphometric features, as well as biochemical and physiological analyses, have confirmed the stability of the plants (Pawłowska et al., 2019).
Radiomutants and Plant Chimeras
Chrysanthemum [Chrysanthemum ×morifolium (Ramat.) Hemsl.] is one of the most popular ornamental plants, along with rose and carnation (Miler et al., 2021). New cultivars of this species are produced each year, often as a result of induced mutational breeding. Unfortunately, the application of aggressive mutagenic factors can cause severe changes in the genome, which often leads to the weakening of plant vigor and resistance (Miler & Zalewska, 2014). Recently, studies have aimed to develop encapsulation/dehydration-based cryopreservation protocols for Polish chrysanthemum cultivars created by irradiation with X-rays and gamma rays (Kulus, Serocka, & Mikuła, 2018). The results were very promising, with a survival rate of 90%–100% among the explants (Kulus, 2018), and could be utilized for establishing a cryobank of radiomutants useful for future generations of breeders.
Another issue related to mutation breeding is that many of the cultivars developed using this method are genetically unstable chimeras (Su et al., 2019). Owing to their high instability, chimeras are problematic in propagation and storage, as injuries to the meristem may result in the rearrangement of histogen layers and separation of chimera components. The first report on the cryopreservation of plant chimeras was described by Fukai et al. (1994). Unfortunately, these studies were unsuccessful because the chimeric structure of the apical dome of 70% of chrysanthemum plants was disturbed by the vitrification protocol used. Therefore, further research is required in this field.
Experiments on the influence of cryotreatment on the ultrastructure of cells and their development in vitro were performed by Kulus & Abratowska (2017) using C. pacificum Nakai [syn. Ajania pacifica (Nakai) K. Bremer & Humphries], a novelty in the horticultural market and a close relative of chrysanthemum. Microscopic imaging analyses revealed significant differences in the structure of the shoot tips stored in LN, influencing their further development. According to Halmagyi et al. (2017), injuries that occur at the cellular and subcellular levels in shoot apices cannot be attributed with certainty to a single stress element, but rather act cumulatively following the successive stages of a cryopreservation protocol and ex vitro acclimatization of recovered plants.
To understand the impact of cryoinjury on the morphogenetic response of explants after rewarming, Kulus, Abratowska, and Mikuła (2018) performed detailed histological and ultrastructural analyses of chrysanthemum shoot tips (solid and periclinal chimeras) stored in LN. They found that extensive damage to the apical meristem (especially the tunica layers) and leaf primordia favors the de novo formation of meristemoids in the living fragments of young leaves covering the meristem and the regeneration of deformed adventitious shoots, which in turn may result in the separation of chimera components. In contrast, keeping the meristem fragments viable in the area of primordia axil activates the development of multiple lateral shoots, while apical meristems, in which all histogen layers have been well preserved, form only single shoots after rewarming (Kulus, Abratowska, & Mikuła, 2018). The last two morphogenetic responses did not affect the stability of the periclinal chimeras.
To eliminate the impact of aggressive penetrating cryoprotectants [e.g., mutagenic Dimethyl sulfoxide (DMSO)] on the structure of the apical dome, an encapsulation-dehydration protocol was developed by Kulus et al. (2019) for cryostorage of chimera cultivars. In this study, the precultured biweekly shoot tips were encapsulated, dehydrated in a sucrose gradient, and desiccated (to 40% of the initial explant fresh weight) before immersion in LN. Genetic, cytometric, biochemical, and biometric analyses of the inflorescences confirmed that the traits of the original cultivars were maintained. However, cryopreservation negatively influenced the development of vegetative organs of the three chrysanthemum cultivars and delayed the flowering time of one of them (Kulus et al., 2019). Nonetheless, the results confirmed for the first time the possibility of the safe storage of plant chimeras in LN without separating their components.
Lamprocapnos
Lamprocapnos spectabilis (L.) Fukuhara (bleeding heart syn. Dicentra spectabilis) is a member of a monotypic genus, valued as a cut flower and pot plant, and has potential use in medicine and cosmetology (S. Park et al., 2018). Because the species can be a good source of health-promoting phytochemicals, it is imperative to develop cryopreservation procedures that are applicable to the most productive cell lines.
