. Introduction
Long-stalked pondweed (Potamogeton praelongus) is a submerged perennial aquatic plant with a long perennial rhizome (Kaplan, 2010). The shoot length varies depending on water depth and transparency (Prausová, Kozelková, Tomášová, & Brodský, 2017); the seeds have a physiological dormancy and germinate after stratification (Prausová et al., 2013). Genetic analyses have revealed a very low genetic diversity in existing micropopulations of the Czech Republic (CR) (Kitner et al., 2013).
The distribution of P. praelongus is classified as circumpolar and suboceanic. The species mainly grows in the boreal climatic zone, rarely extending beyond the polar circle. In Asia, it is found between the fifty-second parallel and the polar circle (Hultén, 1964). In America, it occurs in several parts of the US and Canada (Egertson et al., 2004). Some isolated localities exist in central Mexico (Hultén, 1964). The species was recently found in the western (Bennike & Anderson, 1998) and southwestern part of Greenland (Vöge, 2002). In Europe, the species prefers slightly alkaline water in Norway, Sweden, Denmark, Finland, and Chudskoe Lake (Peipsi) on the Estonia-Russia border. It also occurs in the Netherlands, northwestern Germany, the Spanish Pyrenees, and Slovenia. More than 100 sites have been recorded in Poland (Gąsiorowski & Kupryjanowicz, 2009).
Potamogeton praelongus occurs in lakes, river floodplains (river oxbows, pools), moderately flowing water, ponds, and reservoirs. It grows especially in unpolluted, mesotrophic, 0.2–2.0-m deep water on humic or sandy soils, and clayish, muddy, or peaty beds (Casper & Krausch, 1981). In Nordic countries, it grows in streams and lakes with high water transparency and mainly gravel bottoms in water up to several meters (ca. 4 m, own observation). In the CR, it usually grows in river oxbows or backwater pools in 0.2–0.7-m deep water, where it requires a moderate shade to prevent overheating (Prausová, Kozelková, Tomášová, & Brodský, 2017). In Slovenia (Gaberščik et al., 2005), the species occurs in well-vegetated or slowly running, 2–3-m deep waters, even in flooded alluvial plains. It does not grow well in shallow, heated, and illuminated waters and prefers a 1–2-m water column (Hough & Wetzel, 1977). Ellenberg et al. (1992) mentioned that the species is light-demanding, only rarely growing in conditions with relative lighting less than 40%.
The response of aquatic plants to environmental variability has been intensively discussed during the last few decades (Bornette & Puijalon, 2011; Lacoul & Freedman, 2006). Different life forms of vascular plants use resources (e.g., light and nutrients) in specific ways and differ in their response to environmental changes (Akasaka & Takamura, 2011; Alahuhta & Heino, 2013; Netten et al., 2011; Vöge 2002). Ecological preferences, life forms, and the dispersal of particular plant species are used to investigate the use of functional traits of aquatic plants, as well as their morphology (such as leaf area and morphological index), to assess eutrophication in freshwater lakes (Stefanidis & Papastergiadou, 2019). Eutrophication, which results in high phytoplankton biomass and low summer water transparency, has led to problems not only in densely populated Central Europe but also in the Nordic part of Europe (Mäemets et al., 2010; Rintanen, 1996). Increasing eutrophication also correlates with the decline in aquatic plant species richness; e.g., in 100 Danish lakes and streams, the number of Potamogeton species has declined from 16 to nine (Sand-Jensen et al., 2000). Fast-growing species capable of regeneration after disturbances (e.g., weed-cutting and dredging) prevail instead of the once-common Potamogeton species, which are typical of oligotrophic conditions (Sand-Jensen et al., 2000). As P. praelongus has a relatively high tolerance range, it is able to spread to localities that were once oligotrophic while disappearing from highly eutrophic waters (Mäemets et al., 2010). Underwater light availability is one of the most important environmental factors affecting the growth and morphology of aquatic plants (Barko et al., 1986). In the case of low light availability in the water column, submerged macrophytes might adopt one of two distinct strategies: elongation of shoot length towards the water surface to alleviate low-light stress or enhancing low-light tolerance by photosynthetic adjustments (Chen et al., 2016).
