Introduction
Karst sinkholes have a unique scientific value and are of significant economic benefit to the tourism industry (Shui et al., 2015). Most sinkholes are distributed in areas of mature karst and in tropical humid regions (Waltham & Zhang, 2006). In southwestern China, sinkholes are usually enclosed by sheer cliffs, usually more than 100 m deep and 100 m wide, generating a closed environment with higher humidity and lower temperatures compared to those in the surrounding aboveground forested areas and also providing a more habitable environment for living organisms (W. H. Chen et al., 2004; J. J. Wang & Guo, 2007; Zhu, 2010). Sinkholes can be divided into the following two types according to the manner of their formation (Zhu et al., 2003): (i) they can be formed as a result of underground water flow leading to rock dissolution, then to the development of caves, followed by the collapse of surface rocks; or (ii) they can develop due to rock dissolution and erosion by surface water flow. Belowground level forests in sinkholes (UFS – underground forest sinkhole) develop on gravel slopes at the base of sinkholes. This is a long-term successional process that generates forests in unique hydrothermal environments with relatively independent structures connected with underground rivers (Robinson & Wells, 1956).
Bryophytes are an important component of forest communities and are markedly linked to forest succession (Sun et al., 2007). Studies of bryophytes in sinkholes have focused on diversity and distribution relative to the vertical depth of sinkholes. For example, X. F. Li et al. (2018) studied bryophyte diversity in the Monkey-Ear Sinkhole of Guizhou; C. Y. Li et al. (2019) and J. Wu et al. (2019) used cave liverworts and species of Hypopterygiaceae, respectively, to reveal the distribution of bryophytes along vertical gradients of sinkholes. There have been several studies on various aspects of bryophytes from surface-level forests (Delucia et al., 2010; Dynesius et al., 2008; J. J. Wang & Guo, 2007; Z. H. Wang & Zhang, 2010). However, there have been no studies comparing bryophytes of surface-level forests surrounding sinkholes with bryophytes of below-ground level forests in sinkholes.
Soil moisture gradually decreases from the lowest point of the sinkhole up to its sides (Lin, 2005). Differences in direction, slope, and the degree of disturbance of the gravel slopes increase the complexity of underground forests. Therefore, to determine the potential of sinkholes as a refuge and to establish the methods of protection of bryophyte diversity, we analyzed the Monkey-Ear Sinkhole in southwestern China. We hypothesized that the richness and diversity of bryophyte species in the sinkhole forest would be higher than in the surrounding forest, which develops outside the sinkhole. We also hypothesized that bryophyte diversity would be correlated with environmental factors. If the first hypothesis is validated, our results will reveal the importance of sinkhole forests for the maintenance of bryophyte diversity. Testing the second hypothesis will reveal crucial drivers of bryophyte diversity and help to explain the differences in bryophyte diversity between the forests in sinkholes and surroundings.
Material and Methods
Study Area
The area of study is situated in a karstificated plateau in the Guizhou Province, Southwestern China (Figure 1), which has a subtropical monsoon humid climate. The average temperature is 10.6–15.30 °C, and the annual rainfall is 1,141–1,547 mm.
Figure 1
(A) Map showing location of the study area in Guizhou Province, China. (B) Depiction of the cross section of the sinkhole, showing location of the forest in the sinkhole, and two surface forests.
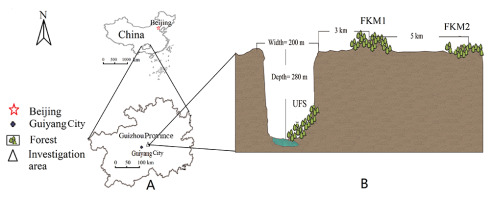
Monkey-Ear Sinkhole is massive, 300 m in diameter and 280 m deep. It is a typical karst negative landform in a plateau which has an average altitude of 1,100 m. The sinkhole was formed over an extremely long period of time, both from weakly acidic rainfall filtering through surface limestone and due to the dissolving of the underlying rock to form a massive underground cave. It also partially developed as a result of similar erosional and dissolution processes of an underground stream, finally leading to the collapse of the top rock of the cave to form the open sinkhole. Successional processes led to the eventual growth of a subtropical broad-leaved forest at the base of the sinkhole, covering an area of about 2,000 m2. A considerable portion of the plateau surface was affected by rock desertification, leaving exposed rock surface. The vegetation is in poor condition and appears to be increasingly subjected to degradation. Two forests on the plateau surface, each with an area about 2,000 m2, were selected for comparison with the UFS (Figure 2); the first was located 3 km from the top of the sinkhole, and designated as FKM1 (Forest Karst Mountain 1); the second, 8 km from the sinkhole, designated FKM2 (Forest Karst Mountain 2). Their basic characteristics are listed in Table 1.
