. Introduction
After decades of anthropogenic water eutrophication, a reverse process, the reoligotrophication, has been observed in water bodies (Gross, 2009; Sand-Jensen et al., 2016). Reoligotrophication is primarily expressed by a reduced phosphorus concentration in water bodies (Van Donk et al., 2008). Frequently, this change is stimulated by reclamation treatments such as hypolimnion water aeration or inactivation of phosphorus (Dondajewska et al., 2019; Kerimoglu et al., 2013).
The improvement in water quality is regularly accompanied by changes in the management of water bodies and the elimination or reduction of pollutants discharged from the catchment basins (Finger et al., 2013; Jaun et al., 2007). Reoligotrophication results in improved water quality (Burkholder & Glibert, 2013), reconstruction of the submerged aquatic vegetation (SAV) (Sand-Jensen et al., 2008), and shifts in phytoplankton structure (Verbeek et al., 2018). Consequently, new ecological niches are created which can be populated by hydrobionts. By improving light availability, the euphotic zone increases, which creates favorable conditions for the development of photoautotrophs at greater depths. The improvement in water quality induces aquatic vegetation recovery or reconstruction. A key factor in this process is the seed and oospore bank deposited in bottom sediments by aquatic angiosperms and charophytes, respectively (Casanova & Brock, 1990). Aquatic vegetation is a key factor in stabilizing the appropriate functioning of water bodies (Blindow et al., 2014; Bornette & Puijalon, 2009). Macrophytes can successfully compete with phytoplankton for nutrients and, therefore, induce a clear-water state, which is an alternative stable state in lakes (Scheffer et al., 1993; Scheffer & van Nes, 2007). The study by Richter and Gross (2013) evidenced charophyte renewal is at the expense of vascular macrophytes in response to the lake reoligotrophication and the associated improvement in light availability. Moreover, this group of macroscopic algae often dominates lower fertility waters and creates more compact communities than most vascular plants, which can strongly influence sedimentation, resuspension, and water column nutrients (Blindow et al., 2014; Schubert et al., 2018). Recent studies demonstrate that even in lakes with increased fertility, charophytes can successfully compete for habitats as they are often competitively stronger vascular plants (Brzozowski et al., 2018; Pełechaty et al., 2014).
Along with improvements in water quality, aquatic vegetation is rebuilding, and often we observe the recovery of precious charophytes in water bodies. In recent years, the sites of the endangered charophyte Lychnothamnus barbatus (Meyen) Leonh. have been recolonized (Pełechaty et al., 2017; Raabe et al., 2012; Sinkevičienė & Urbaitė-Maževič, 2012). This charophyte is threatened with extinction, and red-listed in Poland (Siemińska et al., 2006), Lithuania (Balevičius, 2001), Germany (Schnittler & Ludwig, 1996), the Balkans (Blaženčić et al., 2006), and Australia (Bostock & Holland, 2010). In Poland, L. barbatus is strictly protected. Today, there are only 56 sites of L. barbatus worldwide. Unfortunately, from the nineteenth century, approximately 62 sites of this rare charophyte disappeared (Pełechaty et al., 2017). In the twentieth century, the disappearance of L. barbatus was reported as a result of anthropogenic eutrophication of waters (Sugier et al., 2010 and references therein). Due to the declining number of L. barbatus sites and progressive eutrophication of aquatic environments, this charophyte has been recognized as a sensitive bioindicator of oligo-mesotrophic waters (Karczmarz, 1967; Martín-Closas et al., 2006). Lychnothamnus barbatus is characterized by a disjunctive Euro-Asian-Australian geographical range (Bhatia, 2006; Casanova et al., 2003; Sugier et al., 2010). However, in recent years, this macroalga has expanded its geographical range into North America (Karol et al., 2017). Lychnothamnus barbatus occurs mainly in oligo-mesotrophic to eutrophic lakes in the temperate climatic zone in Central and Eastern Europe and North America (Wisconsin and Minnesota, USA). However, this species is also found in rivers (Casanova et al., 2003), an oxbow lake (Berezovska, personal communication, 2019), excavated peatlands (Sugier, 2009), and paddy fields (Azzella, personal communication, 2016).
