. Introduction
Crossing between individuals of different species or genera provides a way to combine divergent genomes into a single nucleus, thus enabling the creation of novel genetic variation that can be utilized by plant breeders (Visarada & Venkateswaran, 2019). However, various sexual barriers resulting from incompatibility and incongruity prevent interspecific or intergeneric hybridization (van Tuyl & de Jeu, 1997). Depending on the time and place of occurrence, these barriers can be divided into prezygotic (or pre-fertilization) and postzygotic (or post-fertilization) (Dhooghe et al., 2021). Pre-fertilization barriers include lack of stigma receptivity or pollen viability as well as inhibition of pollen tube germination or abnormal growth of pollen tubes in the style and ovary of the recipient. Meanwhile, post-fertilization barriers include abortion of embryos, abnormal growth (over- and under-proliferation) or disintegration of the endosperm, and abnormal growth or sterility of the hybrids (Tonosaki et al., 2016; Zulkarnain et al., 2015).
To bypass the prezygotic barriers, a range of techniques, such as the use of mentor pollen or pollination of buds as well as more sophisticated in vitro direct pollination of pistils with attached perianths, in vitro pollination of ovaries placed on artificial media with partly or entirely removed walls, and in vitro pollination of isolated ovules, have been developed. In addition, in vitro culture of ovaries, ovules, and embryos isolated at the early developmental stages (embryo rescue) has been established to overcome post-zygotic barriers, such as endosperm failure or embryo abortion. In many breeding programs, an integrated method using in vitro pollination and fertilization, followed by embryo rescue, has been applied to facilitate wide hybridization and enable the introgression of genes across species barriers (van Tuyl & de Jeu, 1997; Zenkteler & Zenkteler, 2013).
Another technological aid to conventional breeding, developed to overcome the failure of wide hybridization, is somatic hybridization. This method was introduced 50 years ago, with the first successful somatic fusion between Nicotiana glauca Graham and Nicotiana langsdorffii Weinm. (Carlson et al., 1972). The merger of enzymatically isolated somatic cell protoplasts into a heterokaryon, followed by plant regeneration, circumvents species incompatibility and enables the transfer of valuable traits, particularly polygenic ones or those conferred by organellar genomes, from the donor to recipient species without the knowledge of molecular details of the corresponding genes (Arcioni & Pupilli, 2003; Davey, 2017). Therefore, the greatest expectations of plant breeders are associated with the transfer of desirable qualities, such as resistance to bacterial, fungal, and viral diseases, pests, herbicides, and other stress factors, from wild species into their cultivated forms (Tapingkae et al., 2012). Another approach focuses on creating novel combinations of nuclear and/or cytoplasmic genomes and broadening the genetic base of the species. In the 1980s, somatic fusion techniques garnered enormous global interest, and various intraspecific, interspecific, and intergeneric somatic hybrids of many plant species were obtained in research laboratories worldwide.
In Poland, as in the rest of the world, the abovementioned tissue culture methods have been widely utilized in fundamental and specific applications for the production of distant hybrids. The aim of the present review is to summarize the history of exploitation of these techniques and present examples of the most advanced achievements of Polish research groups working on wide hybridization, among others, for the production of intergeneric hybrids between willows (Salix spp.) and poplars (Populus spp.); resynthesis and broadening of the genetic variability of oilseed rape (Brassica napus L.); interspecific hybridization of Allium; and somatic hybridization of potato (Solanum tuberosum L.), tomato (Solanum lycopersicum L.), and gentians. The key findings of the related studies and their prospects are presented.
. Pioneer Research of Professor Maciej Zenkteler on Overcoming Crossing Barriers
In Poland, research on the wide hybridization of plant species began in the mid-1960s (Zenkteler & Zenkteler, 2013). The first attempts to eliminate pre-fertilization barriers using in vitro cross-pollination were undertaken within the family Caryophyllaceae by Professor Maciej Zenkteler of the Department of General Botany, Adam Mickiewicz University in Poznań. In these experiments, the ovules of Silene latifolia Poir. were aseptically isolated from sterilized pistils by removing the ovary wall; inoculated with the placenta on White’s medium; and pollinated with pollen grains of Silene dioica (L.) Clairv., Silene schafta J. G. Gmel. ex Hohen, Silene tatarica (L.) Pers., and Dianthus carthusianorum L. (Zenkteler, 1967). Fertilization was successful, and hybrid embryos developed in all species combinations. In crosses between Silene latifolia and Silene dioica as well as between Silene latifolia and Silene schafta, healthy seedlings were obtained after the dissection of embryos and their transfer onto fresh medium. Following acclimatization, the plantlets continued to grow and set flowers.
Further experiments conducted by Professor Zenkteler included interfamilial crosses, more precisely between species of the families Caryophyllaceae (Silene latifolia) and Solanaceae (Datura stramonium L., Nicotiana tabacum L., and Petunia hybrida Vilm.), as well as intersubfamilial crosses, such as between Nicotiana tabacum and Petunia hybrida or Nicotiana tabacum and Hyoscyamus niger L. (Zenkteler, 1970; Zenkteler & Melchers, 1978). Although pollen grains germinated in all cases and pollen tubes often reached the embryo sacs, embryo development was observed only in the Nicotiana tabacum and Hyoscyamus niger crosses. Following rescue on a medium supplemented with plant growth regulators, embryos reached the pre-heart stage; soon after, however, their cells started to proliferate, resulting in callus formation (Zenkteler & Melchers, 1978). Interspecific hybridization between Nicotiana tabacum and Nicotiana knightiana Goodsp. was more successful, resulting in the regeneration of fully formed flowering plants containing 2n = 36 chromosomes (Ślusarkiewicz-Jarzina & Zenkteler, 1983). Earlier, such hybrids were produced via protoplast fusion, although they were cytogenetically unstable.
