. Introduction
Fungi of Candida spp. can be a part of the natural microbiota of immunocompetent individuals and may cause superficial and systemic fungal infections and fungemia. In recent years, there has been a rapid increase in the incidence of Candida infections. An estimated 750,000 people worldwide are affected by candidiasis annually, with a mortality rate of up to 40% (Ben-Ami, 2018; Bongomin et al., 2017). Symptomatic, invasive candidiasis concerns patients with severely weakened immune system, including those with cancer, organ transplantation, major abdominal surgeries, and implanted catheters as well as premature neonates and patients treated with aggressive chemotherapy or broad-spectrum antibiotics and corticosteroids (Ben-Ami, 2018; Yapar, 2014).
Candida albicans is the most commonly isolated species from patients. However, its frequency has been decreasing in recent years. The worldwide incidence of C. albicans has decreased from 73% in 1997 to 65% in 2007. In certain countries, this phenomenon is still being observed; for instance, the prevalence of C. albicans has decreased from 52% to 38% between 1999 and 2012 in the United States and from 51% to 45% between 2005 and 2013 in Spain (Guinea, 2014). However, there has been a gradual increase in the frequency of infections caused by species other than C. albicans, mainly C. glabrata, C. parapsilosis, and C. tropicalis (Ben-Ami, 2018; Guinea, 2014). There are differences in the prevalence of individual species depending on the type of predisposing factors; for example, C. parapsilosis is more common in newborns and young children than in adults, whereas C. glabrata is more common in stem cell recipients (Guinea, 2014).
It is becoming increasingly challenging to successfully treat patients with candidiasis, especially those with invasive candidemia (Sharafutdinov et al., 2020). The limited number of antifungal agents and their widespread use are thought to be the main factors causing drug resistance, which further restricts treatment options (Antonovics et al., 2007; Scorzoni et al., 2017).
Azoles (fluconazole – FCZ, itraconazole – ICZ, and voriconazole – VCZ) are the most commonly used antifungals. They are known to inhibit ergosterol biosynthesis via the cytochrome P450 enzyme 14-α demethylase, which catalyzes the conversion of lanosterol to ergosterol, thereby affecting the integrity of fungal cell membranes (Choi et al., 2016; Jensen, 2016; Scorzoni et al., 2017). There has been a very rapid increase in the prevalence of azole resistance, especially FCZ, in recent years, especially among species other than C. albicans. In particular, three species, namely, C. glabrata, C. parapsilosis, and C. tropicalis, are considered as FCZ-resistant (Berkow & Lockhart, 2017; Castanheira et al., 2017; Wiederhold, 2017). Azole resistance in Candida spp. has been associated with overexpression or mutations of ERG11, CDR1, and MDR1 genes, the products of which are active cell membrane transporters, “efflux pumps” (de Oliveira Santos et al., 2018). In fungi, two different types of drug efflux systems modulate azole resistance, the ATP-binding cassette (ABC) superfamily and major facilitator superfamily (MFS). Overactivity of efflux pumps can lead to multidrug resistance (MDR) or cross-resistance (Cowen et al., 2015; Jensen, 2016).
Cross-resistance can be defined as the acquired resistance to a certain antimicrobial agent after exposure to another drug (Figure 1). Cross-resistance can result from the induced expression of one of the ABC or MFS transporters by the first drug. After induction, ABC or MFS transporters can pump out another type of drug from the cell (Brody, 2016). An example of already detected cross-resistance between antifolates and azoles is the correlation between FCZ and methotrexate (MTX) in C. albicans (Ben-Yaacov et al., 1994; Kohli et al., 2001). MTX is frequently used in patients who are at an increased risk of mycosis. Indications for the use of MTX include acute lymphocytic leukemia in children, acute lymphoblastic and myeloid leukemia in adults, patients with breast, ovarian, cervical, testicular, lung, or bladder cancer, those with bone sarcoma, epithelium and chorionic adenoma, advanced mycosis fungoides, meningeal forms of leukemia and lymphoma, severe psoriasis, psoriatic arthritis, severe active rheumatoid arthritis, osteosarcoma, and non-Hodgkin’s lymphoma (Abolmaali et al., 2013; Bello et al., 2017; Huennekens, 1994; Polańska et al., 2016; Roszkiewicz et al., 2020, 2021).
