Introduction
Approximately 8% of global plant species are threatened with extinction. This is one of the environmental consequences of increasing human population and urbanization that has led to habitat loss and fragmentation, overexploitation, alien species invasion, pollution, and climate change (Chapin et al.,2000; Miller et al.,2012). Some plants are also threatened by inherent reproductive obstacles (e.g., low pollination success and low germination rates) (Cerabolini et al.,2004; Cursach & Rita,2012; Gaudeul & Till-Bottraud,2004; Massey & Whitson,1980). It is generally accepted that the primary conservation strategy should focus on populations in their natural habitats, i.e., in situ conservation (Volis,2017). For many species this method is insufficient, and ex situ actions are introduced, which provide a second line of security for rare plants by allowing specimens to be grown in the absence of natural environmental challenges (Burney & Burney,2009). There is an increasing need to integrate in situ and ex situ conservation planning to ensure that, whenever appropriate, ex situ conservation is used to support in situ conservation to the best effect possible (IUCN/SSC,2014).
Primula farinosa L. is a Eurasian species with a highly irregular range in Europe that spans across southeastern Scandinavia, the plains on the eastern coast of the Baltic Sea, and the mountains of Central and Southern Europe (Gajewski et al.,2018; Kaźmierczakowa,2014). Scattered European lowland locations are of relict character and mostly historic; therefore, in much of its European range, this species is considered to be endangered. It has been deemed extinct in the Czech Republic (Grulich,2012), and endangered in Germany, Lithuania, and Kaliningrad Oblast (Kaźmierczakowa,2014). There is also concern for its Carpathian populations, as the species is extinct in the Ukrainian Carpathians, critically endangered in Hungary, and endangered in Slovakia and Romania (Witkowski et al.,2003). In Poland, P. farinosa is subject to legal protection, and is included in the Polish red book of plants as a critically endangered species (CR category) (Kaźmierczakowa,2014), in the Polish Red List of Pteridophytes and Flowering Plants under category E – disappearing species, critically endangered (Kaźmierczakowa et al.,2016), and in the Red Book of the Polish Carpathians as a critically endangered species (Kaźmierczakowa,2008).
In Poland, P. farinosa is found only on the southern edge of the Radziejowa Range, within the Poprad Landscape Park and in the Natura 2000 – Ostoja Popradzka area (PLH 120019). The habitat of P. farinosa is an alkaline fen (habitat 7230), classified in phytosociological terms as Valeriano-Caricetum flavae Pawł. association. The population size of this species has been rapidly declining in recent decades, and in 2011, it reached the critical level of 158 flowering specimens (Gajewski et al.,2013). As a result, projects for active conservation of this species have been developed. In situ activities are aimed at improving growing conditions, and involve single mowing with crop removal, extensive sheep grazing, and local water damming (Gajewski et al.,2013). For the purpose of ex situ protection, seeds from the Polish population have been deposited in a seed bank at the Center for Biological Diversity Conservation, in Powsin (Kapler et al.,2013). Another aim of the active conservation project was to strengthen the existing population, to create secondary localities with plants propagated from seeds, and to establish collections at botanical gardens using plant tissue culture tools. A genetic analysis proved that the sole remaining P. farinosa population in Jaworki is genetically distinct from its nearest Slovak counterparts, and thus, all plants used in reintroduction interventions should be produced using only locally collected seeds (Gajewski et al.,2018).
Primula farinosa is a small, rosette-shaped perennial belonging to hemicryptophytes. In May, it develops distylic flowers (long-styled and short-styled morphs) clustered on top of a leafless stem. Fruits, in the form of multiseeded capsules, ripen in July. Preliminary attempts at seed propagation of primrose in a greenhouse proved ineffective due to low germination rate (despite laboratory-confirmed vitality) (Gajewski et al.,2013), slow seedling growth and rosette formation (about 4 months from seeding to replanting-suitable plants), and high (ca. 50%) mortality rate of juvenile plants.