Further wide hybridization experiments employing in vitro ovule pollination and embryo rescue involved species from different genera of the family Brassicaceae, including Alliaria petiolata (M. Bieb.) Cavara & Grande, Arabis caucasica Willd., Barbarea vulgaris R. Br., Brassica cretica Lam., Brassica napus L., Brassica oleracea L., Brassica rapa L., Diplotaxis tenuifolia (L.) DC., Erysimum crepidifolium Rchb., Erysimum pulchellum (Willd.) J. Gay, Erysimum virgatum Roth, Erysimum witmannii Zaw., Hesperis matronalis L., Moricandia arvensis (L.) DC., Sinapis alba L., Sinapis arvensis L., and Sisymbrium loeselli L. However, because of intergeneric crosses, the obtained immature hybrid embryos were at different stages of development (Zenkteler, 1990, 1992). Viable hybrid plants developed only from hybrid embryos of Brassica napus × Brassica rapa, Brassica rapa × Brassica napus, Brassica oleracea × Brassica cretica, and Brassica oleracea × Moricandia arvensis (Zenkteler, 1992).
Further, limited success was achieved with intergeneric and interspecific crosses within the family Fabaceae. In these experiments, single ovules cultured directly on a medium were pollinated in vitro. A few globular embryos were obtained from only one (Trifolium rubens L. × Cytisus albus Hacq.) of the five species combination tested (Zenkteler, 1995). In addition, studies aimed at overcoming pre- and post-zygotic barriers in reciprocal crosses between the important legume Vicia faba L. and its wild relative, Vicia narbonensis L., which is diseases resistant and drought tolerant, achieved little success, as the isolated ovaries and pistils were susceptible to sterilization agents (Zenkteler et al., 1998).
Of note, Professor Zenkteler also investigated the possibility of in vitro pollination of angiosperm ovules (Nicotiana tabacum, Silene latifolia, and Allium moly L.) with the pollen of the gymnosperms Ephedra distachya L. and Pinus wallichiana A. B. Jacks. Although pollen grains of either species germinated soon after their placement on ovules and some histological symptoms indicated the entrance of pollen tubes into the embryo sacs, the fusion of male and female gametes was not observed (Zenkteler, 2000).
. Use of In Vitro Fertilization and Embryo Rescue to Obtain Distant Hybrids in Poland
Studies employing in vitro culture to overcome crossing barriers, initiated by Professor Zenkteler, were continued by his associates and collaborators, and in vitro pollination and embryo rescue methods were extended to many other Polish universities and institutes. These methods were soon harnessed in both fundamental and applied research to create new plant traits and improve various woody and herbaceous crop species.
Intergeneric Hybridization of Willows and Poplars
The genus Salix contains species of high value as biomass crops. The fast-growing and short-rotation tree species Salix viminalis L. has attracted much research attention due to its productivity and economic potential. Meanwhile, the genus Populus, represented by hardwood trees with large biomass increments, appears promising from the perspective of sustainable energy economy. Thus, the introgression of poplar genes into the genome of Salix viminalis has been the objective of research on their intergeneric hybridization (Bagniewska-Zadworna et al., 2011; Zenkteler et al., 2005).
In the initial experiment, stigmas and placentas of Salix viminalis were pollinated in vitro with the pollen grains of Populus tremula L., Populus tomentosa Carrière, and Populus lasiocarpa Oliv. but sporadic globular embryos were obtained only after placental pollination (Zenkteler et al., 2003). Subsequent attempts to pollinate isolated and partially sterilized catkins of Salix viminalis with pollen of Populus tremula, Populus tomentosa, Populus lasiocarpa, and Populus alba L. yielded far better results, with 141 embryos, from which 92 plantlets were developed (Zenkteler et al., 2005). The application of embryo rescue as a complement to in vitro pollination of catkins maximized the efficiency of intergeneric hybridization between Salix and Populus species and allowed for obtaining hybrid progeny between Salix viminalis and Populus alba, Salix viminalis and Populus tremula, and Salix viminalis and Populus violascens Dode. The success of hybridization was confirmed using scanning electron microscopy, flow cytometry, and molecular markers. Three Salix viminalis × Populus alba, 52 Salix viminalis × Populus tremula, and 6 Salix viminalis × Populus violascens plants were transferred to the Botanical Garden in Poznań for vegetative propagation (Bagniewska-Zadworna et al., 2011).
Interspecific Hybridization for De Novo Synthesis of Oilseed Rape (Brassica napus L.)
Brassica napus L. (oilseed rape or rapeseed) is the third largest oilseed crop in the world, following palm and soybean. The production of this crucial species for food and feed has rapidly increased over the past decades not only because of market growth in the cultivation area but also because of the availability of high-yielding, open-pollinated, and hybrid cultivars.
Oilseed rape (AACC genome) is an allopolyploid plant composed of two genomes, Brassica rapa (AA genome) and Brassica oleracea (CC genome). Presumably, Brassica napus arose as a result of multiple spontaneous and independent hybridizations between Brassica rapa and Brassica oleracea off the coast of Northern Europe, where both diploid parental species are widespread (Sosnowska et al., 2010). Another speculation is that oilseed rape originated in the Mediterranean region or in Western or Northern Europe. Possibly, Brassica napus originated elsewhere from crosses between different genotypes of Brassica oleracea and Brassica rapa (Szała et al., 2019).