One of the lakes containing a recovered population of L. barbatus is Lake Kuźnickie, where the aquatic vegetation disappeared in the early 1980s, due to improper lake management (Pełechaty et al., 2017). In recent years, the vegetation of the studied lake has rebuilt and the water quality has improved. The objectives of this study were: to investigate the long-term trends in aquatic vegetation abundance with the decreasing water fertility and to present new direction changes in the SAV structure, species richness, and biodiversity in Lake Kuźnickie using the perspective of the last 40 years.
. Material and Methods
Study Lake
Lake Kuźnickie (N 52°12′ 56.7″, E 16°05′ 31.0″) is located in the Greater Poland Lakeland, in the Obra River basin. Administratively, the lake belongs to the Greater Poland Voivodship and is situated 80 km south-west of Poznań. The studied water body is a holomictic ribbon lake with a volume of 5,264.9 m3 (Szyper et al., 1980).
The official morphometric data for Lake Kuźnickie are based on measurements collected by the Polish Inland Fisheries Institute in the 1950s, initially the lake area was 75.7 ha, and the maximum depth was 13.2 m (Radziej, 1959). Measurements collected during the study provided evidence of a decrease in the surface area and the maximum depth, which are currently 74.5 ha and 12.4 m, respectively (Figure 1). The average depth of the lake is ca. 7 m. The catchment basin, excluding the lake’s surface area, accounts for 200.3 ha. The catchment area consists mainly of forests with pine dominance in the stand. Arable fields and urbanized areas constitute a small part of the catchment.
Lake Kuźnickie was unsustainably used in the second half of the twentieth century. In 1976, forest areas located in the lake’s catchment were fertilized. Unfortunately, this affected the entire area bordering the northern marshy section of the lake (Szyper et al., 1980). In the years 1975–1977, Lake Kuźnickie was stocked with 2,790 fry individuals of grass carp (Ctenopharyngodon idella Valenciennes) (Szyper et al., 1980). Moreover, 4,880 fry individuals of Cyprinus carpio L. were introduced. In 1975, Lake Kuźnickie was stocked with 17,500 fry individuals of Anguilla anguilla L. The above data prove intensive restocking of the lake with plankton-herbivorous and herbivorous fish, which resulted in significant changes in Lake Kuźnickie, despite intensive harvesting in that period (Szyper et al., 1980). In the 1980s, aquatic vegetation and the L. barbatus population dramatically declined in the lake (Gołdyn, personal communication, 2016; Pełechaty et al., 2017). Although currently a bream lake type, Lake Kuźnickie is intensively stocked with Coregonus albula L. individuals due to the appropriate thermal conditions and water transparency (Polish Angling Association, 2018). Between 2009 and 2018, Lake Kuźnickie was stocked with 9,000,000 individual C. albula hatchlings. Since 2010, the restocking of Esox lucius L. in the lake has been significantly limited (Polish Angling Association, 2018) to reduce the pressure of predatory fish on C. albula.
Water Chemistry and Phytoplankton Analyses
In April and July 2018, basic physical and chemical properties of the Lake Kuźnickie surface waters, including conductivity and pH were analyzed using the deepest part of the lake (Figure 1) with the portable apparatus Elmetron CX-401. In the same location, water transparency was measured using Secchi disc and water samples were collected using a 5-L bathometer (Uwitec Plexiglas Watersampler, Mondsee, Austria) and distributed into 1-L plastic bottles to determine the concentrations of the total phosphorus (TP), Ca2+, and chlorophyll a. Laboratory investigations of the samples followed Standard Methods (Greenberg et al., 1992). Chlorophyll a concentration was determined by the extraction of GF/C-filtered samples in 90% acetone for 24 hr. The results were compared with data from previous studies conducted in 1978 (Gołdyn, 1983; Szyper et al., 1980), 1993 (Voivodship Inspectorate of Environmental Protection [VIEP] in Poznań, 1993), and 2008 (VIEP in Poznań, 2008). In each of the study periods, the research was conducted once in spring (March or April) and summer (July or August) in the pelagic zone free from vegetation. For each analyzed parameter range, the average with standard deviations were calculated. The Trophic State Index (TSI) was calculated according to the method (Carlson, 1977) based on the summer values of TP, chlorophyll a, and transparency for 1993, 2008, and 2018. The TSI was not calculated for the summer of 1978 as no data was available for the total phosphorus concentration.