Comparative analyses indicated that plant vitrification solution 3 (PVS3) (Nishizawa et al., 1993) was the most effective in the cryopreservation of L. spectabilis, both in terms of explant survival and the quality of the recovered plants (Kulus, 2020a). It was also reported that encapsulated shoot tips had a higher tolerance to prolonged dehydration than the “naked” explants, regardless of LN storage (Kulus, 2020b). Supplementing the alginate bead matrix with salts and vitamins from the MS medium and the effect of this factor on plant development were evident (Kulus, 2020a). Encapsulated explants produced longer shoots with larger leaves and higher fresh weights than untreated controls did (Kulus, 2020c). Among the four tested cryopreservation techniques (e.g., vitrification, droplet vitrification, encapsulation-vitrification, and encapsulation-dehydration), the encapsulation-vitrification approach was the most effective. This technique not only provided the highest recovery level of the explants (73.1%), but also the most dynamic development of plants, that is, shoot proliferation and rooting, which were not observed in the non-treated control (Kulus, 2020b). However, the vitrification technique was the least effective in securing the viability of shoot tips stored in LN (6.4%–25.8% survival, depending on the dehydration duration). Interestingly, opposite results were found for C. morifolium ‘Escort’ (Halmagyi et al., 2004). In this study, vitrification yielded the highest shoot recovery rates, and the droplet method was also successful in this respect. This highlights the need to test various protocols before their routine application for germplasm safeguarding.
Maintaining the stability of LN-stored plant materials is essential for every cryopreservation procedure. Molecular analyses based on several single primer amplification reaction (SPAR) marker systems demonstrated that the encapsulation-based cryopreservation techniques are the most effective in securing the integrity of the genetic material (100% homology of DNA sequence with the non-treated control). In contrast, minor genetic variations (in 5% of the analyzed samples) were detected by the random amplified polymorphic DNA (RAPD) and inter-simple sequence repeat (ISSR) markers after using the other cryotechniques, as well as in the untreated control (Kulus, 2020b, 2020c). Similar low-level variations were detected in Hladnikia pastinacifolia Rchb. (Ciringer et al., 2018), chrysanthemum (Kulus et al., 2019), and other species (Kulus & Mikuła, 2016). Thus, this phenomenon is rare. The detected polymorphisms in the non-treated in vitro-grown control indicate that the long-term storage of L. spectabilis germplasm should be set under cryogenic conditions, particularly by using the encapsulation-vitrification procedure based on the 150-min PVS3 treatment of shoot tips precultured previously for 1 week on MS medium with 9% sucrose and 10 µM ABA, as this protocol ensures the stability of the biological samples.
Geophytes
Studies on the cryopreservation of geophytes carried out at the University of Agriculture in Krakow have focused on endangered species (Tulipa and Galanthus) and ornamental cultivars (Narcissus ‘Carlton’), which are widely used in urban green areas and home gardens. This is the first study in the world on the cryopreservation of these species (Maślanka et al., 2013, 2016; Maślanka & Szewczyk, 2021). This may open new perspectives for creating gene banks of ornamental bulbous plants, which are mainly propagated vegetatively and thus cannot be stored in seed banks. The explants stored in LN were harvested from an in vitro culture and cryopreserved using droplet vitrification.
The cryopreservation of Galanthus elwesii Hook. f. and Tulipa tarda Stapf. involved in vitro isolation of bulb apical meristems after droplet vitrification and resulting in survival rates of 97% and 100% and regeneration rates of 76% and 100%, respectively. This high cryopreservation efficiency was obtained following the preculture of donor plants on a medium containing 6% sucrose. The optimization of the duration of exposure to PVS2 (at least 30 min) was also crucial. The excellent outcome in T. tarda was facilitated by a 10-week period of cooling (5 °C) of the plant material prior to its cryopreservation (Maślanka et al., 2013; Maślanka & Szewczyk, 2021).
The cryopreservation of Narcissus ‘Carlton’ required the collection of somatic embryos at the globular stage. Although they showed a 100% survival rate after cryopreservation by droplet vitrification, the individual stages of the procedure still require optimization. Successful cryopreservation was determined by the size of the embryos, which was 2 mm in diameter. After thawing, single globular embryos may yield a few direct secondary somatic embryos, which is desirable for reducing the possibility of genetic variation (Maślanka et al., 2016).