In vitro propagation is considered to be a complimentary conservation tool (Saransan et al.,2006), and has successfully been used to protect many species of the genus Primula, including rare and endemic species (P. scotica, P. heterochroma, P. cuneifolia var. hakusanensis) (Benson et al.,2000; Sharaf et al.,2011; Shimada et al.,1997) as well as those with decorative and/or medicinal (P. veris, P. vulgaris, P. pubescens, P. forbesii) properties (Hayta et al.2016; Jędrzejczyk et al.,2018; Jia et al.,2014; Morozowska & Wesołowska,2004; Okršlar et al.,2007; Schween & Schwenkel,2002,2003; Takihira et al.,2007). Depending on the propagation objective, different explant types and cultivation methods were used. The initial material included apical meristems of seedlings grown from seeds of P. scotica, P. veris, and P. heterochroma (Benson et al.,2000; Jędrzejczyk et al.,2018; Morozowska & Wesołowska,2004; Sharaf et al.,2011) sown on appropriate media from which shoot cultures were derived. Callus cultures originated from the petiole (Schween & Schwenkel,2002,2003) and leaf explants of P. vulgaris (Hayta et al., 2016), secondary explants of P. veris, P. cuneifolia var. hakusanensis, and P. pubescens (Okršlar et al.,2007; Shimada et al.,1997; Takihira et al.,2007), and P. forbesii anthers were used to raise haploid callus lines (Jia et al.,2014).
The aim of our study was to develop an efficient method of propagation of the endangered Polish species P. farinosa from seeds sown in vivo and in vitro. The specific objectives of this work were as follows: (i) optimization of germination conditions for P. farinosa seeds, (ii) acceleration of seedling development and improvement of plant material quality via inoculation with mycorrhizal preparations, (iii) in vitro plant propagation, (iv) quality comparison of plants obtained from seeds and acclimated micropropagated plants.
Material and Methods
Plant Material
Primula farinosa seeds were obtained from the only natural location in Poland, with the consent of the Regional Directorate of Environmental Protection in Krakow, during fruit ripening in 2011, 2012, and 2013.
Germination Optimization
The ability of seeds to germinate was assessed in the germination test (International Seed Testing Association,1999). We evaluated the effects of factors that break dormancy – gibberellins and stratification. Stratification was carried out for 6 weeks at +4 °C in sealed Eppendorf tubes (dry stratification; DS), or in open test tubes placed in moist sand (wet stratification; WS). Gibberellic acid (GA3) solution at a concentration of 1.8 × 10−3 M (Morozowska,2002) was used to soak seeds overnight before placing them on moist blotter paper. The experiment consisted of six combinations: C, DS, WS, GA3, DS+GA3, and WS+GA3, with seeds soaked in water serving as the control (C). The germination ability was evaluated for three subsequent years. Four Petri-dishes containing 10 seeds were used for each combination. The seeds were germinated in a phytotron under 16/8-hr day/night photoperiod and photon flux density of 70 μmol m−2 s−1, at 24 ± 2 °C.
The number of seeds used in the germination test was limited because our priority was to obtain the greatest number of plants from a restricted pool of available seeds.
The ability of seeds to germinate in different conditions was evaluated after 28 days. In 2012, observations noted every few days enabled us to trace the dynamics of germination. The most effective seed treatment was used to produce plants in further experiments. The seeds were sown in boxes with peat substrate and deacidified peat (1:1), used as a standard substrate mixture for P. farinosa.
Growth in Controlled Conditions and Mycorrhization
In 2018, seed-derived juvenile rosettes at the stage of 4–5 leaves (Figure 1A) were replanted individually into pots of 7-cm diameter containing sterilized substrate. During planting, mycorrhizal vaccines Mykoflorin, for flowers and vegetables (Mykoflor, Poland), and Agrecol, with a hydrogel (Agrecol, Poland), were applied at a rate of 1.5 g and 20 mL per plant, respectively. Nonmycorrhized seedlings served as a control. The endomycorrhizal fungi included in Mykoflorin were Rhizophagus aggregatus N. C. Schenck & G. S. Sm. Walker, Rhizophagus intraradices (N. C. Schenck & G. S. Sm.) C. Walker & A. Schüßler, Claroideoglomus etunicatum (W. N. Becker & Gerd.) C. Walker & A. Schüßler, Funneliformis mosseae (T. H. Nicolson & Gerd.) C. Walker & A. Schüßler, Funneliformis caledonius (T. H. Nicolson & Gerd.) C. Walker & A. Schüßler, and Gigaspora margarita W. N. Becker & I. R. Hall, as specified by the manufacturer. The composition of Agrecol was not provided by the manufacturer.