Vegetation Study
Prior to the vegetation study, detailed bathymetric measurements of Lake Kuźnickie were performed in October 2017, to update the lake’s bathymetric map. For this purpose, the echo sounder (Garmin echoMAP CHIRP 52dv XDCR) was used to create an even network of 3,422 waypoints. The bathymetric map was prepared using QGIS Lyon software.
The vegetation of Lake Kuźnickie was mapped based on field surveys in October 2017 (emerged vegetation) and July 2018 (submerged and floating-leaved vegetation). The map indicates ranges of emergent, submerged, and floating-leaved vegetation and documents the distribution of L. barbatus sites in the lake. The areas occupied by various types of vegetation including L. barbatus were calculated. The results were compared with the study conducted in 1978 (Szyper et al., 1980) and monitoring studies carried out by the VIEP in Poznań (2008). The qualitative and quantitative structure of the SAV was studied in detail from a boat along a depth gradient, using an anchor with a calibrated rope. In each of 323 sites (waypoints), the species composition and abundance were determined using the Braun-Blanquet (1964) method. Macrophytes were sampled outside the reed and floating-leaved plant zones. Since the depth ranges of floating-leaved plants overlapped with the SAV, nymphaeids present in the waypoints were included in the study.
The relative species abundance (RSA) was calculated for each macrophyte species to reflect the quantitative portion of the species in the lake’s submerged vegetation. The RSA is the ratio of the sum of the coverage value of a given species with the sum of the coverage of all identified taxa (Brzozowski et al., 2018). Based on the available hydrobotanical data from previous years, the RSA was also calculated for 1978 (Gołdyn, 1983) and 2008 (VIEP in Poznań, 2008) to investigate the differences in the aquatic vegetation structure during the last 40 years (1978–2018). In the presented research, the RSA was used to compare the macrophyte structure data between the studied periods. In each period, the study covered the entire phytolittoral, however, the studies differed in their methodology. In 1978, the macrophyte structure was estimated using 23 phytosociological plots (Gołdyn, 1983), in 2008 the vegetation was studied using 11 transects according to VIEP environmental monitoring, and this study was performed in 2018 using waypoints. In 1978, 2008, and 2018, macrophyte coverage was determined using the Braun-Blanquet (1964) scale. For the RSA calculation, the Braun-Blanquet (1964) scale (range from r to 5) was transformed into the van der Maarel (1979) scale (range from 1 to 9), as follows: r = 1, + = 2, 1 = 3, 2 = 5, 3 = 7, 4 = 8, 5 = 9. Moreover, for each of the previously presented periods (1978, 2008, 2018), the relative abundance was calculated for macrophyte groups (RAMG), such as vascular plants, charophytes, bryophytes, and filamentous algae. The RAMG is the ratio of the sum of the coverage values of all species belonging to the selected macrophyte group and the sum of the total coverage of all macrophyte taxa. To investigate the biodiversity of the SAV in the studied periods, the Shannon–Wiener index was calculated (Shannon & Weaver, 1949).
Analysis of Phytoplankton Structure
Phytoplankton sampling was carried out in July 2018 at the deepest pelagic site of the studied lake (Figure 1) with a 5-L bathometer (Uwitec Plexiglas Watersampler) from a depth of 0.5 m and stored in a 1-L bottle. The samples were preserved using Lugol’s solution and concentrated to a known volume in the laboratory.