Figure 2
(A) Top view of the belowground level forest in the sinkhole (UFS). (B) View from the base to the top of the sinkhole (UFS). (C) Lateral view along the sinkhole (UFS). (D) View of the first forest on karst mountain plateau (FKM1). (E) View of the second forest onthe karst mountain plateau (FKM2).
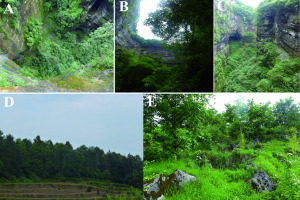
Table 1
Details of the forest survey plots.
Sample Collection
From September 2017 to April 2018, the three forests were explored six times. Within each forest, 12 sample points were established with regular spacing along the transect, and within each collection point, five 10 × 10-cm2 squares were sampled randomly. A total of 180 bryophyte samples and 180 soil samples were collected from the three forests. The coverage, habitat, latitude, longitude, and altitude of all samples were recorded in detail, and environmental parameters were measured. Air temperature and humidity was measured with a hand-held air temperature and humidity meter (HT-635; Guangzhou Hongcheng, China), and illumination using a digital illuminometer (PM6612L; Huayi United, China). The canopy was evaluated by processing the sample photos with Photoshop software (CC 2018) (Qi et al., 2009) by calculating its coverage according to the formula
Specimen Identification
Bryophytes were identified using classical morphological identification techniques, using an HWG-1 (Shanghai Yuguang, China) anatomical lens and a XSZ-107TS (Shenzhen Yiqiang, China) microscope, and the following floras: Flora Bryophytorum Sinicorum (Gao, 1996; Hu, 2005; X. J. Li, 2006; P. C. Wu, 2011; P. C. Wu & Jia, 2004), Genera Hepaticopsida et Anthocerotopsida Sinicorum (Gao & Jia, 2006), and the Moss Flora of China (Chien et al., 1999, 2003; Hu et al., 2008; X.-J. Li et al., 2001).
Soil organic carbon (SOC) was measured using concentrated sulfuric acid and potassium dichromate heated in an oil bath at 180 °C for 5 min (X. L. Li, 2013). Total nitrogen (TN) was measured using a half-automated Kjeldahl Distillation Unit (KDN-520; Ningbo rodek, China) (Jiang & Fan, 1981). Total phosphorus (TP) was measured using molybdenum antimony colorimetric method by CARY Bio UV-visible spectrophotometer (Evolution 201; Thermo Fisher, U.S.) (Yuan, 1987). Total potassium (TK) was measured by flame atomic absorption spectrophotometry (ICE-350; Thermo Fisher, U.S.) (M. Wang, 2016). Alkali-hydrolyzed nitrogen (AN) was titrated with standard sulfuric acid solution by alkali-hydrolysis-diffusion method (Bao, 2000). Available phosphorus (AP) was estimated by sodium cyanide carbonate-molybdenum blue colorimetry (Evolution 201) (Bao, 2000). Available potassium (AK) was determined by ammonium acetate leaching and flame photometry (ICE-350) (Bao, 2000).
Data Analysis
The following Shannon–Wiener diversity index, Pielou evenness index, ecological importance value and Jaccard index were calculated using the bryophyte data collected. We selected three forests as our study sites, and each forest was considered as an independent sampling unit, and we calculated frequency and the coverage of species per sampling unit. We defined and classified the life forms of bryophytes by referring to Mägdefrau’s (1982) and Bates’s (1998) systems. Dominant species can better reflect the adaptability of plants to environmental factors and the accumulation of soil nutrients. Regarding the value of ecological importance, we determined the top six species in each forest, and they were further selected as research objects. The relationships between the forest bryophyte species (the top six species from each forest), environmental factors, and soil nutrients in the three forests were analyzed using redundancy analysis (RDA) in CANOCO 4.5 software. One-way ANOVA in SPSS 10.0 (IBM; U.S.) was used to determine the differences between the species abundances and soil nutrients in the three forests. Box plots were established to compare the differences of soil nutrients in the three forests using ORIGIN 9.0 software.