Although underwater light availability in shallow waters is favorable, the high temperature of water during the growing season supports the expansion of hygrophytes, algae, plankton, and aquatic mosses instead of the less competitive P. praelongus (Prausová, 2016; Prausová, Kozelková, Tomášová, & Brodský, 2017). Strong eutrophication of shallow water bodies results in a large decline in macrophytes, caused by the intense shading from phytoplankton and filamentous algae (Lacoul & Freedman, 2006). Parts of P. praelongus shoots covered with filamentous algae necrotize, forming a smelly and muddy black layer of organic material on the bottom (Prausová, Kozelková, et al., 2015). The washing away of P. praelongus shoots and increased disturbance of the populations during high water flow and floods have also been recorded (Grinberga, 2010; Husák & Kaplan, 1997). The influence of animals is another factor limiting the growth of P. praelongus (Egertson et al., 2004; Prausová, Kozelková, Tomášová, Brodský, Havelka, Dvořák, et al. 2017; Prausová, Kozelková, et al., 2015).
Potamogeton praelongus is a critically endangered species in the CR (Čeřovský et al., 2001; Grulich, 2012). About 90% of the historic Czech localities have disappeared due to eutrophication, successional extinction, destruction of the natural habitats, the introduction of herbivorous fish, and intensive fishing, as well as water pollution from chemicals used on the surrounding agricultural landscapes (forests, gardens, and banks of water bodies) (Prausová, Kozelková, Tomášová, & Brodský, 2017). Therefore, only one native population currently exists, located in the oxbow of the Orlice River near Hradec Králové in Eastern Bohemia. According to Law No. 114/1992 Coll., on nature conservation and landscape protection, the species is listed as a critically threatened species in the CR. This species is included in The IUCN (International Union for Conservation of Nature and Natural Resources) Red List of Threatened Species in the least concern (LC) category of species protection. According to the List, it is a widespread species with stable populations that are not in danger. However, the IUCN List also states that local loss of the species requires attention, and it is necessary to gather new information about the level of risk throughout its distribution range (Lansdown, 2014). In the European red list, the species is also in the LC category (Bilz et al., 2011) but is considered a critically endangered species in Central Europe (Pott, 1995; Schubert et al., 1995). Within the framework of a rescue program for P. praelongus, reintroductions in the CR have been currently restricted to the Orlice and Ploučnice basins. The species has been experimentally grown in several revitalized pools in the Protected Landscape Area of Kokorinsko in Northern Bohemia.
The first aim of this study was to find differences between site conditions in the optimal biotopes of P. praelongus in North-European countries (Norway, southern Sweden), Poland (also glaciated during the Last Glacial Maximum), and Czech sites with a decreasing population. Sweden, Norway, and Poland were selected for this study because of the high density of natural P. praelongus sites in these countries. The second aim was to compare morphometric characteristics of shoots and leaves of the species, indicating its adaptation to different stress factors, and links to several bioindicators of water trophy, such as the species composition of vascular plants, desmids, and cyanobacteria. As P. praelongus grows in extreme ecological conditions for submerged mesotrophic macrophytes in the CR, the third aim of this study was to provide recommendations on how to restore its last native site in the Orlice River floodplain.
. Material and Methods
Field Data Collection
Potamogeton praelongus sites were studied in 2014–2016 in four European countries ranging between 50–67° of latitude and 10–23° of longitude (Figure 1). These were localities in the CR (five pools and three river oxbows; observation for the whole growing seasons 2014–2016), southern Sweden (four lakes; September 16–18, 2014), Norway (14 lakes; July 18–26, 2015), and Poland (five localities: three lakes, one lido, and one river lagoon; August 26–27, 2016). Sweden, Norway, and Poland were selected for this study because of the high density of natural P. praelongus sites in these countries.
Figure 1
Location of the studied localities: Poland (1), Sweden (2), Czech Republic (3), and Norway (4).
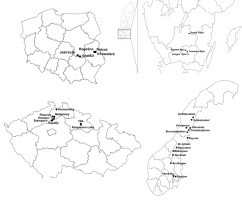
Water parameters (electrical conductivity, pH, dissolved oxygen content) were measured in sites with healthy P. praelongus populations by portable devices (Hach HQ 30d) at a water depth of 20–30 cm, water transparency by Secchi desk, and illumination by luxmeter (Voltcraft LX-1108). Shade level (%) was calculated as the difference between 100% and the ratio (%) of illumination (lx) of the P. praelongus site to the illumination (lx) of an open, fully lighted place. In Sweden, Norway, and Poland, only one measurement was done at each site, while parameters were measured repetitively and subsequently averaged in the Czech localities. The WorldClim database version 2 (Fick & Hijmans, 2017) for 1970–2000 was used to compare the thermic conditions and annual precipitation in particular localities. Annual precipitation reflects the fluctuating water regime typical of the (mostly 2-month) hot and dry period in summer, observed in the last few years in the CR.