Figure 1
Ex situ propagation of Primula farinosa: seed-derived plants (A,C,D) and left side in (B) and micropropagated plants (E–H) and right side of (B). (A) Six-week-old seedlings after cultivation in growth chamber. (B) Comparison of seed-derived (left side) and in vitro-derived (right side) plants at the end of first year of cultivation in open air collection. (C) Blooming seed-derived plants inthe second year of cultivation in the collection. (D) Seed-derived plants before planting on the secondary locality (2016). (E) Rooting shoots after 5 weeks of cultivation on MS medium supplemented with 0.1 mg dm−3 IBA. (F,G) Transient abnormalities of generative organs in the year of acclimatization of in vitro-derived plants: altered morphology of corolla of flowers (F); gemmae within inflorescence (G). (H) In vitro-derived plants in the collection of the Botanical Garden in Krakow (2014).
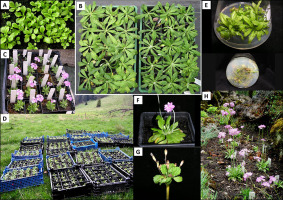
Each combination included 25 plants representing five replications. The plants were cultivated for 5 weeks in Sanyo vegetative chambers, under 16/8-hr day/night photoperiod and photon flux density of 45 μmol m−2 s−1, at 18/16 °C, with humidity gradually decreasing from 90% to 55%, and then in a nonheated greenhouse. Eleven weeks after planting, biometric measurements of aboveground parts were recorded and fresh weight was evaluated for each plant. Root system development was assessed according to the following scale: 1 – root system accounts for less than 25% of the pot volume; 2 – 25–50%; 3 – above 50%. Inoculation efficiency was assessed under a microscope.
Microscopic Research
The root samples were prepared according to the modified Phillips and Hayman method (Phillips & Hayman,1970). The roots were washed with tap water to remove any soil particles, and then softened in 10% KOH for 24 hr, washed again with water, acidified in 5% lactic acid for 1 hr at room temperature, and stained with 0.01% aniline blue in pure lactic acid for 24 hr. After staining, the roots were cut into 1-cm pieces, mounted on slides in glycerol, squashed slightly, and examined using Axio Imager M2 microscope (Zeiss) with AxioVision software. Ten randomly selected root fragments were evaluated for each of the 25 plants in all combinations.
Micropropagation
Seeds were surface disinfected by dipping in 70% (v/v) ethanol for 30 s, and then in 0.1% (w/v) mercuric chloride (HgCl2) solution for 2 min. After rinsing three times with sterilized water, the seeds were sown on Murashige and Skoog (MS) basal medium (Murashige & Skoog,1962) supplemented with 20 g dm−3 of sucrose, 1.0 mg dm−3 GA3, and 7.5 g of agar, and with a pH of 5.8. Shoot tips (approximately 1.5 cm in size) with 3–4 leaves were excised from the seedlings and transferred onto a proliferation medium for multiplication. The experiment was conducted using a mixture of shoots representing five clones. Shoot proliferation was carried out on MS medium supplemented with different concentrations of the cytokinin 6-benzyl-aminopurine (BAP; 0.1 or 0.2 mg dm−3) and the auxins indole-3-acetic acid (IAA; 0.1 or 0.2 mg dm−3) or indole-3-butyric acid (IBA; 0.05 or 0.1 mg dm−3). After 5 weeks of culture, the rate of shoot propagation (multiplication coefficient) and quality of the rosettes (size of shoots and leaves) were assessed. Multiplication coefficient was determined as the number of newly differentiated shoots divided by the number of shoots planted on medium at the beginning of the subculture.
Single rosettes were rooted in the MS medium supplemented with 0.1 mg dm−3 of IAA, α-naphthalene-acetic acid (NAA), or IBA. Rooting efficiency was evaluated after 5 weeks of culture. Propagation and rooting cycle were repeated three times, in four 100-mL Erlenmeyer flasks with five explants each, with one flask being a statistical repetition. All propagation steps were performed in a phytotron, under photoperiod conditions of 16/8-hr day/night and photon flux density of 70 μmol m−2 s−1, at 24 ± 2 °C.
Rooted plants transferred into pots were acclimated in a growing chamber under conditions similar to the mycorrhized seedlings.