Crop Species
Solanum tuberosum L.
Solanum tuberosum L. is a fundamental crop and is important for food security worldwide. In Poland, potatoes belong to the group of strategic crops cultivated in 2020 on over 320 thousand hectares, with a total yield of 8.87 million tones. Farmers were supported with seed potatoes of 108 varieties registered in Poland (Lenartowicz, 2021), and several hundred of those registered in the EU catalog. Potatoes, as vegetatively propagated crops, are at risk of degeneration owing to viral, bacterial, and fungal diseases. Advances in the development of new varieties is based on achievements in breeding research and the availability of breeders with new resources for resistance and quality traits. Potato germplasm is stored in the field and in vitro; however, these traditional conservation methods are time- and labor-intensive, and the risk of losing valuable germplasm is relatively high (Kaczmarczyk et al., 2008).
The conservation of shoot tips in LN has been recognized as an important method for the long-term storage of potato germplasm (see reviews by Kaczmarczyk et al., 2011; Keller et al., 2008). Studies on the cryopreservation of potato shoot tips started in the late 1970s, and since then, different methods have been developed using several cooling approaches, including two-step cooling (Bajaj, 1977), encapsulation-dehydration (Bouafia et al., 1996), PVS2 vitrification (Sakai et al., 1990), droplet vitrification (Kim et al., 2006), and the DMSO droplet method (Schäfer-Menuhr et al., 1996). In 2014, approximately 2,800 accessions were cryopreserved from over 10,000 accessions in vitro stored in five potato cryobanks.
In a study spanning from 2002 to 2005, the cryopreservation of shoot tips, pollen, and potato true-seeds was studied for the first time in Poland to develop an efficient method for the long-term storage of valuable potato germplasm (Kryszczuk, 2005). In this study, a selected group of 18 genotypes was represented by seven 4x varieties, nine 2x Solanum interspecific hybrids, and two 4x breeding lines from the in vitro collection. Three methods of potato shoot tip cryopreservation were compared, where the percentage of meristem regeneration after thawing was the main evaluation criterion. The PVS2 vitrification of shoot tips (Sakai et al., 1990) was the most effective method compared to both the encapsulation-dehydration method (Bouafia et al., 1996) and DMSO droplet method (Schäfer-Menuhr et al., 1996). The average regeneration of vitrified meristems after storage in LN was 63.7%, ranging from 57.5% to 82.5%, which was significantly higher than that of the encapsulation-dehydration method (22.5%) and DMSO droplet method (28.8%). By optimizing the vitrification of shoot tips procedures, the percentage of regeneration of smaller meristems (1 mm in length) was significantly higher (63.7%) than that of meristems 3 mm in length (30.4%), which was consistent with the reports of Escobar et al. (2000) for cassava and Takagi et al. (1997) for taro, and was contrary to the study of Schäfer-Menuhr et al. (1996) for potato tips cryopreserved using the droplet method, where the larger meristems had a higher regeneration rate. Kryszczuk (2005) also demonstrated that the incubation time in a cryoprotectant (PVS2) influenced the regeneration efficiency and was dependent on the size of the explants. However, it was not influenced by the potato genotype. For the tips of 1 mm in length, the optimal time for PVS2 treatment was 30 min, which was 10 min shorter than that for shoot tips of 3 mm in length. The cold treatment of donor plant cultures had no significant influence on the efficiency of regeneration of potato genotypes cryopreserved using the PVS2 vitrification method. However, when the DMSO droplet method was applied, the regeneration rate was significantly enhanced by cold treatment of the donor plants, which is in agreement with Kryszczuk et al. (2006) and Kaczmarczyk et al. (2008).