Figure 1
Cross-resistance mechanism with the examples of fluconazole and methotrexate in Candida albicans. Fungal cell that has never been exposed to fluconazole acquires resistance to fluconazole after being exposed to methotrexate. This phenomenon occurs because both methotrexate and fluconazole leave the cell through the cell membrane transporters, “efflux pumps.” MTX – methotrexate; FCZ – fluconazole; CaMDR1 – membrane transporter.
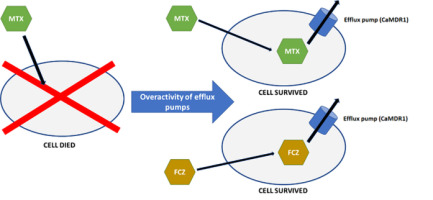
The cross-resistance between FCZ and MTX is mediated through the overexpression of CaMDR1 gene, which encodes an MFS protein (Ben-Yaacov et al., 1994; Jensen, 2016; Kohli et al., 2001). Whether the same mechanism is responsible for the cross-resistance between MTX and other azoles is, however, unclear. The available literature does not indicate whether analogous membrane transporters found in other species of the genus Candida can also transport MTX. Stimulation with MTX leads to the overexpression of MFS transporters, which may mediate resistance to FCZ and other azoles in strains that have never been in contact with azoles. Therefore, we decided to evaluate the possibility of cross-resistance between selected azoles and MTX for C. albicans and Meyerozyma guilliermondii (syn. Candida guilliermondii).
Meyerozyma guilliermondii is a relatively less studied species that can be a part of the natural microbiota; however, its prevalence is increasing among patients at the risk of mycosis. Some authors have indicated that M. guilliermondii as a re-emerging pathogen, especially in patients with cancer. The documented cases of infection indicate a mortality rate of up to 66% (Ahangarkani et al., 2019; Castillo-Bejarano et al., 2020; Tseng et al., 2018). In M. guilliermondii, cross-resistance between MTX and FCZ has never been observed before. As the species is considered to have a reduced sensitivity to azoles (Marcos-Zambrano et al., 2017), it is possible that it may acquire resistance more quickly following exposure to MTX.
The aim of this study was to determine the possibility of cross-resistance between azoles and MTX in selected strains of M. guilliermondii and C. albicans. We investigated this phenomenon using the azoles FCZ, VCZ, and ICZ.
. Material and Methods
In this study, 15 strains of M. guilliermondii (Wick.) Kurtzman & M. Suzuki were used, including one standard ATCC 6260 strain and 14 isolates from the collection of the Department of Biology and Parasitology. In addition, eight strains of C. albicans (C. P. Robin) Berkhout, including standard strains CBS 2312, L 45, and ATCC 24433 and five isolates from the collection of the Department of Biology and Parasitology, Medical University of Lodz were tested. Susceptibility of all strains for FCZ (Pol-Aura, 894BNE), VCZ (Pol-Aura, 544IED), and ICZ (Pol-Aura, 943YVR) was evaluated, and the minimum inhibitory concentrations (MICs) were determined in triple repetition before and after stimulation with MTX (Pol-Aura, 269QEF) using the EUCAST microdilution method (Arendrup et al., 2020; European Society of Clinical Microbiology and Infectious Diseases, 2020). The test range was as follows: FCZ, 0.125 to 64 mg/L; VCZ, 8 mg/L; and ICZ, 8 mg/L (European Society of Clinical Microbiology and Infectious Diseases, 2020). MIC values were interpreted according to EUCAST 2020 and CLSI M27 (Clinical and Laboratory Standards Institute, 2017; European Society of Clinical Microbiology and Infectious Diseases, 2020; Tseng et al., 2018). EUCAST 2020 did not provide any breakpoints for M. guilliermondii; therefore, based on Tseng et al. (2018), we determined the epidemiological cutoff values for non-wild isolates (resistant) as >8, >0.5, and >1 mg/L for FCZ, VCZ, and ICZ, respectively. For C. albicans, the following MIC breakpoints were applied: >4, >0.25, and >0.06 mg/L for isolates resistant to FCZ, VCZ, and ICZ, respectively (European Society of Clinical Microbiology and Infectious Diseases, 2020). Double stimulation with MTX was performed using the microdilution method in the test range of 0.0088 to 45 mg/L, which was developed based on the bacterial sensitivity to MTX (Nayak et al., 2019; Sulavik et al., 2001). Fungal cells of each strain that had been exposed to the highest non-inhibitory concentration of MTX were collected, multiplied, and used in further experiments. The data are presented as the average of three repeated measurements and their standard deviations. The median for each strain was determined using the interquartile range (IQR). Differences between MIC values before and after stimulation with MTX were assessed using the Wilcoxon signed-rank test, while the differences in MIC change between the species were assessed using the Kruskal–Wallis and Mann–Whitney U tests. Statistical analysis was performed using STATISTICA 13.1 software (StatSoft), and statistical significance was set at p < 0.05.