A short history of domestication of Brassica napus and breeding strategies, such as selection for low erucic acid and glucosinolate content of seeds (double-low quality; synonym: 00 quality) using germplasm of the same quality within a repeated breeding cycle, and development of spring and winter crop types, can be attributed to the lack of adequate genetic diversity in this crop. Intensive breeding has significantly reduced the genetic and phenotypic diversity of this species. The genetic bottleneck of modern oilseed rape material restricts and reduces the possibility of further improving seed quality and yield via breeding of new hybrid varieties through heterosis, the effect of which is related to the genetic distinctiveness of parental lines. However, new possibilities have been created by de novo synthesis of Brassica napus (2n = 4x = 38) through interspecific hybridization between the highly polymorphic parental species Brassica rapa (2n = 2x = 20) and Brassica oleracea (2n = 2x = 18).
Research on the resynthesis of winter oilseed rape from different parental subspecies and incorporation of new genotypes into practical breeding has been conducted at the Plant Breeding and Acclimatization Institute – National Research Institute (IHAR-PIB) in Poznań (Cegielska-Taras et al., 2015). For this purpose, two in vitro culture methods, namely in vitro pollination and embryo rescue, were optimized. In the approved protocol, pollen grains were applied directly onto the surface of exposed ovules placed on Murashige and Skoog (MS) medium (Sosnowska & Cegielska-Taras, 2014) and well-developed ovules containing embryos at the early stage of development were isolated from the siliques and placed on MS medium supplemented with kinetin, 1-naphthaleneacetic acid (NAA), and coconut water. Next, embryos from the enlarged ovaries were isolated and placed on the same medium. After greening, the embryos were transferred to MS medium supplemented with 100 µM kinetin to promote shoot development. Next, the shoots were excised and rooted using 49.2 µM indole-3-butyric acid (IBA) (Cegielska-Taras et al., 2002). Plantlets were then transferred to soil. The resulting hybrids contained 19 chromosomes. When the number of chromosomes was doubled, a resynthesized (RS) oilseed rape with 38 chromosomes was obtained (Figure 1).
Figure 1
Resynthesis of Brassica napus L. using Chinese cabbage (Brassica rapa ssp. pekinensis) and curly kale (Brassica oleracea ssp. acephala var. sabellica). Histograms in the scheme show nuclear DNA content, with x-axis showing relative DNA content and y-axis showing the number of nuclei. All photographs are retrieved from the archives of the Department of Oilseed Crops, Plant Breeding and Acclimatization Institute – National Research Institute, Poznań.
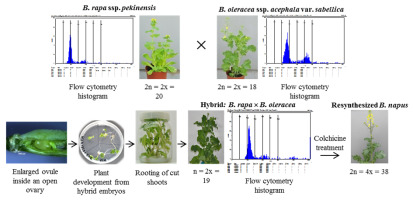
The application of the aforementioned protocol allowed for obtaining RS plants that were genetically and phenotypically different from natural Brassica napus, that is, from all genotypes of Brassica napus that were derived from oilseed rape resulting from spontaneous and independent hybridization between Brassica rapa and Brassica oleracea. Figure 2 illustrates the large phenotypic diversity in the leaves and flowers of RS plants obtained through interspecific hybridization, depending on the parental components. The use of in vitro culture techniques has also accelerated the reconstruction of oilseed rape from different parental subspecies.
Figure 2
Morphological variation in the leaves (A) and flowers (B) of different resynthesized (RS) oilseed rape lines: RS-2, RS-3, RS-26 [Brassica rapa ssp. chinensis var. chinensis (Pak Choy) × Brassica oleracea ssp. acephala var. sabellica ‘Halbhoher Grüner’]; RS-33, RS-43 [Brassica rapa ssp. oleifera ‘Skye’ (spring type) × Brassica oleracea ssp. acephala var. sabellica ‘Halbhoher Grüner’]; RS-34, RS-37, RS-39 [Brassica rapa ssp. oleifera ‘Kova’ (spring type) × Brassica oleracea ssp. acephala var. sabellica ‘Halbhoher Grüner’]; RS-42 [Brassica oleracea ssp. acephala var. sabellica ‘Halbhoher Grüner’ × Brassica rapa ssp. oleifera ‘Skye’ (spring type); RS-44, RS-48 [Brassica oleracea ssp. acephala var. sabellica ‘Vitessa’ × Brassica rapa ssp. oleifera ‘Ludowy’ (winter type)]; RS-50, RS-51 [Brassica oleracea ssp. acephala var. sabellica ‘Vitessa’ × Brassica rapa ssp. oleifera ‘Kova’ (spring type)]; RS-49, RS-53, RS-54 [Brassica oleracea ssp. acephala var. sabellica ‘Vitessa’ × Brassica rapa ssp. oleifera ‘Skye’ (spring type)]; RS-61, RS-63, RS-66 [Brassica oleracea ssp. acephala var. sabellica ‘Vitessa’ × Brassica rapa ssp. oleifera ‘Premium’ (winter type)]; RS-67 [Brassica rapa ssp. oleifera ‘Salut’ (winter type) × Brassica oleracea ssp. acephala var. sabellica ‘Kapral’]; RS-68, RS-69, RS-70 [Brassica rapa ssp. pekinensis ‘Kilakin’ × Brassica oleracea ssp. acephala var. sabellica ‘Vitessa’]; RS-71, RS-72, RS-73, RS-74 [Brassica oleracea ssp. fruticosa var. gemmifera ‘Crispus’ × Brassica rapa ssp. pekinensis ‘Kilakin’]; RS-75, RS-76, RS-77 [Brassica rapa ssp. oleifera, breeding line CR2877 (winter type) × Brassica oleracea ssp. acephala var. sabellica ‘Vitessa’]; RS-78, RS-79, RS-80 [Brassica oleracea ssp. fruticosa var. gemmifera ‘Crispus’ × Brassica rapa ssp. oleifera, breeding line CR2877 (winter type)]. All photographs are retrieved from the archives of the Department of Oilseed Crops, Plant Breeding and Acclimatization Institute – National Research Institute, Poznań.