Kryszczuk (2005) found significant differences in meristem regeneration efficiency among 18 potato genotypes, which were not associated with their ploidy level. A high regeneration efficiency (80.6%) was noted for the group of four genotypes represented by three 4x varieties and a 2x hybrid clone DG92-227. A medium efficiency (61.0%) was noted for the group of 10 genotypes, including 4x and 2x breeding lines and two varieties, whereas a lower regeneration efficiency (33.8%) was noted for the group of four genotypes, including two varieties and two 2x hybrids. These results confirm the finding of Kaczmarczyk et al. (2011) that the critical point of potato cryopreservation is the diverse response between different genotypes in terms of their regeneration capacities after thawing. The ongoing procedures for the cryopreservation of potato tissue and organs were slightly modified by Smyda-Dajmund (2017) to increase the regeneration efficiency of the 2x hybrids.
The genetic stability of two 4x and two 2x cryopreserved genotypes was confirmed by Kryszczuk (2005) with four RAPD and ISSR markers each, selected on the basis of previous studies (Flis et al., 2005; Marczewski et al., 2004) to cover all 12 potato chromosomes. For the four studied genotypes, no difference was observed among the electrophoretic data in comparison with the control of in vitro plants. The genetic stability of cryopreserved plant material was also confirmed by Schäfer-Menuhr et al. (1997) using restriction fragment length polymorphism (RFLP) markers, Hirai and Sakai (1999) using RAPD analyses with 17 primers, and Zarghami et al. (2008) using amplified fragment length polymorphism (AFLP) analyses.
Allium sativum L.
Allium sativum L. is highly appreciated in both the medical field and spice markets. It is an annual plant species reproduced vegetatively by daughter bulbs (“cloves”) and bulbils from the flower head. Therefore, there is a risk that some genotypes will be lost owing to biotic and abiotic factors (Keller & Senula, 2013). As garlic does not produce fertile seeds, the maintenance of field collection is laborious and expensive. In vitro-based techniques are effective methods for supporting the preservation of the garlic gene pool (Mahajan, 2016). Long-term cryostorage is well developed to maintain the species germplasm. Cryopreservation procedures for in vitro-derived shoot tips of A. sativum were previously developed with a success rate of 35%–82% (Baek et al., 2003; Keller, 2005; Volk & Walters, 2006). In Poland, one of the largest field collections of garlic in the world has been at InHort since 1986 (Olas-Sochacka & Kotlińska, 2015). The research team of InHort compared, for the first time, the efficacy of bolting and non-bolting accessions, suggesting that the latter is slightly more suitable for LN storage. The main method used in the Polish cryobank is the vitrification of shoot tips (approx. 1.5 mm in length) based on cryoprotectant mixture PVS3 treatment for 120 min. Thus, it is possible to obtain a high regeneration level of explants on MS medium with 1-naphthaleneacetic acid and N6-(2-isopentenyl)adenine, ranging from 70% to 80%, for bolting and non-bolting accessions, respectively (Olas-Sochacka, 2017). According to the authors, 100 garlic explants (per genotype) are sufficient for the safe long-term preservation of the species in LN.
Forest Trees
The cryopreservation of valuable embryogenic lines is an important tool for the production of somatic seedlings of coniferous species, both for commercial purposes and in pilot silvicultural programs in countries, such as the United States, Canada, New Zealand, and Sweden (Egertsdotter et al., 2019; Lelu-Walter et al., 2013; Y. S. Park, 2002). This procedure allows for the storage of the best tree genotypes previously selected in experimental plots in the form of embryogenic tissues, followed by the thawing and regeneration of seedlings under in vitro cultures. This helps to preserve valuable gene resources of forest trees ex situ. The cryopreservation of embryogenic tissues has been successful in tree species, such as holm oak (Quercus ilex L.) (Barra-Jiménez, 2015), pedunculate oak (Quercus robur L.) (Martínez et al., 2003), and black pine (Pinus nigra Arn.) (Salaj et al., 2007) or hybrid firs (Abies alba × A. cephalonica, Abies alba × A. numidica) (Salaj et al., 2010).