. Results
Meyerozyma guilliermondii
The studied strains showed various initial azole susceptibilities (Table 1). Stimulation of M. guilliermondii strains with MTX led to a significant increase in the median MICs for both FCZ and ICZ. The median MICs before and after stimulation were 9.333 (IQR: 0.667–64) and 64 mg/L (IQR: 64–64; p = 0.005) for FCZ; 0.917 (IQR: 0.583–1.333) and 1.667 mg/L (IQR: 1.333–2.667; p = 0.001) for ICZ, respectively. For VZC, the median MICs before and after stimulation were 3.5 (IQR: 1.008–6.333) and 3.167 mg/L (IQR: 1.359–4.167; p = 0.218), respectively.
Table 1
MIC values of the examined strains.
The differences in the MIC values before and after stimulation were statistically significant for FCZ and ICZ (p = 0.005 and 0.001, respectively) but not for VCZ (p > 0.05) (Figure 2). According to the epidemiologic cutoff values, 100%, 0%, and 62.5% of the previously susceptible strains became resistant to FCZ, VCZ, and ICZ, respectively, following stimulation with MTX.
Candida albicans
In the case of C. albicans strains, MTX stimulation led to a significant increase in the median MIC values for FCZ that showed a value of 0.917 mg/L (IQR: 0.667–1.083) before stimulation and 64 mg/L (IQR: 64–64 mg/L; p = 0.012) after stimulation and VCZ, as evident from a value of 0.344 mg/L (IQR: 0.122–1.208) before stimulation and 1.135 mg/L (IQR: 0.216–2.333; p = 0.018) after stimulation but not for ICZ. The MIC values for ICZ before and after stimulation were 4.333 (IQR: 2.333–6.002) and 4.667 mg/L (IQR: 2.667–6; p = 0.89), respectively.
The differences in the MIC values before and after stimulation were statistically significant for FCZ and VCZ (p = 0.012 and 0.018, respectively) but not for ICZ (p = 0.89) (Figure 3). According to the EUCAST breakpoints, 100%, 25%, and 0% of the previously susceptible strains were resistant to FCZ, VCZ, and ICZ, respectively, after stimulation with MTX.
. Discussion
Numerous studies have indicated resistance (including multi-drug resistance) or reduced sensitivity to antimycotics in species other than C. albicans (Berkow & Lockhart, 2017; Cretella et al., 2016). A typical example of a multidrug-resistant species is Candida auris. This species was first identified in 2009 in Japan and has been found in over 30 countries worldwide. Candida auris strains are characterized with high resistance to polyenes, azoles, nucleoside analogs, and echinocandins. Therefore, some C. auris strains can be considered pan-resistant (Ademe & Girma, 2020).