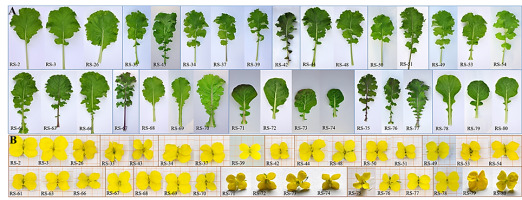
The application of embryo culture techniques facilitated interspecific hybrids between all C and A genomes of Brassica. However, cytogenetic observations have revealed large genomic instability in some RS lines of oilseed rape. RS hybrids from ancestral species could introduce new diversity to Brassica napus, although the hybrids were often chromosomally unstable and presented morphological abnormalities. Using fluorescence in situ hybridization (FISH), chromosome constitutions in a range of new oilseed rape genotypes resynthesized from various parental subspecies were tested. Majority of the allopolyploids were euploid, with the expected chromosome numbers and constitution; however, some deviations were also identified (Sosnowska et al., 2020).
Introgression of the RS genotypes of Brassica napus in breeding programs can be used to enhance the variation in the oilseed rape gene pool. In general, the RS lines are characterized by a high content of erucic acid in the oil and glucosinolates in the meal. Moreover, the RS lines of oilseed rape exhibit poorer performance in terms of many agronomic traits and lower seed yield than the current breeding materials. In addition, RS lines are characterized by low fertility and unfavorable utility values (Sosnowska et al., 2020). Therefore, RS Brassica napus was crossed with double-low-quality oilseed rape. From the resulting hybrid, a population of doubled haploid (DH) semi-resynthesized (semi-RS; containing approximately 50% of the RS genome) lines could be obtained through androgenesis; from these, DH plants with improved seed yield and quality as well as significant genetic distance from the natural cultivars could be selected.
Next, RS plants were crossed with double-low winter oilseed rape lines harboring the Rfo gene for cytoplasmic male sterility (CMS-ogura). Populations of a large number of androgenic semi-RS plants were developed from F1 hybrids using isolated microspore in vitro culture methods (Cegielska-Taras et al., 2002; Szała et al., 2020). The seeds of all obtained semi-RS DH lines with the Rfo gene were analyzed biochemically in terms of the 00 quality. Among the populations of semi-RS DH lines with the Rfo gene, lines with zero erucic acid content and low levels of glucosinolates were selected (Szała et al., 2016). Genetic similarity between these genotypes and natural Brassica napus has been assessed using different molecular markers (Sosnowska et al., 2017; Szała et al., 2016). The new semi-RS lines of 00 quality were genetically distinct from natural oilseed rape but closer to the RS Brassica napus lines (Sosnowska et al., 2017; Szała et al., 2019).
Preliminary field experiments with a double-low semi-RS restorer line as a component of the CMS-ogura line revealed a potentially high degree of heterosis compared with that in the natural component (Szała et al., 2016). The effectiveness of this strategy was confirmed based on the yield of the experimental hybrids evaluated in field experiments. Introduction of 50% of the RS oilseed rape (semi-RS) genotype into a natural restorer lines produced a marked heterosis effect on seed yield, ranging from 4.56% to 90.17%, with respect to the better parent (Szała et al., 2019).
In 2021, four new generations of hybrids, created with the involvement of the DH semi-RS line with the Rfo gene and in cooperation with the Plant Breeding Strzelce Company in Division Borowo, were tested for seed yield in field experiments at four locations. Among the 29 tested hybrids, three new-generation hybrids yielded the best reference hybrid cultivars (unpublished data).
Broadening the Variability of Oilseed Rape Through Interspecific Hybridization With Immature Embryo Culture
Research on interspecific hybridization of oilseed rape has been conducted in the Department of Genetics and Plant Breeding of the Poznań University of Life Sciences (PULS). The work started with the first reciprocal crosses between Brassica rapa and Brassica oleracea supported by the in vitro culture of immature embryos (Wojciechowski, 1985). Subsequent studies aimed at transferring valuable traits into the genome of oilseed rape, with special attention paid to the introduction of resistance to clubroot (Plasmodiophora brassicae). To achieve this goal, several reciprocal crosses between Brassica napus and Brassica rapa ssp. chinensis were performed. The results revealed unilateral crossability between hybridized species, manifesting in a greater pollen germination index and better embryo development when Brassica rapa ssp. chinensis was used as the female parent and Brassica napus as the male parent (Niemann et al., 2015). True F1 hybrids between these species were intermediate in terms of morphological traits compared with the parental genotypes; however, in their pollen mother cells (PMCs), various abnormalities, such as univalent, lagging chromosomes and disorders in synchronization, were observed during meiosis (Niemann et al., 2019).