In Poland, positive embryogenic tissue cryopreservation results were obtained at the Institute of Dendrology PAS for the following conifer species: Picea abies (L.) H. Karst, Abies alba Mill., Larix decidua Mill. (Hazubska-Przybył et al., 2013; Szczygieł, 2005), Abies nordmanniana (Steven) Spach (Nawrot-Chorabik & Sitko, 2014), and Picea omorika (Pančić) Purk. (Hazubska-Przybył et al., 2010). Primarily, embryogenic tissues were introduced into the LN because they are the most convenient material for cryopreservation in this group of plants. For this purpose, the slow or fast freezing technique (Nawrot-Chorabik & Sitko, 2014; Szczygieł, 2005) and the technique of gradual tissue dehydration (Hazubska-Przybył et al., 2010, 2013) were used. Szczygieł (2005) demonstrated the effectiveness of the slow freezing technique for embryogenic cultures of P. abies (L.) H. Karst, A. alba, and L. decidua of native origin. However, cryopreservation efficiency was clearly dependent on the tree species and tissue genotype and ranged from to 86%–100% for P. abies, 67%–100% for L. decidua, and 50% for A. alba. The possibility of the cryopreservation of somatic embryos of P. abies and L. decidua by direct immersion in LN after drying has also been demonstrated. Nawrot-Chorabik and Sitko (2014) revealed a high efficiency for the cryopreservation of A. nordmanniana embryogenic tissues using a protocol based on the rapid freezing and thawing of tissues and the use of ABA instead of harmful DMSO as a cryoprotectant. In contrast, Hazubska-Przybył et al. (2010, 2013) obtained high levels of P. omorika tissue survival (ca. 100%) and slightly lower P. abies tissue survival (ca. 55%), as well as plant regeneration after cryopreservation (Figure 2), using a stepwise desiccation method. Embryogenic tissues before freezing in LN were treated with increasing concentrations of sucrose (0.25–1.0 M) for 7 days and air-dried over silica gel to approximately 20% water content.
Figure 2
Somatic embryos of Picea omorika regenerated in vitro after cryopreservation: (A) at the cotyledonary stage; (B) at the germination stage; (C) converted into somatic seedlings. ET – embryogenic tissue.
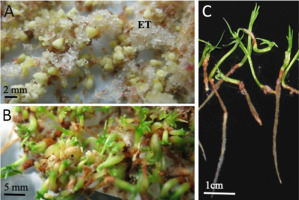
The cryopreservation of embryogenic tissue has also been successfully developed for one of the broadleaf species, Quercus robur (Figure 3). Cultures were established from immature zygotic embryos to initiate somatic embryogenesis. The optimum time for zygotic embryo development is from 26th July to 1st August in Wielkopolska, Poland (Chmielarz, 1999). The cryopreservation of Q. robur embryogenic calli was successful after pretreatment of clumps (Chmielarz et al., 2005). Before cryostorage, clumps were pre-treated on solid agar media with increasing concentrations of sucrose and glycerol, followed by air desiccation. After the rapid cooling of clumps in tightly closed vials to −196 °C, 24 hr of storage, and fast thawing at 42 °C, embryogenic calli were regenerated (Figure 3). Together with a group of French scientists (Chmielarz et al., 2005), the cryogenic treatment of the Q. robur embryogenic tissues consisting of preculture on the solid medium with an increasing concentration of sucrose (0.25 M for 1 day, 0.5 M for 1 day, 0.75 M for 2 days, and 1.0 M for 3 days), followed by the drying of clumps over silica gel to 17.3% (Figure 3), was shown to effectively protect the Q. robur embryogenic tissue during cooling to the temperature of LN (Chmielarz et al., 2005).
The successful cryopreservation of the embryogenic tissues of forest tree species (Picea spp., Quercus spp.) was possible using the same tissue pretreatment method. Before cooling in LN, embryogenic tissue was precultured on solid agar medium with an increasing concentration of sucrose followed by air desiccation to the species-specific moisture content. After thawing, the tissue was regenerated in vitro under special growth conditions: broadleaved species (oak) in light (16-hr day / 24 hr) and coniferous (spruce) in darkness (24/24 hr).
Figure 3
Clumps of Quercus robur embryogenic tissue: (A) desiccated over silica gel were added for 3 hr: (B,C) survived (yellow) and dead (black) after cryopreservation; (D) mature somatic embryos with cotyledons; (E) somatic embryos germinated into plantlets with shoots, leaves, and roots (Chmielarz et al., 2005).