Increased resistance is primarily related to FCZ, as this drug has been most frequently used to treat candidiasis. Based on the data from Centers for Disease Control and Prevention (CDC), the prevalence of FCZ resistance is around 2% for C. albicans and 9%–12% for other Candida species. In the case of the echinocandin resistance, it is around 1% for C. albicans and 1%–2% for other Candida species (Cleveland et al., 2012).
Meyerozyma guilliermondii exhibits reduced susceptibility to antimycotics, especially FCZ. Marcos-Zambrano et al. (2017) reported about 10% prevalence of azole-resistant strains and Deorukhkar et al. (2014) observed that 18.2% and 27.3% M. guilliermondii were resistant to FCZ and ICZ, respectively. However, in patients with fungemia, 22.7%–36.4% of M. guilliermondii strains were found to be resistant to FCZ, ICZ, and VCZ (Deorukhkar et al., 2014; Marcos-Zambrano et al., 2017; Tseng et al., 2018). Among the strains we examined, 53.3% were resistant to FCZ before stimulation with MTX but all strains acquired resistance after MTX stimulation. This observation was not reported for ICZ and VCZ. Unfortunately, there were no breakpoint values for this species. Classification of sensitive and resistant isolates is based on epidemiological cutoff values. Therefore, it is difficult to determine the actual incidence of resistance in M. guilliermondii and compare strains of different origins (Ahangarkani et al., 2019; European Society of Clinical Microbiology and Infectious Diseases, 2020; Marcos-Zambrano et al., 2017; Tseng et al., 2018).
According to the published data, the frequency of azole-resistant C. albicans strains is approximately 0.5%–2% (Berkow & Lockhart, 2017; Jensen et al., 2016). The analysis of isolates from hospitals from 29 countries showed that about 0.4% C. albicans isolates were resistant to FCZ and less than 0.1% were resistant to VCZ (Castanheira et al., 2017). However, Deorukhkar et al. (2014) found that the prevalence of resistance to FCZ and ICZ was 33.8% and 38.5%, respectively. In our study, all C. albicans isolates were sensitive to FCZ and 50% were sensitive to VCZ before exposure to MTX. However, all strains became resistant to FCZ and there was no increase in VCZ resistance following MTX stimulation.
The mechanism underlying drug resistance is not fully understood. The most important mechanisms include drug target overexpression, drug target alteration, bypass pathways, efflux pump overexpression, aneuploidy, or loss of heterozygosity (Berkow & Lockhart, 2017). In addition, microorganisms also exhibit the ability to acquire resistance to a drug after contact with another drug, a phenomenon termed as cross-resistance. Cross-resistance has been observed not only in the field of infectious diseases but also during oncological treatment. In a study by van Soest et al. (2015), it was proved that prostate cancer treatment with docetaxel was less effective in patients treated with a novel androgen receptor-targeted agent, enzalutamide. However, this phenomenon was not observed for cabazitaxel, which belongs to the same group of drugs as docetaxel (taxanes) (Nakazawa et al., 2017; van Soest et al., 2015).
In the case of cross-resistance in fungi, cell membrane transporters are very important because they have the ability to actively eject drug molecules used in the therapy of other diseases, for instance cancer, out from the host pathogen cell. It has been established that in C. albicans, the CaMdr1p transporter can actively remove FCZ and MTX from cells (Kohli et al., 2001). MTX is used to treat many skin diseases, particularly severe psoriasis and psoriatic arthritis, as well as pityriasis rubra pilaris, sarcoidosis, skin lymphomas, lichen planus, atopic dermatitis, bullous pemphigoid, dermatomyositis, cutaneous lupus erythematosus, and localized scleroderma (morphea) (Polańska et al., 2016). MTX, one of the earliest cancer chemotherapy agents, continues to be extensively used in the management of acute leukemia and a variety of other solid tumors, including breast cancer, osteosarcoma, primary central nervous system, and head and neck cancers (Abolmaali et al., 2013; Huennekens, 1994). Therefore, MTX is mainly used in patients with an increased risk of invasive candidiasis. The awareness of cross-resistance between FCZ and MTX may be of great significance in the field of medicine where the use of both FCZ and MTX is widespread. To date, no correlation has been described between MTX and other azoles, including VCZ and ICZ. However, our research has demonstrated the possibility of cross-resistance between MTX and FCZ or ICZ in M. guilliermondii and MTX and FCZ or VCZ in C. albicans.