Next, series of interspecific crosses with the application of immature embryo culture between Brassica napus MS8 line (male sterile line, selected from the F8 generation of RS oilseed rape Brassica rapa ssp. chinensis × Brassica oleracea var. gemmifera) and different Brassica species, including Brassica rapa ssp. trilocularis, Brassica rapa ssp. pekinensis, Brassica carinata A. Braun, Brassica juncea (L.) Czern., and Brassica napus, were developed. The hybrid generations obtained from these crosses were tested for various quality traits. First, seeds from 96 hybrid lines obtained through the crosses of MS8 with Brassica rapa ssp. trilocularis, Brassica carinata, and Brassica juncea were assessed for fiber and glucosinolate, sinapine, and tocopherol content. All tested hybrid lines showed greatly increased variability relative to the parental species; moreover, lines with reduced glucosinolate content were selected (Niemann et al., 2012). Second, the fatty acid composition of F1–F3 generations of hybrids was analyzed, which allowed for the selection of a single hybrid line (H5) for further breeding programs (Niemann, Bocianowski, et al., 2020). Third, the F4 generation of hybrids from crosses between MS8 and Brassica rapa ssp. pekinensis was tested for clubroot resistance, and the most resistant 10 lines were evaluated for seed quality. Characterization of plant material in these experiments included cytological techniques, such as FISH using two types of probes, namely ribosomal DNA for the detection of ribosomal genes (rDNA-FISH) and artificial bacterial chromosome (BAC) clone BoB014O06 from a Brassica oleracea BAC library for the detection of the C genome (BAC-FISH). Two of the tested hybrid lines showed marked resistance to clubroot, with appropriate plant morphology, good seed quality traits, and a stable chromosome number and arrangement (Niemann et al., 2017).
The next generations (F5–F7) of hybrids between MS8 and Brassica rapa ssp. trilocularis, Brassica rapa ssp. pekinensis, Brassica carinata, and Brassica juncea, together with two lines of Brassica napus (MS8 and ‘Californium’) were tested for fiber, protein, oil, and glucosinolate levels in seeds at three different locations. All quality parameters were affected by either genotype or the environment. Specifically, the genotype had the most significant impact on glucosinolate content, whereas the fiber, protein, and oil levels were affected mostly by the field location. Finally, individual lines with appropriate combinations of desirable traits were identified (Niemann et al., 2018).
Another series of interspecific hybrids was generated through in vitro culture of isolated embryos from the crosses of six cultivars and one line of Brassica napus with Brassica rapa ssp. pekinensis, Brassica rapa ssp. chinensis, Brassica rapa ‘Pak Choi’, Brassica oleracea var. alboglabra, Brassica carinata, Brassica fruticulosa Cirillo, Brassica tournefortii Gouan., and Sinapis alba. The obtained hybrids were tested for resistance to cabbage root fly (Delia radicum) and cabbage aphid (Brevicoryne brassicae) and a hybrid combination (Brassica napus ‘Jet Neuf’ × Brassica carinata PI 649096), which exhibited marked resistance across all years tested, was selected (Niemann, Szwarc, et al., 2020).
Interspecific Crosses in Allium
Many important traits (disease and pest resistance and CMS) have been successfully transferred to Allium crops through sexual hybridization (Kik, 2002; van Heusden et al., 2000). At the Department of Plant Biology and Biotechnology (formerly Department of Genetics Plant Breeding and Seed Science) of the University of Agriculture in Krakow (URK), a series of experiments on interspecific crosses have been performed to introgress desirable traits from Allium fistulosum L., Allium roylei Stearn., and Allium galanthum Kar. & Kir. into Allium cepa L. (Adamus, 2004; Chuda & Adamus, 2005, 2009; Chuda et al., 2006, 2012). Introgression was performed through manual pollination. The plants were crossed in the following pairs: Allium cepa × Allium roylei, Allium cepa × Allium fistulosum, and Allium galanthum × Allium cepa (Figure 3A–E). Soon after successful pollination, the ovaries of the mother plants started enlarging (Figure 3F). Hybrid embryos were obtained via embryo rescue via the excision of ovules from the ovaries approximately 10–14 days after pollination. The embryos started emerging from the ovules approximately 3–4 weeks after the inoculation of the ovules on solid media (Figure 3G). The embryos were then regenerated and acclimatized to ex vitro conditions (Figure 3H,I). All regenerants were subjected to molecular analysis to verify their hybrid status. Then, F1 hybrids were evaluated in terms of plant morphology, pollen viability, microsporogenesis, and female gametophyte development (Chuda et al., 2015; Kiełkowska, 2012; Kiełkowska & Adamus, 2010).
Hybridization of onion with Allium fistulosum was undertaken to transfer disease and pest resistance into cultivated onions. Allium fistulosum harbors resistance genes against onion smut (Urocystis cepulae), pinkroot (Phoma terrestris), onion leaf blight (Botrytis squamosa), anthracnose (Colleotrichum gloeosporioides), onion yellow dwarf virus (OYDV), onion fly (Delia antiqua), and thrips (Thrips tabaci) (Khrustaleva & Kik, 2000; Kik, 2002). Previously, the Allium cepa × Allium fistulosum hybrids were proven very less effective, because only a few seeds were obtained from several hundred hand pollinations (Khrustaleva & Kik, 1998, 2000; van der Valk et al., 1991). Thus, the aim of the experiments was to increase the number of plants obtained after crossing the two species. To achieve this goal, embryo rescue was applied (Adamus, 2004; Adamus & Samek, 2007). Through in vitro experiments, nearly 100 F1 (Allium cepa × Allium fistulosum) hybrids were obtained. Unfortunately, because of hybrid sterility, difficulties in backcrossing F1 plants to bulb onion occur (Chuda & Adamus, 2009).