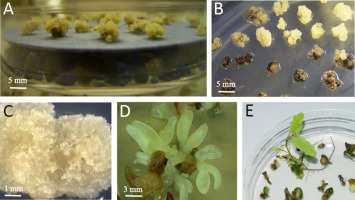
Ferns
Ferns are a group of spore plants that exhibit two independent life forms: gametophytes and sporophytes. Gametophytes, although small and inconspicuous, are excellent materials for the mass in vitro propagation and ex situ conservation of ferns (Ballesteros & Pence, 2018; Pence, 2018). The establishment of an efficient multiplication procedure for Cyathea australis Domin in 1995 at PAS BG-CBDC (Goller & Rybczyński, 1995) resulted in a large in vitro collection of various tree fern species at PAS BG-CBDC (Goller & Rybczyński, 2007). The need for long-term maintenance has prompted research to develop a cryopreservation procedure for gametophytes. Cyathea australis was the first fern species for which this procedure has been described (Mikuła et al., 2009; Mikuła & Rybczyński, 2006). Various cryopreservation methods, such as vitrification (PVS2 and PVS3), encapsulation-vitrification, and encapsulation-dehydration with or without a 7- or 14-day preculture on ABA, were tested for their effectiveness in preserving the viability of a wide range of fern species (Makowski, 2013; Mikuła, Makowski, et al., 2011). The encapsulation-dehydration with preculture on ABA, reduced size of alginate beads from 5 mm to 2.5 mm, and 7 days of darkness during the recovery of post-thaw cultures of gametophytes provided the best results (Makowski et al., 2015). This protocol was subsequently used to prepare the gametophytes of other fern species for long-term banking in the LN (Figure 4). Finally, 13 of the 15 fern species studied were frozen at LN for more than 10 years (Zimnoch-Guzowska et al., 2022). Their survival rates were monitored and remained unchanged.
Although the fern species studied grow in drastically different environmental conditions from aquatic species [e.g., Ceratopteris thalictroides (L.) Brongn.] in tropical and submontane rain forests (e.g., Cyathea delgadii Sternb.) to serpentine (e.g., Asplenium adulterinum Milde) habitats, cryopreservation by encapsulation-dehydration consistently protected their gametophytes, resulting in a survival rate of over 63%. After improving the pre- and post-cryopreservation treatments, the survival rates of as many as 11 species, including two water fern species, reached over 90% (Figure 4). The pre- and post-cryopreservation treatments reduced the incidence of necrosis in gametophytes recovered after storage in LN (Mikuła, Makowski, et al., 2011).
Figure 4
Fern species cryopreserved as gametophytes using the encapsulation-dehydration technique. Gametophytes were precultured for 2 weeks with 0.25 M sucrose and 10 µM abscisic acid (ABA). Alginate beads were 5 mm or 2.5 mm in size. Incubation for 7 days in the dark was applied during the recovery culture of small beads. With Ceratopteris cornuta (P. Beauv.) Lepr. and Dicksonia sellowiana (Pr.) Hook., only small capsules were used. Species were categorized by ecogeographic region of origin: first group – water ferns of the humid tropics; second group – ferns of tropical and submontane rain forest; third group – mild, subfreezing winters; fourth group – temperate species with winter temperatures as low as −32 °C.
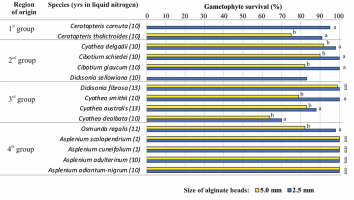
In Osmunda regalis L., a powerful strategy for fern propagation and conservation has been developed using tissue culture and cryopreservation (Makowski et al., 2016). Gametophytes of this species grown in vitro and frozen in LN can be used to restore or reinforce wild depauperated populations. The whole system of micropropagation starting from spores, through the cryopreservation of multiplied gametophytes and the restoration of gametophyte culture, to sporophyte production and their acclimatization to ex vitro conditions, can be completed in approximately 8 months.
. Final Remarks
To ensure the sustainable and practical use of cryopreservation, basic knowledge of storage protocols must be combined with greater awareness of the implementation and application of cryobanking technologies for different plant species. Although the number of species tested in Poland is not large (Table 3), the results obtained also have practical applications.
Table 3
Plant species for which cryopreservation protocols of tissues have been developed in Polish laboratories.