Results
Bryophyte Species Diversity
Seventy-one bryophyte taxa from 36 genera and 23 families were recorded from UFS (Figure 3), including 24 liverwort taxa. The dominant families were Brachytheciaceae, Fissidentaceae, Plagiochilaceae, and Hypopterygiaceae and the dominant species were Conocephalum conicum, Homaliodendron montagneanum, Fissidens cristatus, and Leucobryum glaucum. Less than half of the number of taxa were recorded from FKM1, including 29 bryophyte taxa from 16 genera and 12 families. Only two liverwort taxa were recorded. With the exception of Brachytheciaceae, a completely different assemblage of dominant families was recorded, including Thuidiaceae, Hypnaceae, Mniaceae, and the dominant species were Thuidium pristocalyx, Plagiomnium arbusculum, Entodon compressus, and Cyrto-hypnum pygmaeum. The plateau forest more distant to the sinkhole (FKM2) included only 22 bryophyte taxa belonging to 17 genera and eight families. There were no liverwort taxa present and the dominant families, Brachytheciaceae, Thuidiaceae and Hypnaceae, were the same as those of FKM1, but also included Pottiaceae, a family characteristic of dry environments. The dominant species were Brachythecium homocladum, Claopodium gracillimum, Hypnum leptothallum, and Brachythecium perminusculum. Ninety-three percent of the bryophytes in karst mountain sinkhole were not found in surface forests. The ecological importance values of the bryophytes significantly differed in the top 10 species in each forest (Table 2). For bryophytes in FKM1, the maximum value was 14 times higher than the minimum of the ecologically important values, 19 times in FKM2, but only twice in UFS, demonstrating that the living environment of bryophytes in the sinkhole forest is more complex than that of the forests on the plateau surface (Table 2).
Figure 3
Diversity of bryophytes in forest enclosed within the sinkhole (UFS) and in the two open forests (FKM1 and FKM2) on the plateau surface.
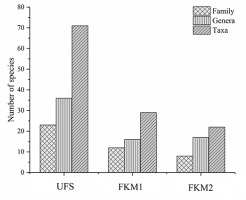
Table 2
Dominant bryophyte species ranked by importance values in the forest within the sinkhole (UFS) and in the forests on the plateau surface (FKM1 and FKM2).
Alpha Diversity Characteristics
The diversity index of bryophytes in the sinkhole forest was significantly different from that of the surface forest (p < 0.05) (Table 3, Figure 4). The trend of the Shannon–Wiener diversity index was as follows: UFS > FKM1 > FKM2; in contrast, the trend of the Pielou evenness index was as follows: UFS < FKM1 < FKM2. Thus, the Pielou evenness index showed a negative trend compared to the Shannon–Wiener diversity index.
Table 3
The diversity index of bryophytes (mean ± standard deviation) in the forest within the sinkhole (UFS) and in the forests on the plateau surface (FKM1 and FKM2).
Pielou evenness index | Shannon–Wiener diversity index | |
UFS | 0.054 ± 0.004 a | 3.868 ± 0.002 a |
FKM1 | 0.091 ± 0.005 b | 2.66 ± 0.02 b |
FKM2 | 0.102 ± 0.003 c | 2.241 ± 0.001 c |
Beta Diversity Characteristics
In this study, the Jaccard index was used to measure the differences among the three sites (Table 4). The Jaccard index between any two compared sites was below 0.25, indicating that they were highly dissimilar, evidently due to their different topographies and their habitats.
Bryophyte Life Forms
The classification of species into life forms can combine species with similar environmental requirements into one category (Mägdefrau, 1982; Mälson & Rydin, 2009). Field observations combined with indoor identification showed that the life forms of bryophytes in the study areas can be divided into 10 types, namely, annuals, short turfs, tall turfs, cushions, mats, wefts, Meteorium type, tails, fans, and dendroids. The sinkhole forest included all 10 above-mentioned life forms (Figure 5), whereas plateau surface forests had significantly less life forms; four in FKM1 (short turfs, cushions, mats, and wefts), and only two in more distant FKM2 (short turfs and wefts).