Another species, Allium roylei, is considered a source of resistance to onion leaf blight, downy mildew (Peronospora destructor), and anthracnose (Khrustaleva & Kik, 2000). The first sexual hybridization between Allium cepa and Allium roylei was conducted by Van der Meer and de Vries (1990); as a result, only a single interspecific hybrid plant was obtained. To increase the number of plants obtained after crossing Allium cepa and Allium roylei, Chuda and Adamus (2005) used in vitro culture. After pollination of Allium cepa with the pollen of Allium roylei, ovules were isolated in vitro and approximately 170 plants were obtained. These plants were examined for several morphological characteristics at both vegetative and generative stages (Figure 3J). Nuclear DNA analysis of the obtained progeny revealed that 98% of the regenerants were interspecific hybrids. Genomic in situ hybridization (GISH) revealed that hybrid plants possessed eight chromosomes identified as Allium cepa and eight chromosomes identified as Allium roylei (Chuda & Adamus, 2005, 2007, 2012; Chuda et al., 2012, 2015).
Hybrid onion seed production is based on CMS. Two CMS systems (S and T) have been characterized and commercially used to produce hybrid seeds. Majority of the hybrid onion cultivars are produced using the S cytoplasm (Havey, 1997). Diversification of male-sterile cytoplasm in onions is of great importance. Crosses between onion and Allium galanthum were aimed at the induction of a new type of male sterility. In particular, male sterile onion lines possessing cytoplasm from Allium galanthum have been produced. The interspecific F1 (Allium galanthum × Allium cepa) hybrids were obtained via embryo rescue and acclimatized to ex vitro conditions (Figure 3K). Morphological traits and pollen fertility of the hybrids and their parental plants were evaluated. The tested F1 hybrids were similar to the maternal form in terms of leaf length, while the number of bulbil leaves and stem diameter were similar to the paternal form. Moreover, pollen viability test with Alexander’s dye (Alexander, 1969) showed that the F1 hybrid plants exhibited complete or partial male sterility (Figure 3L). Analyses of chloroplast DNA proved that all F1 progenies examined possessed Allium galanthum cytoplasm (Kiełkowska & Adamus, 2010).
All materials obtained from the abovementioned crosses (i.e., Allium cepa × Allium fistulosum, Allium cepa × Allium roylei, and Allium galanthum × Allium cepa), including the F1 hybrids as well as backcross progenies, were transferred to Polish companies (Plantico, KHiNO POLAN, and Spójnia Nochowo) for further breeding.
Figure 3
Obtaining interspecific F1 hybrids in Allium through in vitro culture. Allium cepa: An emasculated umbel (A) and an umbel serving as the pollen donor (E). Pollen donors: Allium roylei (B), Allium fistulosum (C). Emasculated umbel of Allium galanthum (D). Enlarged ovaries at 10 days after pollination of Allium cepa with the pollen of Allium roylei (F). Embryo rescue: Excised ovules and embryo development after crossing of Allium cepa and Allium roylei (G). Regenerated F1 (Allium cepa × Allium roylei) plantlet (H). F1 (Allium galanthum × Allium cepa) regenerant acclimatized to ex vitro conditions (I). Flowering of F1 (Allium cepa × Allium roylei) hybrids (J). F1 hybrids (Allium galanthum × Allium cepa) planted into pots (K). Pollen viability of F1 (Allium galanthum × Allium cepa) hybrid tested with Alexander’s dye: Partially viable (L) pollen; magenta-green pollens are viable, transparent-green pollens are sterile. Scale bar: 20 µm. All photographs are retrieved from the archives of the Department of Plant Biology and Biotechnology, Faculty of Biotechnology and Horticulture, University of Agriculture in Krakow.
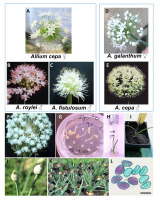
. Somatic Hybridization
Although extensive studies on the isolation of plant protoplasts started in Poland at the end of the 1960s following the initial work of Professor Edward Pojnar of the University of Agriculture in Krakow (Pindel et al., 2012), Professor Zenkteler was the first Polish researcher who attempted protoplast fusion (Zenkteler & Melchers, 1978). Although the trials aimed at obtaining somatic hybrids between Nicotiana tabacum and Petunia hybrida failed, further research conducted in Polish institutes, largely on cultivated potato (Solanum tuberosum) and its wild relatives, witnessed great success.
Somatic Fusion of Potato
Somatic hybridization has been frequently used to overcome crossing barriers between Solanum tuberosum and wild and primitively cultivated potato species. In Poland, researchers at IHAR-PIB in Młochów and Radzików as well as at the Institute of Biochemistry and Biophysics of the Polish Academy of Sciences (IBB PAS) in Warsaw have conducted studies on potato somatic hybrids, primarily to transfer resistance to Phytophthora infestans, potato viruses, nematodes, Colorado beetles, and bacterial diseases and tolerance of abiotic stresses, such as drought and salinity (Orczyk et al., 2003; Tiwari et al., 2018). The most frequent trait transferred via somatic fusion is resistance to Phytophthora infestans, one of the most destructive pathogens of cultivated potatoes globally, causing potato late blight disease (Fry, 2008). In Poland, resistance to Phytophthora infestans was transferred into cultivated potatoes from the wild potato species Solanum nigrum L. (Szczerbakowa, Bołtowicz, & Wielgat, 2003; Zimnoch-Guzowska et al., 2003), Solanum villosum Mill. (Tarwacka et al., 2013), and two of the most frequently used species, namely Solanum pinnatisectum Dunal (Szczerbakowa et al., 2005) and Solanum bulbocastanum Dunal (Bołtowicz et al., 2005; Szczerbakowa, Maciejewska, et al., 2003). Another promising source of resistance to late blight is Solanum ×michoacanum (Bitter.) Rydb., a diploid wild potato species originating from Mexico; it is a spontaneous hybrid of Solanum bulbocastanum and Solanum pinnatisectum (Hawkes, 1990) and has never been used as a source of resistance. At the Młochów Research Center of IHAR-PIB, clones of Solanum ×michoacanum resistant to Phytophthora infestans in leaflets and tubers were selected (Jakuczun & Wasilewicz-Flis, 2004). In collaboration with IBB PAS and the Julius Kühn Institute (JKI) in Quedlinburg, Germany, Solanum ×michoacanum (+) Solanum tuberosum somatic hybrids were obtained via polyethylene glycol (PEG)-mediated hybridization (Szczerbakowa et al., 2010) and electrofusion (Smyda et al., 2013). Among the obtained fusion products, tuber-bearing and fertile hybrids that were resistant to late blight were selected.