Plant species | Plant material | Cryo-technique | Survival (%) | References |
---|---|---|---|---|
Ornamental plants: | ||||
Chrysanthemum ×morifolium (Ramat.) Hemsl. | Shoot tips | E-D | 67 | Kulus, Abratowska, and Mikuła (2018) |
C. pacificum Nakai | Shoot tips | E-D | 77.8 | Kulus and Abratowska (2017) |
Galanthus elwesii Hook. f. | Apical meristems | D-V | 96.7 | Maślanka et al. (2013) |
Gentiana capitata Buch.-Ham. ex D. Don | PEM | E-D | 100 | Tomiczak and Markowski (2021) |
G. cruciata L. | PEM | E-D | 83 | Mikuła et al. (2008) |
G. decumbens L. f. | PEM | E-D | 100 | Tomiczak and Markowski (2021) |
G. kurroo Royle | PEM | E-D | 95 | Mikuła, Tomiczak, et al. (2011) |
G. pannonica Scop. | PEM | E-D | 70 | Mikuła p.c., 2022 |
G. tibetica King ex Hook. f. | PEM | E-D | 68 | Mikuła et al. (2008) |
Lamprocapnos spectabilis (L.) Fukuhara | Shoot tips | E-V | 73.1 | Kulus (2020b) |
Narcissus ‘Carlton’ | Somatic embryos | D-V | 100 | Maślanka et al. (2016) |
Rosa agrestis Savi | Apical buds | D-V | 50.5 | Pawłowska and Szewczyk-Taranek (2015) |
R. canina L. | Apical buds | D-V | 78.0 | Pawłowska (2012) |
Dormant buds | D-V | 96.0 | Pawłowska and Szewczyk-Taranek (2014) | |
R. dumalis Bechst. em. Boulenger | Apical buds | D-V | 71.4 | Pawłowska and Szewczyk-Taranek (2015) |
R. pomifera ‘Karpatia’ | Dormant buds | D-V | 84 | Kwaśniewska et al. (2017) |
R. rubiginosa L. | Apical buds | D-V | 63.2 | Pawłowska and Szewczyk-Taranek (2015) |
R. rubiginosa L. | Dormant buds | D-V | 93.5 | Pawłowska and Szewczyk-Taranek (2014) |
Tulipa tarda Stapf. | Apical meristems | D-V | 100 | Maślanka and Szewczyk (2021) |
Viola stagnina Kit. | Adventitious shoot apices | E-D | 64.7 | Żabicki et al. (2021) |
Crop species: | ||||
Allium sativum L. | Shoot tips | V | >30 | Olas-Sochacka (2017) |
Solanum tuberosum L. | shoot tips | V | 38.5/10–70 | Smyda-Dajmund (2017) |
Forest trees: | ||||
Abies alba Mill. | Embryogenic tissue | Slow freezing | 50 | Szczygieł (2005) |
A. nordmanniana (Steven) Spach | Embryogenic tissue | Rapid freezing | 65 | Nawrot-Chorabik and Sitko (2014) |
Larix decidua Mill. | Embryogenic tissue | Slow freezing | 67–100 | Szczygieł (2005) |
Picea abies (L.) H. Karst. | Embryogenic tissue | Slow freezing | 86–100 | Szczygieł (2005) |
P. omorika (Pančić) Purk. | Embryogenic tissue | Stepwise desiccation method | 100 | Hazubska-Przybył et al. (2010, 2013) |
Quercus robur L. | Embryonic axes | D-V and two-step cooling | 96% (regrowth 14%) | Chmielarz (1997) |
Q. robur L. | Embryogenic tissue | Preculture on sucrose solution (0.25 M for 1 day, 0.5 M for 1 day, 0.75 M for 2 days, and 1.0 M for 3 days), followed by air desiccation | 50 | Chmielarz et al. (2005) |
Q. robur L. | Plumules | Cryoprotection (in 0.75 M sucrose, followed by 1.0 M sucrose and 1.5 M glycerol solutions), next desiccation over silica gel | 60 | Chmielarz et al. (2011) |
Q. robur L. | Embryonic axes | D-V | 50 | Nuc et al. (2016) |
Fagus sylvatica L. | Embryonic axes | D-V | 30 | Nuc et al. (2016) |
Ferns: | ||||
Asplenium adulterinum Milde | Gametophytes | E-D | 100 | Makowski (2013) |
A. adiantum-nigrum L. | Gametophytes | E-D | 100 | |
A. cuneifolium Viv. | Gametophytes | E-D | 100 | |
A. scolopendrium L. | Gametophytes | E-D | 100 | Mikuła, Makowski, et al. (2011) |
Ceratopteris cornuta (P. Beauv.) Lepr. | Gametophytes | E-D | 95 | Mikuła p.c., 2022 |
C. thalictroides (L.) Brongn. | Gametophytes | E-D | 91 | Makowski et al. (2015) |
Cibotium glaucum (Sm.) Hook. & Arn. | Gametophytes | E-D | 82 | Mikuła, Makowski, et al. (2011) |
C. schiedei Schltdl. & Cham. | Gametophytes | E-D | 90 | |