Comparison of Seed-Derived and Micropropagated Plants
Plants obtained via the two methods (seeding and in vitro) were compared, using a group of fifteen individuals, with five plants for each of the three repetitions. Micropropagated plants represented five different clones. For 3 years, plants were grown in pots of 7-cm diameter in field conditions (Live Plant Collection of the University of Agriculture in Krakow, Poland). The first year was devoted to the production of plant material, at the end of which plants from both groups reached similar size (Figure 1B). In the second and third year after planting, selected morphological features and pollen viability were assessed. Pollen viability analysis was based on staining (Alexander,1969). In both plant groups, we evaluated three mixtures of pollen collected from five different plants. Microscopic observations were made using 250–300 pollen grains for each evaluation. We also assessed the germination ability of plants produced ex situ, for C and GA3+WS combination, as previously described. Plant ploidy analysis based on flow cytometry was performed in the Cytogenetics Laboratory of the Kutno Sugar Beet Breeding Station. The ploidy level was determined for samples of young leaves collected separately from each of the 15 plants in both combinations and prepared according to the Galbraith method (1989) modified by Thiem and Śliwińska (2003). Chopped plant material in 2 mL of lysis buffer added with fluorochrome dye DAPI was filtered and analyzed using PAII cytometer. In each sample, 1,520–1,720 nuclei were assessed. Plants of seed-origin served as a diploid reference.
Results
Germination Optimization
Germination ability of P. farinosa seeds in control conditions was low, with an average of 13.3%. Conditioning in gibberellin, especially when combined with stratification, significantly improved this ability (Table 1). Gibberellin treatment also accelerated the germination process. In GA3+WS and GA3+DS variants, all seeds germinated after 3 or 5 days, while the first control seedlings emerged only after 7 days (Figure 2).
Growth in Controlled Conditions and Mycorrhization
Survival rate of the replanted plants grown under controlled conditions was 100% in all combinations. Inoculation with mycorrhizal fungi did not increase the dimensions of aboveground parts (Table 2), whereas the treatment with Mykoflorin inhibited plant growth. After 11 weeks of cultivation in presence of Mykoflorin, the plants developed lower number of rosettes of reduced size. Agrecol treatment yielded the most homogeneous material, while plants treated with Mykoflorin appeared to be in the worst condition (Figure 3A–C). Control plants developed the most extensive root system (Figure 3D).
Microscopic observations revealed no effects of inoculation, and no arbuscular mycorrhizae were found (Figure 3E). Nonmycorrhizal fungal associations were present in four root samples. These were: resting spores of Olpidium sp. in the roots of a Mykoflorin-treated plant, microsclerotia of a dark septate endophyte in an Agrecol-treated plant, hyphae of endophytes in a control plant, and pathogenic fungi forming singular septum in a Mykoflorin-treated plant (Figure 3F). Plants with fungal associations on the roots did not show any difference in their morphology.
Table 2
Effect of mycorrhization on quality parameters of Primula farinosa plants.
Figure 3
Primula farinosa plants after cultivation in controlled condition and mycorrhization. (A–C) The morphology of plants. (D) Root systems. (E,F) Microscopic observation of roots after mycorrhization with Mykoflorin: not colonized (E); with fungi of nonmycorrhizal character (F). Roots were stained with aniline blue.
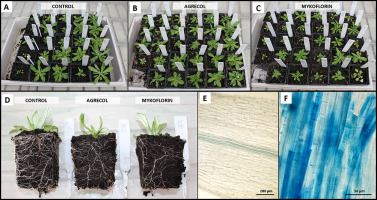
Micropropagation
The efficiency of shoot production in the tissue cultures was significantly affected by the concentration of plant growth regulators in the MS medium (Table 3). Propagation rate ranged between 2.1 and 4.5, with the greatest being recorded on the medium with the lowest dose of growth regulators. In all combinations, both the mean size of shoots and the number of leaves per plant were not significantly different. Rooting efficiency of rosettes was high on all media (Table 4, Figure 1E) regardless of the auxin used, and further simultaneous propagation was observed on all rooting media.
Table 3
Shoot multiplication of Primula farinosa on MS medium supplemented with BAP and IAA or IBA after 5 weeks of culture.