Diversity of Bryophyte Communities
The diversity of a bryophyte community reflects the complexity of an environment. The statistical analysis of the bryophyte communities revealed that the number of mixed communities varied significantly in each forest (Figure 5). Thirty-six mixed communities were recorded in UFS; significantly less communities, i.e., only six in FKM1 and only one bryophyte community in FKM2 were recorded, which included Timmiella anomala and Gammiella pterogonioides.
Soil Chemistry Characteristics
There were considerable differences in soil chemistry between the three forests (p < 0.05) (Figure 6), with significantly higher levels of minerals in the sinkhole forest compared to the two surface forests. SOC, TN, TP, TK, AN, AP, and AK were significantly higher in the sinkhole forest than in the surface forests. SOC, TN, TP, AN, AP, and AK, but not TK, were significantly higher in the surface forest closer to the sinkhole (FKM1) than those in the more distant forest (FKM2).
Figure 6
Soil nutrients: soil organic carbon, total nitrogen, total phosphorus, total potassium, alkali-hydrolyzed nitrogen, available phosphorus, and available potassium in the sinkhole forest (UFS) and the two surface forests (FKM1 and FKM2). Bottom and top sections ofthe box plots indicate the inner quartile ranges. The horizontal bar within the box represents the median. Whiskers indicate spread.
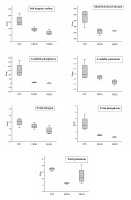
Redundancy Analysis (RDA) of Forest Bryophyte Species, Environmental Factors, and Soil Nutrients
The relationships between forest bryophyte species, environmental factors, and soil nutrients of the three forests were determined by RDA (Figure 7). The characteristic values of the first axis and the second axis were 0.515 and 0.292, respectively, explaining 51.5% and 29.2% of the adaptation characteristics of the species to soil and environment. The cumulative interpretation value was 80.7%, reflecting significant evidence of environment–soil–species relationships. RDA showed that light, temperature, humidity, TN, TP, and SOC were the main factors affecting species distribution. Species with strong tolerance to high temperature and aridity were observed in the negative axis; species with low tolerance to high temperature and aridity were observed in the positive axis. Humidity, canopy density, TP, TN, TK, and SOC were observed in the positive axis, indicating that shaded and humid environments had an evident effect on the accumulation of soil nutrients. Light was the main factor affecting the distribution of bryophytes in the sinkhole forest, whereas temperature, humidity, and soil nutrients were the main factors affecting bryophyte distribution in the two surface forests (FKM1 and FKM2).
Figure 7
Redundancy analysis (RDA), two-dimensional ordination diagram of species, environmental factors, and soil factors. The green solid arrow represents the environmental factors variable, the red solid arrow represents the soil factors variable, and the black solid arrow represents the number of species in the sequencing diagram. The angle between the arrows represents the correlation between variables, and the angle cosine represents the correlation coefficient between the two variables. Abbreviations of the species: Con.con – Conocephalum conicum; Leu.gla – Leucobryum glaucum; Hom.mon – Homaliodendron montagneanum; Mak.cri – Makinoa crispata; Fis.cri – Fissidens cristatus; Pla.rhy – Plagiomnium rhynchophorum; Thu.pri – Thuidium pristocalyx; Cyrto-hyp.pyg – Cyrto-hypnum pygmaeum; Pla.arb – Plagiomnium arbusculum; Eur.ang – Eurhynchium angustirete; Ent.com – Entodon compressus; Dre.rev – Drepanocladus revolvens; Bra.hom – Brachythecium homocladum; Bra.per – Brachythecium perminusculum; Cla.gra – Claopodium gracillimum; Tim.ano – Timmiella anomala; Hyp.lep – Hypnum leptothallum; Bra.rot – Brachythecium rotaenum. Other abbreviations: Hum. – humidity; Lig. – light; Sha.– shading; Tem. – temperature; TK – total potassium; TN – total nitrogen; TP – total phosphorus; SOC – soil organic carbon.