Cyathea australis Domin. | Gametophytes | E-D | 83 | |
C. dealbata (G. Forst.) Sw. | Gametophytes | E-D | 68 | |
C. delgadii Sternb. | Gametophytes | E-D | 92 | |
Somatic embryos | E-D | 16 | Tomiczak et al. (2018) | |
C. smithii Hook. f. | Gametophytes | E-D | 79 | Mikuła, Makowski, et al. (2011) |
Dicksonia fibrosa Colenso | Gametophytes | E-D | 99 | |
D. sellowiana (Pr.) Hook. | Gametophytes | E-D | 83 | Mikuła p.c., 2022 |
Osmunda regalis L. | Gametophytes | E-D | 82 | Mikuła, Makowski, et al. (2011) |
Studies conducted by Polish scientists have shown that encapsulation-dehydration is the best technique for the fast restoration of suspension cultures after cryopreservation. It can be used to rejuvenate cultures and restore their regenerative potential. With some limitations, the TTC test can be successfully applied for the early prediction of post-thaw viability. Cryopreservation is now routinely used at PAS BG-CBDC for the storage of PEMs of the three Gentiana species. Tissues showed no significant decrease in viability after 15 years of cryostorage. Cryopreservation reduces maintenance activities, provides the best balance between effort and cost to maintain cell suspension collection, and ensures embryogenic and genetic stability. Studies have also shown that the application of non-conventional additives during various stages of cryotreatment may greatly affect its efficacy. Nanoparticles added to the alginate bead matrix appear to be particularly promising because they significantly increase the survival of LN-stored explants. Further research in this area is recommended, especially with respect to the type, size, and shape of the NPs.
Research on ornamental plants, such as chrysanthemum, has shown that cryoinjury to the meristematic dome may affect the morphogenetic response of shoot tips by leading to callus development, shoot malformation, and rooting inhibition. These events are critical for plant chimeras and that are widely represented in ornamental plants. However, it was possible to develop an encapsulation-dehydration protocol that enables safe and long-term storage of periclinal chimeras (and other cultivars obtained via radiomutation) without separating their genetic components. Similarly, in the bleeding heart, encapsulation-vitrification is the most optimal approach in terms of plant material survival and recovery, as well as maintaining its genetic integrity. Droplet/vitrification is highly effective for roses and ornamental geophytes. These studies have allowed for the development and verification of protocols for cryogenic gene banks that guarantee not only high survival and regeneration but also the stability of the regenerants. In geophytes, this is the first step towards developing protocols for the protection of ornamental bulbs in cryobanks.
Effective protocols for the cryopreservation of shoot tips developed in Poland have allowed for the establishment of collections of the most valuable genetic resources of potato and garlic, which were stored in PBAI-Młochów RC (Młochów) and InHort (Skierniewice). The cryopreservation of gametophytes provides the opportunity to preserve fern diversity for many years (including tree ferns), as well as the rapid and mass restoration of cultures and plants according to needs. This was accomplished using a cryobank at PAS BG-CBDC (Warsaw). Protocols developed for the cryopreservation of embryogenic tissues of forest tree species of the genera Abies, Larix, Picea, and Quercus are ready for use in the cryobank, and the protocol developed for Fraxinus has been successfully applied at the Kostrzyca FGB.