Comparison of Seed-Derived and Micropropagated Plants and Their Collection
In the first year of assessment, both groups of plants did not differ significantly, except for the number of flowers per inflorescence, which was higher in plants grown from seeds. In the following vegetative season, the groups did not differ in any of the analyzed parameters (Table 5). Irrespective of plant origin, pollen viability was high in both years. The percentage of germinating seeds in the control variant was the same in plants obtained from seeds and from in vitro cultures. In both cases, treatment with GA3+WS considerably improved the seed germination ability (Table 6).
Table 5
Comparison of seed-derived and micropropagated plants of Primula farinosa in 2014 and 2015.
Table 6
Germination ability of Primula farinosa seeds (%) collected in ex situ conditions from seed-derived and micropropagated plants after application of gibberellin and wet stratification in 2015.
Origin | Control | WS + GA3 | Average |
Seeds | 54.0 a | 90.7 b | 72.4 A |
In vitro | 52.7 a | 85.5 b | 69.1 A |
Average | 53.5 A | 88.8 B |
Results of flow cytometric analysis presented on DNA histograms (Figure 4) revealed a distribution of relative DNA content with a single dominant peak corresponding to the 2C level in G1 phase of the cell cycle. It indicates that there were no dividing cells in the portions of leaves sampled. Nuclear DNA content was the same in all analyzed plants and did not differ between seed-origin and micropropagated plants of P. farinosa, indicating that they were all diploids.
Figure 4
Histogram of relative DNA content in the nuclei of Primula farinosa leaf. Seed-origin (A) and micropropagated (B) plant.
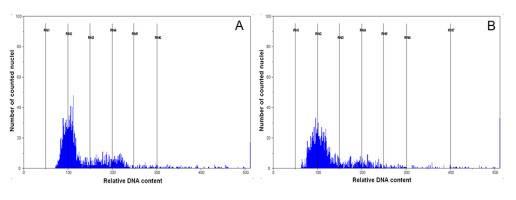
Over 1,300 plants grown from seeds sown into the substrate strengthened the parental population (in the years 2013–2015) and were used to create a replacement population (in the years 2016–2018) in the Beskid Sądecki (Figure 1D). Plants representing 12 in vitro propagated and acclimatized clones were transferred to botanical gardens in Krakow (Figure 1H), Lublin, Łódź, and Zakopane, in 2014, to create conservative collections of the species. The collection of the University of Agriculture in Krakow holds over 100 plants, yielding seeds every year.
Discussion
Objectives for P. farinosa Ex Situ Propagation
Active protection of P. farinosa in Poland has been advocated for a long time (Kaźmierczakowa,2000), and this plant is included in a group of 20 domestic species for which restitution of wild populations is most justified (Ziarnek,2017). Our preliminary observations and attempts made in domestic botanical gardens (Ziarnek,2017) indicated that propagation and cultivation of primrose in ex situ conditions are difficult. Specific requirements of the species in the early developmental stages are confirmed by the lack of juvenile plants at the natural site (Gajewski et al.,2013; Kaźmierczakowa,2000). Species reintroduction preferably uses plants grown from seeds obtained from a local wild population. This is particularly important when the population features a unique genetic composition, as described for P. farinosa at the site in the Beskid Sądecki (Gajewski et al.,2018). Primula farinosa growing at the only site in Poland still produces viable seeds, despite the fact that as a heterostylic species, it is likely to be pollinated by specific pollinating insects. It is also infested by Urocystis primulicola, a pathogen that limits the number of developed seeds (Gajewski et al.,2013; Kućmierz,1971).
Restricted availability of seeds and low survival rate of juvenile plants imply that we need effective methods allowing the best possible use of existing diaspores. Our study discusses the effectiveness of treatments introduced at early stages of propagation and a technique of micropropagation, and compares plants obtained using these two methods.