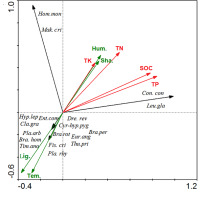
Discussion
Impact of Sinkhole Environment on Diversity, Community, and Life Forms of Bryophytes
There were significantly higher numbers of bryophyte species, mixed communities, and life forms found in the sinkhole forest than in the two surface forests. The key reason for this is that the high cliffs surrounding the UFS shade the forest and reduce the rate of water loss (Zhu et al., 2003), while humidity is increased by the evaporation of water from the underground river. For bryophyte life forms, differences in light levels and moisture availability contributed to the high heterogeneity of the sinkhole forest, providing more habitats that support a greater range of bryophyte life forms (C. Li et al., 2020; Pharo, 2002; Strazdina et al., 2013). Another important reason for bryophyte diversity is the fact that the relatively closed and isolated environment of the sinkhole forest is also less subjected to human disturbance than surface sites, a factor important in the preservation of bryophyte species diversity (R. Liu et al., 2019; Virtanen et al., 2015). The two surface forests are subjected to frequent human disturbance and have lower humidity (Jian et al., 2018). The forests become increasingly more uniform in terms of species composition and forest structure, such that many vulnerable bryophyte species are gradually replaced by a fewer, more robust species eventually comprising a single community (S. Wang et al., 2015). This lack of diversity of bryophytes contributes to further vulnerability of the surface forests and leads to their higher susceptibility to damage by natural and human activities (R. Liu et al., 2019). Thus, the significantly higher bryophyte diversity in the sinkhole forest compared to that of the two forests on the plateau surface showed that the sinkhole forest provided a better condition for bryophyte maintenance and thus it could be a refuge for vulnerable bryophyte species.
Effects of Environmental Factors on Bryophyte Diversity
Bryophytes are small plants lacking vascular tissues and true roots, which are highly sensitive to environmental changes. According to the redundancy analysis, light was the main factor limiting the distribution of bryophytes in the sinkhole forest; but a combination of temperature, humidity, and soil nutrients were the main contributory factors limiting bryophyte distribution in the two surface forests studied. The unique negative landform of the sinkhole increased the humidity of forest environment. Humidity, however, is not the main factor limiting the distribution of bryophytes in areas that experience particularly high atmospheric moisture (W. Q. Liu et al., 2006). As demonstrated by our study, light is the major factor affecting bryophyte distribution in the sinkhole forest. These results are consistent with those reported by W. Q. Liu et al. (2006), who studied environmental factors affecting bryophyte distribution on black rock in Guangdong. Furthermore, in our study, there was more light in the two surface forests than that observed in UFS. Therefore, the temperatures were also higher in these surface forests, which affected bryophyte growth and development because of temperature affecting above and underground parts of the plant via restriction of the enzymatic activity. The microenvironment of the surface forest that was more distant to the sinkhole was characterized by impoverished vegetation, minimal soil water storage capacity, and exposure to direct sunlight, thus it was relatively dry. Bryophytes rely principally on absorption of moisture from the atmosphere directly through stems and leaves and lack protective cuticle to minimize water loss by evaporation. Thus, bryophyte distribution in FKM2 was principally affected by low humidity. This result is consistent with that of L.-L. Wu et al. (2010) who studied the relationships between bryophyte distribution and environmental factors in Yangjifeng Nature Reserve, China. On the other hand, the cliffs of the sinkhole impede the penetration of light into the sinkhole forest and additionally provide shading and protection from surface winds, which together contribute to the high humidity that is conducive to maintaining bryophyte diversity (Pharo et al., 2005).
Relationship Between Soil Nutrients and Species Distribution
The sinkhole provides a unique, shady environment with high humidity, favorable for decomposition of biological residues by soil microorganisms, including fungi and bacteria, resulting in higher soil fertility (Davis, 1986). Compared to the sinkhole forest, the two surface forests are much hotter and drier, and experience considerably higher light levels, all factors which have a negative effect on accumulation of soil nutrients (Shen et al., 2018). Soil fertility is an important driving force for forest productivity and biomass production (Schröder & Fleig, 2017). It can also facilitate the activity of soil microorganisms (Rola et al., 2015) and influence the survival of plants and animals (Treseder & Vitousek, 2001), as well as the maintenance of biodiversity. The soil fertility of the sinkhole forest was much higher than that of the two surface forests and thus, indirectly, the sinkhole forest is advantageous for the maintenance of bryophyte diversity.
Conclusions
The sinkhole forest provides a natural refuge for bryophyte species, increasing their richness and diversity, which is higher than in the surrounding forests which develop outside the sinkhole. The shading and cooling generated by the enclosing cliffs reduces the rate of water loss and increases the humidity inside the sinkhole. The differences in light levels and water availability contribute to the high heterogeneity of the sinkhole forest. Higher levels of soil nutrients were found in sinkhole forest than in surface forests, which further indirectly promoted bryophyte diversity.