Phytoplankton taxa identification was carried out using a light microscope (at ×200, ×400, and ×1,000 magnification; Leica DMLB). Qualitative and quantitative analyses were conducted using a Fuchs-Rosenthal counting chamber (height: 0.2 mm, area: 0.0625 mm2). The biomass of the phytoplankton was calculated using species biovolume following the criteria and formulae presented by Wetzel and Likens (1991). The results were compared with the monitoring studies from summer 2008 by the VIEP in Poznań. Due to the differences in the sampling methodology for the phytoplankton in 2008 (VIEP in Poznań, 2008) and 2018 (original study), the analysis was limited to a comparison of species richness and the dominant taxa in phytoplankton structure in both periods.
. Results
Decadal Changes in Water Trophy of Lake Kuźnickie
Comparing the older reports with the new results, one may notice that the fertility of Lake Kuźnickie has strongly decreased (Table 1). The TSI values calculated for the three summer seasons of 1993, 2008, and 2018 were as follows: 55.5, 50.3, and 39.8. This result indicates a change in the lake’s trophic status from eutrophic to mesotrophic. The decrease in phosphorus concentration had the greatest impact on the lake’s change in trophic status. Relatively high water transparency was recorded in each of the years analyzed, except in 1978, with the results oscillating from 2.8 m to 4.3 m (Table 1). The chlorophyll a concentration ranged from 1.2 µ g L−1 to 20.9 µ g L−1. The highest level of chlorophyll a occurred in 2008. A minor decrease in electrolytic conductivity and the concentration of Ca2+ were observed along with a slightly increased pH.
Table 1
Decadal changes in the physical and chemical properties of Lake Kuźnickie pelagic waters.
[i] Ranges with mean ± standard deviations from spring and summer sampling campaigns are presented. Source of data: 1978 (Gołdyn; 1983; Szyper et al., 1980); 1993, 2008 – monitoring data of Voivodship Inspectorate of Environment Protection in Poznań (VIEP in Poznań, 1993, 2008); 2018 – this study. Abbreviations: TP – total phosphorus; SD vis. – Secchi disk visibility.
Decadal Changes in the Vegetation Structure
The littoral vegetation (phytolittoral) of Lake Kuźnickie covered 23.1 ha (30.9% of the lake’s area) in the summer season of 2018 (Figure 2). The vegetation occurred up to a depth of 6.9 m. Compared to 2008 (22.2 ha), the lake’s phytolittoral area had increased by 0.9 ha. In 2008, the maximum vegetation depth was 7.5 m. However, in 1978 the vegetation reached a depth of 6 m (Gołdyn, 1983). At that time, the phytolittoral area covered 25.5 ha (33.7% of the lake’s area) (Szyper et al., 1980).
During this study, rush vegetation (8.4 ha) constituted 36.4% of the phytolittoral area. The primary component of the emerged species was Phragmites australis (Cav.) Trin. ex Steud., exceeding 59% of the rush zone. The second most prolific component was Typha angustifolia L., characterized by a 36% contribution. Compared to 2008 (4.9 ha), the area of emerged vegetation increased by 3.5 ha, and P. australis and T. angustifolia were the main components of the rush vegetation (VIEP in Poznań, 2008). Notwithstanding, in the 1978 survey, rush vegetation (10.5 ha) covered 41.2% of the phytolittoral area (Szyper et al., 1980) and was represented mainly by P. australis, Schoenoplectus lacustris (L.) Palla, and T. angustifolia (Szyper et al., 1980).
In 2018, Nymphaea alba L. and Nuphar lutea (L.) Sibth. & Sm. constituted the floating-leaved vegetation zone, covering 1.2 ha (5.0% of the phytolittoral area). There was no significant changes to this vegetation type. Similar to 2008, it covered 1.23 ha (5.5% of the phytolittoral area). In 1978, elodeids and nymphaeids were categorized into a single group (Szyper et al., 1980).