Optimization of Seed Germination
Seed germination behavior is an integral part of ex situ conservation, and understanding the germination requirements is the first step in the reinforcement of endangered plant species [e.g., Cerabolini et al., (2004); Cho et al., (2018); Dziurka et al., (2019)]. The ability of P. farinosa seeds to germinate, assessed in the germination test in consecutive years, was low, despite high (over 90%) seed viability confirmed in our previous work using tetrazolium (Gajewski et al.,2013). This led us to conclude that the seeds of P. farinosa were dormant. A factor that dramatically increased their ability to germinate was the application of GA3. This natural phytohormone is known for its ability to reduce the duration of seed dormancy, and for successfully stimulating seed germination in other Primula plants (Cerabolini et al.,2004; Morozowska,2002; Sharaf et al.,2011). Gibberellin is an antagonist of abscisic acid, and both hormones are crucial for germination control. Their proportions, and thus, the seed germination ability, may be considerably modified by external factors, such as temperature (Izydorczyk et al.,2018), due to which low or high temperature treatment is often used to stimulate germination (Arslan et al.,2011; Morozowska,2002). Our study demonstrated synergistic effects of low temperature in presowing stratification and GA3 treatment in P. farinosa seeds. As the seed dormancy depended on internal factors, we concluded that it was endogenous dormancy resulting from mutual proportions of growth regulators responsible for germination. The response of P. farinosa seeds to the treatments indicated their physiological dormancy, typical for plants of temperate zone (Baskin & Baskin,2004). This mechanism may be important in preventing early germination of seeds in summer or early fall, when the conditions are not conducive to the development of new plant generations.
Stratification combined with gibberellin increased the number of germinating seeds by more than 7 times when compared to the control. We achieved higher effectiveness of dormancy-breaking treatments in P. farinosa than described for P. veris by Morozowska (2002).
Seedling Development
Soil microorganisms, especially arbuscular mycorrhizal fungi (AMF), are considered crucial for proper plant performance. AMF may be useful in the development of effective methods for the maintenance and propagation of threatened plant species and may significantly improve the success of plant conservation actions (Bothe et al.,2010; Jurkiewicz et al.,2010; Zubek et al.,2009). Vesicular-arbuscular mycorrhizae have been reported in P. farinosa from the British Isles (Harley & Harley,1987), and Primula species have also been recommended for the use of mycorrhizal inoculum products in gardens and for floricultural crops (Azcòn-Aquilar & Barea,1997).
Cultivation of plants derived from P. farinosa seeds under controlled conditions was successful, despite inoculation failure. We obtained good quality material in a short time. Greenhouse or field cultivation is associated with considerable temperature fluctuations, often greater than at the natural mountainous site. Therefore, ensuring optimal thermal conditions during ex situ cultivation of juvenile plants seems crucial. This assumption is confirmed by ecological preferences of P. farinosa, which thrives in fairly cool climate, typical of lower subalpine forest or special lowland habitats (peatlands) (Zarzycki,2002).
Experimental mycorrhization is not always successful, and the degree of root colonization may vary from 5.3% to 80% (Bizabani et al.,2016; Navarro & Morte,2019). It may be negatively affected by mineral nutrient fertilization (Bizabani et al.,2016). In our study, plants were not fertilized; hence, this was not the cause of the low colonization rate. Another reason for unsuccessful inoculation might be that the species of mycorrhizal fungi contained in Agrecol and Mykoflorin preparations were not compatible with P. farinosa. Arbuscular mycorrhizae were not necessary for the development of P. farinosa ex situ; however, investigating the actual mycorrhizal status of the species at the site in the Beskid Sądecki appears to be a valid research challenge.
In Vitro Propagation
Micropropagation allows for rapid production of relatively large number of plants from a small amount of initial material. It is, therefore, a technique worth recommending for endangered species for which obtaining the initial material for reproduction is difficult. However, it should be kept in mind that it can stimulate genetic variability (Us-Camas et al.,2014), and thus, plants obtained through micropropagation should be carefully evaluated. Presently, this evaluation involves an array of molecular techniques (Moharana et al.,2018; Nalousi et al.,2019; Sharma et al.,2019); however, a complete comparison should also include ploidy assessment and qualitative features (Jędrzejczyk et al.,2018; Nalousi et al.,2019; Żabicki et al.,2019). Molecularly confirmed genetic fidelity only concerns fragments of the genome, not necessarily associated with trait inheritance. It does not provide sufficient information on changes in the regenerated plant ploidy. Despite molecular similarity, micropropagated plants may differ from the parental plants in qualitative traits (Us-Camas et al.,2014), and plants with confirmed genetic variability have the same reproductive potential as the parental plants and those from natural populations (Żabicki et al.,2019).