Yang et al. (2019) showed that MTX exhibits antifungal properties and can synergistically act with azoles, including FCZ, ICZ, and VCZ. This phenomenon may be associated with the affinity for MDR1 transporters, which remove MTX from Candida cells instead of azoles (Yang et al., 2019). This confirms the possibility of cross-resistance between these drugs. Our in vitro studies indicate that such a relationship between both drugs may occur in Candida spp. Unfortunately, the available literature lacks information on the relationship between the frequency of FCZ resistance in fungi and the use of MTX in patients.
By comparing the sensitivity of C. glabrata to FCZ in patients with candidiasis before and after FCZ therapy, Jensen et al. (2016) showed a significant increase in the percentage of resistant isolates. The initial 4.8% isolates increased to 29.4% after 7 days. These authors also observed an increase in the number of resistant isolates belonging to C. dubliniensis and C. parapsilosis, while no increase in the prevalence of resistance was noted in C. albicans (Jensen et al., 2016). In our study, the exposure to MTX caused a rapid increase in resistance to FCZ in the studied strains. After exposure, all the tested strains of C. albicans and M. guilliermondii were resistant to FCZ. Studies on the occurrence of cross-resistance between azoles used as fungicides in agriculture and those used as medicines in humans may also reveal interesting information about this phenomenon. The use of tetraconazole in agriculture has been shown to result in the acquisition of FCZ resistance in C. parapsilosis (Rocha et al., 2016).
The gene CaMDR1 is responsible for the cross-resistance between FCZ and MTX in C. albicans strains, whereas other species of Candida genus have homologous genes encoding membrane transporters (for example, CpMDR1 in C. parapsilosis) whose overexpression may also be associated with resistance to FCZ (Arastehfar et al., 2020; Ben-Yaacov et al., 1994; Kohli et al., 2001). In addition, azole resistance in Candida species has been associated with overexpression or mutations of ERG11 or CDR1 (Arastehfar et al., 2020; de Oliveira Santos et al., 2018). Further studies investigating the molecular mechanism of cross-resistance between azoles and MTX showed that these genes and their expression products (proteins) should be taken into consideration. Aside from MDR1, other transport systems such as multidrug resistance-associated proteins (MRP1–5) and breast cancer resistance proteins (BCRP or ABCG2) have also been shown to be involved in the efflux of MTX from cancer cells (Hagner & Joerger, 2010; Wielinga et al., 2005). For this reason, the interaction between azoles and cancer cells should be assessed to detect their azole-induced resistance to MTX chemotherapy.
Appropriate antifungal agent selection and avoiding FCZ may result in an increase in the effectiveness of antifungal empiric therapy and mycosis treatment. In particular, patients with comorbidities who have been previously exposed to antifolates have high probability of cross-resistance to azoles. The prediction of possible drug resistance profiles and selection of a more suitable agent may be crucial for the survival of patients with sepsis of fungal origin (Sipsas & Kontoyiannis, 2012). More targeted therapy would reduce the speed of MDR (Holmes et al., 2016).
Although FCZ continuous to be a first-line treatment for candidiasis, the ESCMID and IDSA guidelines suggest echinocandins for unstable patients and infections caused by C. glabrata, which is an azole-resistant strain (Matthaiou et al., 2015). Further, IDSA guidelines do not recommend FCZ as first-line treatment for patients recently exposed to FCZ (Delaloye & Calandra, 2014; Matthaiou et al., 2015). In the context of the cross-resistance phenomenon observed in our study, recent treatment with MTX can also serve as an indication for the use of echinocandins as the first-line treatment for sepsis. However, other aspects such as drug interactions and contraindications for echinocandins should also be considered (Yeoh et al., 2018). Empiric therapy with azoles for fungemia caused by Candida spp. should be chosen according to the fungal species and the patient’s medical history. This may help achieve a higher possibility of successful treatment.