Submerged macrophytes had the greatest share in the lake’s littoral vegetation, namely 58.5%, accounting for more than 13.5 ha of the phytolittoral area. However, in 2008, submerged vegetation (16.1 ha) covered an area 2.6 ha larger than today (VIEP in Poznań, 2008). In 1978, elodeids and nymphaeids occupied 15 ha (Szyper et al., 1980).
An analysis of the changes in the SAV structure of Lake Kuźnickie in the last 40 years evidenced significant remodeling in the group of dominant macrophytes (Figure 3). Currently, only four species of macrophytes are dominant, namely L. barbatus, Nitellopsis obtusa (N. A. Desvaux) J. Groves, Ceratophyllum demersum L., and Myriophyllum spicatum L. In 2008, M. spicatum was the most abundant species in Lake Kuźnickie, while Chara tomentosa L., C. demersum, Rannunculus circinatus Sibth., and Chara contraria A. Braun ex Kützing., were less abundant. In 1978, C. demersum was dominant, whereas L. barbatus, Fontinalis antipyretica Hedw., and Elodea canadensis Michx. were very abundant macrophytes. Although L. barbatus was one of the codominant macrophytes in Lake Kuźnickie in 1978 (Figure 3), in 2008 the species belonged to the accessory group of species (Figure 3), occupying only 0.05% (0.01 ha) of the phytolittoral area (VIEP in Poznań, 2008). Between 2008 and 2018, the L. barbatus population rebuilt and in 2018 it accounted for 7.9% (1.8 ha) of the phytolittoral area (original study). Since 1978, there has been a decline in the species richness from 17 to 13 species recorded in 2018. We also noted a decline in the biodiversity between 1978 and 2018. The Shannon index for the SAV was 0.97 in 1978, 1.03 in 2008, and 0.78 in 2018.
Figure 3
Relative species abundance (RSA, %) of taxa identified in Lake Kuźnickie in the summer seasons of 1978, 2008, and 2018. To calculate RSA the following data were processed: 1978 – Gołdyn (1983); 2008 – monitoring data of Voivodship Inspectorate of Environmental Protection in Poznań (VIEP in Poznań, 2008); 2018 – this original study.
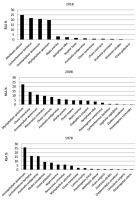
Moreover, in tandem with a decrease in the number of submerged macrophyte species, changes occurred in the relative abundance of macrophyte groups (Figure 4). The relative abundance of vascular plants decreased by 6% from 1978 to 2018. However, in each of the studied periods, vascular plants were the main component of the aquatic vegetation (Figure 4). Over 40 years, the relative abundance of charophytes increased by 21%, these photoautotrophic macroalgae are the main beneficiaries of the changes occurring in Lake Kuźnickie. Currently, the relative abundance of charophytes is similar to vascular plants. Since 1978, the RSA increased, particularly in L. barbatus and N. obtusa (Figure 3). However, simplification of the aquatic vegetation structure was observed, as evidenced by the apparent disproportion between the dominant and accompanying species in 2018 compared to previous years (Figure 3). In the lake, the relative abundance of bryophytes dropped significantly from 16.2% in 1978 to 1.3% in 2018. Filamentous algae were recorded only in 2008 and were characterized with low relative abundance (Figure 4).
Figure 4
The relative abundance of macrophyte groups (%) in Lake Kuźnickie in the summer seasons of 1978, 2008, and 2018. To calculate the abundance of each group of macrophytes the following data were processed: 1978 – Gołdyn (1983); 2008 – monitoring data of Voivodship Inspectorate of Environmental Protection in Poznań (VIEP in Poznań, 2008); 2018 – this original study.