During somatic hybridization, several procedures inhibit the growth of homokaryons in at least one of the parental populations. Heterokaryons can be selected through the addition of fluorescein diacetate (FDA) to the enzyme mixture; protoplasts with double fluorescence (red from chlorophyll and yellow/green from FDA) are recognized as heterokaryons and selected for further regeneration (Przetakiewicz et al., 2007). Smyda et al. (2013) have described the intentional selection of homokaryons (4x Solanum ×michoacanum). The increased degree of ploidy in Solanum ×michoacanum allowed for overcoming the crossing barriers with the cultivated potato.
Although most of the obtained potato somatic hybrids require backcrossing to remove undesirable traits of the wild parent, only a few studies have established BC1, BC2, and BC3 generations within potato somatic hybrids (e.g., Chen et al., 2013; Helgeson et al., 1998; Rakosy-Tican et al., 2015; Thieme et al., 2004, 2008). At IHAR-PIB, Młochów BC1 and F1 generations were obtained from crosses in both directions of Solanum ×michoacanum (+) Solanum tuberosum somatic hybrids resistant to Phytophthora infestans and 4x Solanum ×michoacanum autofusants with the selected potato cultivars (Smyda-Dajmund et al., 2017). After 2 years of Phytophthora infestans resistance screening, 25 BC1 progeny originating from the crosses between Solanum ×michoacanum and five F1 progeny obtained between 4x Solanum ×michoacanum and potato cultivars were found to be resistant to late blight. Among these forms, two tetraploid BC1 genotypes were also fertile males and presented desirable agronomic traits; therefore, they were considered potentially useful for further crosses. Although several research centers have obtained BC offspring, no potato variety originating from somatic hybrids has been registered to date. The reason for such poor results appears to be alterations produced by the somatic hybridization process.
Somatic fusion may lead to the deletion of whole chromosomes or their parts, aberrations, elimination, or recombination between homologous fragments of chromosomes (Orczyk et al., 2003). Moreover, it is a highly random technique, and its results cannot be accurately predicted; therefore, the structure of the nuclear and organellar genomes of somatic hybrids must be analyzed. The chromosome composition of the somatic hybrids Solanum villosum (+) Solanum tuberosum (Tarwacka et al., 2013), Solanum brevidens Phil. (+) Solanum tuberosum (Gavrilenko et al., 2002), and Solanum bulbocastanum (+) Solanum tuberosum (Iovene et al., 2007) was assessed using GISH and/or FISH cytogenetic techniques. The genome composition of Solanum pinnatisectum (+) Solanum tuberosum was evaluated using restriction fragment length polymorphism (RLFP) markers (Menke et al., 1996), whereas that of Solanum bulbocastanum (+) Solanum tuberosum (Bołtowicz et al., 2005) and Solanum brevidens (+) Solanum tuberosum (Polgar et al., 1999) was examined using random amplification of polymorphic DNA (RAPD) markers. Simple sequence repeat (SSR) markers were used to analyze the Solanum chacoense (+) Solanum tuberosum genome (Chen et al., 2013). Chromosome deletions and recombination between homologous chromosomes were observed in the nuclear genomes of all characterized somatic hybrids. For the first time, microarray-based diversity array technology (DArT) in combination with genetic and physical maps of potato was used for detailed analysis of the genomic composition of Solanum ×michoacanum (+) Solanum tuberosum somatic hybrids (Smyda-Dajmund et al., 2016). Differences in the composition of individual chromosomes within and between fusion combinations were noted; however, there was no complete chromosome elimination. In addition, similarities were observed in the composition of the analyzed chromosomes between the fusion combinations. Thus, DArT may be recommended for the analysis of nuclear genome structures of somatic hybrids in other plant species. Furthermore, the types of cytoplasmic DNA of Solanum ×michoacanum (+) Solanum tuberosum hybrids were analyzed using markers specific to chloroplast (cpDNA) and mitochondrial (mtDNA) DNA. The individual hybrids of Solanum ×michoacanum (+) Solanum tuberosum contained the cytoplasm of only one of the fusion components, which was randomly or non-randomly inherited. Changes in the structure of the nuclear and organellar genomes and different segregations of organelles in hybrid forms modify their mutual interactions and lead to epigenetic changes, resulting in the silencing of certain genes.
The analysis of the genome composition of Solanum ×michoacanum (+) Solanum tuberosum somatic hybrids was supplemented with the analysis of the degree of DNA methylation (Smyda-Dajmund et al., 2021). Quantitative and qualitative analyses of cytosine methylation in the genomic DNA of Solanum ×michoacanum (+) Solanum tuberosum indicated that somatic hybridization altered the level of cytosine methylation in potato somatic hybrids (Smyda-Dajmund et al., 2021). The degree of DNA methylation was increased in somatic hybrids compared with that in their parents, which may potentially affect the expression of desired trait. Comprehensive analyses of the nuclear and cytoplasmic genomes of many Solanum ×michoacanum (+) Solanum tuberosum somatic hybrids and autofusants, including the degree of cytosine methylation of their genomic DNA, as well as studies on the generative crosses of fusion products with cultivated potatoes, can provide novel information on fusion products and their use.