Potamogeton praelongus and other species of vascular plants were observed and determined in open water (from a boat) and the littoral zones. Undetermined species were gathered and identified by specialists (A. Hájek, M. Hroneš, and Z. Kaplan). The nomenclature of vascular plant species followed Danihelka et al. (2012) and The Plant List (2013).
The depth of P. praelongus sampling for morphological measurements was site-specific. Morphological parameters of 15–20 individuals of P. praelongus at each locality were examined in situ and in vivo by measuring the internode length and the length and width of three leaves in the central part of a shoot. Leaf length was measured from the point of leaf intersection with the shoot to the leaf apex. The widest part of the leaf was measured for leaf width. All measurements were done on fresh biological material to an accuracy of 1 mm. All these parameters were selected because of their ability to indicate environmental variability and availability to be measured in all the studied sites (fertile individuals were not found at all localities). To verify a preference of vegetative to generative reproduction, we also counted the number of germinating plants of P. praelongus, the determination of which was based on our knowledge of seed germination stages and plantlet growth from laboratory germination tests (Prausová et al., 2013; Prausová, Sikorová, & Šafářová, 2015).
Site conditions recorded in all localities (Table S1) were used for comparison between the Czech and the other localities. Since five Czech localities were monitored in 2014–2016, the mean values of the measured site condition were used.
Statistical Analysis
The environmental data collected to explain the differences between Potamogeton praelongus sites in North and Central Europe were statistically evaluated by principal component analysis (PCA). The environmental conditions were characterized by mean monthly temperature, annual temperature range, and annual precipitation [taken from the database WorldClim version 2 (Fick & Hijmans, 2017)], as well as pH, water transparency, shade level, electrical conductivity, water depth, and content of dissolved oxygen. Environmental conditions were used as response data after logarithmic transforms due to nonnormal distributions. Data on the environment variables were centered; thus, no standardization was performed. Data locations were not centered or standardized.
The floristic data of vascular plants were coded as 1 or 0 for presence or absence, respectively. A preliminary survey of the data revealed the length of the longest gradient exceeded 4. The unimodal detrended correspondence analysis (DCA) method without any data transformation was selected as the most suitable to evaluate site similarities based on vascular plant species composition. TWINSPAN in JUICE 7 (Roleček et al., 2009; Tichý, 2020) was used to compare the vascular plant species composition of the localities according to the Sorensen index of dissimilarity (Maximum Dissimilarity Index 0.657) and created three clusters. A synoptic table was arranged according to the class of species frequency in the localities of each cluster, and only species occurring in frequency classes II–V (21%–100%) are shown in Table 1.
Table 1
Synoptic table of the most frequent species of aquatic plants in three groups of localities (see Table 3).
Table 2
Statistical evaluation of differences in morphological parameters of Potamogeton praelongus leaves and shoots.
Table 3
Characteristics of the studied localities (Groups 1–3: differentiation of localities according to trophy and occurrence of vascular plants).
[i] Group 1: altitude (m a.s.l.) – min 40, max 880, mean 317.33, SD 245.46; area (ha) – min 0.155, max 256, mean 78.54, SD 92.54. Group 2: altitude (m a.s.l.) – min 140, max 500, mean 212, SD 123.83; Area (ha) – min 6, max 112,700, mean 12,651.34, SD 35,372.87. Group 3: altitude (m a.s.l.) – min 180, max 480, mean 254.18, SD 105.81; area (ha) – min 0.01, max 46, mean 5,46, SD 12.58.
The mutual similarity of localities based on species composition correlated with environmental data was tested by constrained multivariate analysis. Binary coded floristic data were projected on a graph based on environmental data, which included air temperature, water temperature, pH, water transparency, shade level, electrical conductivity, water depth, and dissolved oxygen content. When choosing a suitable method, the longest gradient length in the biological data, which exceeded 4 (exactly 4.63), had to be taken into account. Data were evaluated by canonical correspondence analysis (CCA) with a permutation test (999 permutation). Subsequently, forward selection was used to estimate the significance of individual environmental characteristics. All multivariate data were tested in CANOCO 5 (Ter Braak & Šmilauer, 2012).