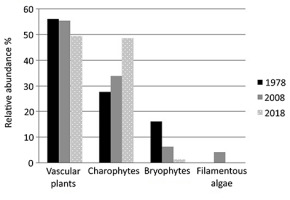
Phytoplankton Assemblage
In total, 39 taxa representing eight taxonomic groups of phytoplankton were identified in the summer of 2018. The most diverse group were chlorophytes (22 taxa, i.e., 56% of the total number of phytoplankton taxa). Other groups were represented by a smaller number of taxa (Table 2).
Table 2
Number of phytoplankton taxa, including taxonomic groups in 2008 and 2018.
Phytoplankton group | 2008 | 2018 |
---|---|---|
Cyanoprokaryota | 4 | 3 |
Chlorophyta | 4 | 22 |
Bacillariophyceae | 6 | 3 |
Chrysophyceae | 1 | 4 |
Haptophyta | 0 | 1 |
Xanthophyceae | 0 | 1 |
Dinophyta | 3 | 3 |
Euglenophyta | 2 | 0 |
Cryptophyta | 1 | 2 |
Total number of taxa | 21 | 39 |
In 2018, the phytoplankton biomass in the epilimnion of Lake Kuźnickie reached 1.6 mg L−1 which is a value typical for mesotrophic waters (Heinonen, 1980). Diatoms [Cyclotella radiosa (Grunow) Lemmermann] and green algae (Oocystis lacustris Chodat) dominated in this period (Table 3). Cyanoprokaryota comprised only 5% of the total biomass and were represented mainly by the chroococcalean picoplanktonic Cyanocatena planctonica Hindák.
Table 3
The biomass of particular taxonomic groups of the phytoplankton assemblage in the summer of 2018.
Phytoplankton group | Biomass mg L−1 |
---|---|
Cyanoprokaryota | 0.091 |
Chlorophyta | 0.471 |
Bacillariophyceae | 0.625 |
Chrysophyceae | 0.067 |
Xanthophyceae | 0.002 |
Haptophyta | 0.047 |
Dinophyta | 0.245 |
Cryptophyta | 0.089 |
Total | 1.637 |
When compared to 2008, the species richness was higher in 2018 (Table 2). The increase in the number of green algae (Chlorophyta) and chrysophyte (Chrysophyceae) taxa is particularly noticeable. In addition, in 2018, previously unlisted species belonging to nannoplanktonic cryptophytes (Cryptophyta), haptophytes (Haptophyta), and yellow-green algae (Xanthophyceae) were observed (Table S1).
The phytoplankton structure indicates that the cyanobacterial community was rebuilt over 10 years. In the summer of 2008, the dominance of filamentous cyanobacteria was evidenced, e.g., Planktothrix agardhii (Gom.) Anagn. et Kom. and Oscillatoria rosea Utermöhl. In 2018, cyanobacteria were represented by only two chroococcalean, picoplanktonic species. The cyanobacterial dominance (2008) was replaced by diatoms and green algae (2018; Table 3).
. Discussion
This research indicates the decrease in phosphorus concentration in the studied lake improved water quality, there was also a significant transformation in the aquatic vegetation. The vegetation rebuilt after the degradation caused by the increasing eutrophication and the introduction of herbivorous grass carp (Pełechaty et al., 2017; Szyper et al., 1980). The vegetation of Lake Kuźnickie has significantly changed its characteristics in the last 40 years (1978–2018) from a lake with the SAV dominated by vascular plants into a lake codominated by vascular plants and charophytes. The lake vegetation research of 39 lakes in Lubusz Land evidenced that charophyte contribution increases with increasing water quality, and this group of macroalgae is a bioindicator of a good ecological status (Pukacz et al., 2013).