Somatic Hybridization of Other Crops and Ornamental Plants
In addition to potato, regeneration of interspecific hybrid plants following protoplast fusion has been achieved for tomato (Solanum lycopersicum L.) and gentians. In the case of tomato, somatic hybridization was aimed at utilizing the considerable variation in Solanum lycopersicoides Dun. to obtain plant materials that can be used in breeding. Experiments with PEG-mediated fusion between mesophyll protoplasts of Solanum lycopersicum (2n = 2x = 24) and cell suspension protoplasts of Solanum lycopersicoides (2n = 2x = 24) were conducted at the Department of Plant Genetics, Breeding and Biotechnology, Warsaw Agricultural University (SGGW). Of note, a transgenic line of Solanum lycopersicum, obtained after transformation with Agrobacterium tumefaciens carrying the neomycin phosphotransferase (nptII) and thaumatin genes, was used as the fusion partner, which enabled the development of a new system of hybrid selection on kanamycin-supplemented medium. Of the 50 calli, shoots were regenerated from two. These regenerants were slow-growing and possessed small, strongly wrinkled leaves and thick roots; however, they harbored transgenes derived from Solanum lycopersicum, as shown by Southern blot hybridization and PCR analyses. The work was the first in Poland that used GISH to describe the chromosomal composition of somatic hybrids (Kulawiec et al., 2003).
In the case of gentians, somatic hybrids representing different nucleocytoplasmic combinations could be used as new ornamental varieties and valuable sources of secondary metabolites (Tomiczak et al., 2015). Electrofusion of mesophyll and cell-suspension protoplasts of diploid Gentiana kurroo Royle (2n = 2x = 26), tetraploid Gentiana cruciata L. (2n = 2x = 52), and another tetraploid Gentiana tibetica King (2n = 2x = 52) was conducted at the Polish Academy of Sciences Botanical Garden – Center for Biological Diversity Conservation (PAS BG-CBDC) in Powsin. These experiments led to the regeneration of the first somatic hybrids within the genus Gentiana in the world, between Gentiana kurroo and Gentiana cruciata L. as well as between Gentiana cruciata and Gentiana tibetica King (Tomiczak et al., 2015). The genome composition of hybrids from both combinations and their genetic similarity to the parental species were evaluated using amplified fragment length polymorphism (AFLP) and inter-simple sequence repeat (ISSR) markers, and chloroplast inheritance was established using cleaved amplified polymorphic sequences (CAPS) markers, developed intentionally for these studies. Repeated flow cytometry analyses provided the evidence of genetic instability and gradual elimination of nuclear DNA in the highly polyploid Gentiana kurroo (+) Gentiana cruciata hybrids (Tomiczak et al., 2017) but no significant decrease or increase in the total DNA content of hybrids between Gentiana cruciata and Gentiana tibetica (Tomiczak, 2020). Moreover, the first report on the use of FISH and GISH in gentians has been published recently (Tomiczak, 2020).
. Conclusions and Future Prospects
Genetic recombination and selection are at the core of plant breeding. Since long, development of methods that may lead to specific and targeted genetic modifications of plants has been a desirable goal in fundamental and applied research worldwide and in Poland. Thanks to the use of biotechnology, specifically in vitro cultures, and their combination with interspecific hybridization, the genetic variability of many plant species could be enhanced and, in particular, their cultivated forms could be enriched with valuable traits from wild species. Polish research on wide hybridization complemented by in vitro pollination and/or embryo rescue techniques has revealed the following:
Fertilization barriers can be overcome to generate intergeneric hybrids between Salix and Populus species. An in vitro system enabling the production of hybrids between willows and poplars with the characteristics of both parents is of great importance for increasing biomass production. Recent studies have opened new avenues for improving other traits of trees, such as pathogen resistance, inherited from one parent (Bagniewska-Zadworna et al., 2011).
Brassicanapus hybrid plants can be obtained by crossing Brassica oleracea and Brassica rapa. The resynthesized “genetically new” Brassica napus is strategically important for practical breeding, because it expands the genetic pool of this plant (Szała et al., 2019). Interspecific hybridization and use of relatives for crop improvement in the agriculturally significant Brassica genus may be implemented with the support of gene banks and information resources (Katche et al., 2019).
Interspecific hybridization is an efficient way to introgress desirable traits, including relevant agronomic quality characteristics, in oilseed rape.
The application of embryo rescue allows for increasing the number of interspecific hybrids obtained after crossing Allium cepa with Allium fistulosum, Allium roylei, and Allium galanthum and offers prospects for the transfer of resistance to many diseases and pests as well as CMS into cultivated onions.
Contrary to the research on wide hybridization, protoplast fusion in Poland is of little commercial interest. Somatic hybridization was described as “theoretically elegant but technically demanding” (Simmonds & Smartt, 1999), often requiring an incommensurable amount of work and cost relative to the actual achievements. These limitations are primarily due to the highly random nature of these techniques. In fact, despite many investigations, obtained somatic hybrids hardly ever have direct breeding value because of their high genetic instability, low fertility, or lack of expression of desirable traits. Thus, interest in this technique has waned. However, somatic hybrids still constitute a unique model system for studying the interaction of two distant genomes in the same individual and the processes of stabilization of allopolyploid genomes (Arcioni & Pupilli, 2003). Furthermore, somatic fusion may support conventional breeding methods, because it is more efficient than genetic transformation for transferring polygenically inherited traits and those conferred by organellar genomes.