Our study presents a complete procedure of P. farinosa micropropagation from seeds sown on a sterile medium, and our ploidy analysis revealed no variability in the produced material. The propagation rate of 4.5 is comparable to the rates of shoot cultures of Primula genus (Morozowska & Wesołowska,2004; Sharaf et al.,2011), even though Benson et al. (2000) achieved higher values for P. scotica. The most effective propagation of P. farinosa occurred on the medium with the lowest concentration of growth regulators. The propagated shoots rooted easily, and new rosettes were formed on the media without cytokinins. This may indicate accumulation of cytokinins from previous passages or high levels of endogenous cytokinins that would facilitate propagation irrespective of growth regulator presence in the medium. Similar observations were reported for P. scotica (Benson et al.,2000), however, propagation effectiveness on a medium without growth regulators depended on the clone and was sometimes severely restricted. Primula pubescens and P. heterochroma did not require growth regulators for rooting (Sharaf et al.,2011; Takihira et al.,2007). The fact that a reduction in the phytohormone does not lower the propagation rate of P. farinosa is valuable considering the protection of biodiversity, because excessive concentrations of growth regulators in the media can increase the possibility of somaclonal variation.
Comparison of Seed-Derived and Micropropagated Plants
Jędrzejczyk et al. (2018) reported different effects of micropropagation in P. veris. Micropropagated plants of this species differed from the seed-derived plants, and the differences appeared to be permanent as they persisted for 3 years of the observations. A similar comparison in Cirsium pannonicum (L.f.) Link (Trejgell et al.,2012) demonstrated only transient nature of some differences and permanent nature of some others that appeared in all vegetative seasons. The plants of P. farinosa propagated in vitro showed abnormalities in their flowering phenology at the end of the first year. It could be because most of them bloomed or repeated blooming between August and October, while the seed-derived plants did not bloom at all in the first year (data not shown). We also noticed atypical morphology of generative organs – flowers with numerous petals in corolla (four per seven flowerings of one clone) or gemmae within inflorescences (three per 10 flowerings of another clone) (Figure 1F,G). Variability in the in vitro produced plants ceased in the third year of cultivation, indicating its transient nature.
The results confirming the generative reproduction capability of micropropagated plants are particularly important. Their pollen showed similar viability to their seed-derived counterparts, and the seeds had comparable germination ability. Interestingly, the percentage of germinating seeds harvested during collection was higher than that of seeds from the natural site, even though seed viability was comparable, as indicated by a similar number of germinating seeds following GA3+WS treatment. Postharvest dormancy was more common in seeds collected from the natural site.
The conditions of seed formation in the wild and in the collection differed in many aspects. The collected plants grew at a higher temperature but were shaded, while those at the natural site experienced lower temperatures but a greater intensity of solar radiation.
Effects of environmental conditions during seed production on seed dormancy and germination have already been described. Low temperature before seed maturation may interfere with ABA biosynthesis or signaling and can markedly increase the final depth of seed dormancy (Chahtane et al.,2017; Chen et al.,2014; Kar et al.,2019; Sharma et al.,2019; Trejgell et al.,2012; Żabicki et al.,2019). However, numerous studies have shown that seeds maturing at higher temperatures produce more impermeable seeds when compared with those growing in a low-temperature environment (Jaganathan,2016). Nitrate also appears to influence dormancy, although not as markedly as temperature does (Alboresi et al.,2005; He et al.,2014). Light quality during seed maturation and day length affect seed dormancy, with shorter days leading to thinner seed coats and seeds with higher germination ability at harvest (Penfield & MacGregor,2017).
From 3 years of observation, no differences were found between seed-derived and in vitro-derived plants of P. farinosa, both in the ploidy level and their reproductive abilities.
Conclusions
Our study developed effective protocols for P. farinosa propagation from seeds using two different methods that can be applied in practical species protection.
The seeds of P. farinosa were dormant, and stratification combined with gibberellin increased the number of germinating seeds.
Arbuscular mycorrhiza was not necessary for the development of P. farinosa ex situ, however, optimal thermal conditions during cultivation of juvenile plants were crucial.
The most effective propagation of P. farinosa occurred on the medium with the lowest concentration of plant growth regulators. The propagated shoots rooted easily, and new rosettes were formed on the media without cytokinins.
No differences were observed between seed-derived and in vitro-derived plants of P. farinosa, both in the ploidy level and their reproductive abilities.
Handling Editor
Beata Zagórska-Marek; University of Wrocław, Poland; https://orcid.org/0000-0001-6385-858X