However, despite the increase in the contribution of charophytes in the SAV structure, species richness and biodiversity have visibly decreased in Lake Kuźnickie. Interestingly, the highest biodiversity in the SAV was recorded in 2008, when the lake was characterized as eutrophic with a high concentration of phosphorus. However, at that time, very good light conditions prevailed, favoring the development of SAV. This suggests the macrophytes already present in the lake may have contributed to the clear-water state despite the high nutrient levels (Scheffer et al., 1993; Scheffer & van Nes, 2007). This is because well-developed aquatic vegetation can effectively limit the availability of nutrients in the water and thus inhibit the growth of phytoplankton, even under eutrophic conditions (Blindow et al., 2014; Schubert et al., 2018).
Moreover, an analysis of the spatial changes in the phytolittoral area for the period 1978–2018 evidenced significant changes in the areas of aquatic vegetation. The phytolittoral area was mainly rush vegetation which decreased between 1978 and 2018 by over 2 ha. In 2008, the area of rush vegetation had decreased by more than 50% compared to 1978. Degradation of rush vegetation has significant consequences for the functioning of the lake and the number of ecological niches. Additionally, the rush belt is a significant barrier to nutrients transported into the lake by surface runoff from the catchment area. Thus, the emerged vegetation is a natural barrier in the ecotone zone of the lake (Kłosowski, 1992, 2006; Kłosowski & Kłosowski, 2006; Pełechaty & Burchardt, 1998). Unfortunately, like many water reservoirs, fishing and the use of lakes for recreation contributes to the fragmentation leading to a lack of habitat continuity in this zone. It results in easier access and increased inflow of nutrients and pollutants into the lake (Krupska et al., 2011, 2012; Pełechaty, 1997; Pieczyńska, 2008). The results suggest the degradation of the aquatic vegetation in Lake Kuźnickie with the increasing eutrophication of the water caused the high fertility of the lake water in 1993.
The area occupied by elodeids and nymphaeids was comparable in 1978 and 2018 and accounted for approximately 15 ha. However, in 2008 the elodeids and nymphaeids area was higher and covered 17.3 ha. In 2008, the charophytes C. tomentosa, C. contraria, and Chara aspera Willd., in particular, occurred in the shallow littoral (up to 2 m depth; (VIEP in Poznań, 2008)). In the absence of rushes, the charophyte vegetation probably inhabited the free ecological niches. One of the factors positively influencing the recovery of the SAV in Lake Kuźnickie could have been the habitat-forming impact of charophyte pioneer species. This is due to the fact that charophytes play an important functional role in maintaining high water transparency and limit the development of phytoplankton (Blindow et al., 2014; Schubert et al., 2018). In addition, they affect the nutrients cycle (Kufel & Kufel, 2002), reduce the resuspension of bottom sediments (van den Berg et al., 1998), and increase the sedimentation rate of the suspended solids in the water column to the bottom sediments (Nõges et al., 2003). Thus, their presence in lakes enables several feedback mechanisms they are involved in and supported by submerged angiosperms (Blindow et al., 2014). Therefore, charophytes contribute to the light penetration of deeper layers in the water (Schubert et al., 2018).
This study evidenced significant reconstruction of the SAV in the last 40 years. In 1978, two species (L. barbatus and Chara globularis) dominated among five species of charophytes in Lake Kuźnickie, and vascular plants dominated the overall vegetation structure. Both species have a wide ecological amplitude regarding trophic status, the first species occurs from oligotrophic to eutrophic waters (Pełechaty et al., 2017; Sugier et al., 2010), and the second species occurs from mesotrophic to eutrophic waters (Pełechaty & Pukacz, 2008; Urbaniak & Gąbka, 2014). In vascular plants, the eutrophic species C. demersum and invasive E. canadensis dominated. Moreover, aquatic moss F. antipyretica was very abundant. In the next study period in 2008, among vascular plants, M. spicatum, C. demersum, and R. circinatus dominated, while typical shallow species of charophytes, C. tomentosa and C. contraria dominated. In the most recent analysis, the macrophyte structure was simplified. Four species, two charophytes (L. barbatus and N. obtusa), and two vascular plants (C. demersum and M. spicatum) dominated the SAV structure. Most of the charophytes from the genus Chara are shallow-water species whose share in the vegetation structure was negligible. This is because this group of species has to compete for an ecological niche with the more competitive submerge vascular plants and rush vegetation. Moreover, Chara species are losing the competition for habitats against other charophytes including deep water L. barbatus and shallow water N. obtusa, which form dense monospecies communities.
The current disproportion in the Lake Kuźnickie SAV is consistent with the prediction by Moss et al. (2009) for the lakes of Central Europe, as the climate warms, the growth rate of macrophytes will increase while reducing species richness. In the light of these results, the beneficiaries of these changes seem to be charophytes, L. barbatus and N. obtusa, and vascular plants, C. demersum and M. spicatum. Research by other authors have also evidenced SAV recovery through reoligotrophication is possible, but the species richness and the vegetation structure do not fully return to the period before environmental degradation (Sand-Jensen et al., 2008, 2016). Research on vegetation in Lake Simon (Ontario, Canada, North America) evidenced that a decrease in phosphorus concentration in water coincided with a strong remodeling of the aquatic vegetation, with increased abundance of the invasive species of vascular plant, M. spicatum (Ginn, 2011) and later the charophyte, N. obtusa (Ginn et al., 2021) significantly increased. Our research evidenced that these species are highly expansive in the conditions of reoligotrophication in waters of their native geographical range (Europe).
Remarkably, along with the changes of the aquatic vegetation in Lake Kuźnickie, the population of the endangered charophyte, L. barbatus rebuilt. Moreover, along with the improved water quality, the L. barbatus population increased in the lake. To the authors’ knowledge, there are only 12 lakes in the world where the populations of this macroalga occupy higher than 1 ha or more than 5% of the phytolittoral area. There are four lakes in Europe, three in Poland (Gąbka et al., 2010; Kolada, 2009; Pełechaty et al., 2009; and this study) and one in Lithuania (Balevičius, 2001; Sinkevičienė, 2010), and eight in North America (Karol et al., 2017). Contemporary research evidenced that L. barbatus can occur in more fertile waters (Pełechaty et al., 2009, 2017) than previously thought (Karczmarz, 1967), and the factors determining its occurrence in water reservoirs is high water transparency rather than trophic status. L. barbatus creates a compact community in lakes, which are characterized by various sizes and bathymetry, preferring transparent waters from oligotrophic to eutrophic status (Table S2).
Lake Kuźnickie is an example of water quality improvement having taken place without any additional reclamation measures, except the reduction of nutrient discharge into the lake from the direct catchment. Additionally, a change in the fish stocking structure may have contributed to the improvement of the lake’s ecological conditions (Polish Angling Association, 2018). The improvement in the water quality of Lake Kuźnickie is also evidenced by the significant reconstruction of the SAV structure, in which charophytes are currently codominant, as they are bioindicators of a good ecological status. The shift in the phytoplankton structure in Lake Kuźnickie also confirmed an improvement in water quality. The current phytoplankton assemblage in Lake Kuźnickie is characteristic of mesotrophic water bodies (Pełechata et al., 2015, 2020). Currently, the lake has good water quality, indicating mesotrophic conditions, which are optimal for the development of aquatic vegetation, especially charophytes.
. Conclusions
In the period 1978–2018, the aquatic vegetation structure of Lake Kuźnickie underwent a significant reconstruction and the quality of its waters improved with decreasing phosphorous concentrations. Currently, charophytes play a much greater role in the lake than in the previous 40 years, contributing to the maintenance of the lake’s high water quality. Moreover, the endangered species, L. barbatus has recovered in the lake. At the same time, the biodiversity and species richness of the submerged vegetation has decreased. Currently, only four species dominate in the lake, including two charophytes (L. barbatus, N. obtusa) and two vascular plants (C. demersum and M. spicatum). Over 40 years, Lake Kuźnickie has changed from a eutrophic lake dominated by vascular plants into a mesotrophic lake with a codominant contribution by charophytes.