According to our field experience, the localities were divided into three groups, combining site conditions and the mix of vascular plant, algae, and cyanobacteria species. In the first step, two groups of localities were distinguished according to water transparency (lower than 100 cm, and higher than 100 cm). In the second step, three groups of localities (first and second groups, water transparency higher than 100 cm; third group, water transparency lower than 100 cm) were differentiated according to the occurrence of vascular plant, desmid, and cyanobacteria bioindicator species: Group 1 – oligo-mesotrophic species (Myriophyllum alterniflorum, Potamogeton alpinus, Potamogeton perfoliatus, Potamogeton ×nitens, Utricularia minor, Cosmarium botrytis, Eunastrum elegans, Pleurotaenium trabecula, and Chroococcus turgidus); Group 2 – mesotrophic-mildly eutrophic species (Hydrocharis morsus-ranae, Myriophyllum spicatum, Nuphar lutea, Potamogeton natans, P. obtusifolius, Utricularia australis, Cosmarium granatum, Staurastrum avicula, Phormidium autumnale, and Gomphospaeria aponina); Group 3 – eutrophic species (Ceratophyllum demersum, Elodea canadensis, Potamogeton berchtoldii, P. crispus, Stuckenia pectinata, Closterium limneticum, Microcystis wesenbergii, and Planktothrix agardhii).
On the basis of specific morphological parameters, the differences between localities were evaluated by one-way analysis of variance or a nonparametric version of this test (Kruskal–Wallis test). Several localities had to be excluded because of missing data for the morphological parameters (Kvitblikvatnet, Langen, Harasov, Hermanicky, Kasparovo Lake, Medonosy, and Tupadly).
The selection of the test was based on the analysis of the normality data distribution in each morphological parameter. If a nonnormal distribution of the data set was detected, a logarithmic transformation was performed. In case the transformation was not effective, a nonparametric test version, the Kruskal–Wallis test, was used. Exact information on transformations and tests are given in Table 2. Specifically, morphological parameters of leaf width, length, and area and length of internodes were analyzed. The Kruskal–Wallis Z test with Bonferroni correction was used for subsequent comparison of individual groups.
The statistical relationships between the morphometric parameters and site conditions were tested by linear regression using the environmental factors as independent variables and the morphometric parameters as the dependent variables. If the assumptions for the use of linear regression were not met, a logarithmic transformation of the dependent value was performed. Part of the variability described by the regression model was given by the coefficient of determination (R2), while the statistical significance of the regression was tested by analysis of variance. All analyses of the morphological parameters were performed in NCSS (Hintze, 2001).
. Results
Site Conditions and Vegetation Characteristics
The PCA analysis revealed four well-separated groups of localities that differed in environmental conditions: (i) the first contained most of the localities from Norway and was characterized by a high content of dissolved oxygen and high pH; (ii) the second included localities from Sweden and the Norwegian locality of Dovatnet; this group had a high water depth, and good water transparency; (iii) the Czech localities create a relatively compact group with high shade level, electrical conductivity, and water- and air temperature; (iv) the two Polish localities Jastrząb and Iłżanka, which are located between groups (i) and (iii). According to the species composition and site conditions, the last two groups can be merged into one group, having the highest trophy of the whole set. The first two axes of the PCA explained 75% of all variability of the data (Figure 2).
Figure 2
PCA of ecological variables. Comparison of localities with Potamogeton praelongus in the Czech Republic (black circles), central Poland (yellow triangles), Norway (green diamonds), and south Sweden (red squares). The first axis explains 54% of data variability, while the first two axes explain together 75% of data variability.
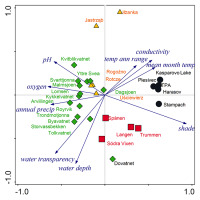
Figure 3
DCA. Species composition of vascular plants in Potamogeton praelongus localities in the Czech Republic (black circles), central Poland (yellow triangles), Norway (green diamonds), and south Sweden (red squares). The first axis explains 11% of the data variability, while the first two axes together explain 18% of the data variability. Only the 50 most important species are shown. Abbreviations of species: BatrCirc – Batrachium circinatum; BatrFlui – Batrachium fluitans; BatrPelt – Batrachium peltatum; BatrTric – Barachium trichophyllum; CaldParn – Caldesia parnassifolia; CallHaml – Callitriche hammulata; Call×Vg – Callitriche ×vigens; CertDemr – Ceratophyllum demersum; ElodCand – Elodea canadensis; GroeDens – Groenlandia densa; HippVulg – Hippuris vulgaris; HydrMors – Hydrocharis morsus-ranae; IsoëLacs – Isoëtes lacustris; LemnMinr – Lemna minor; LemnTris – Lemna trisulca; LitrUnif – Litorella uniflora; LoblDort – Lobelia dortmanna; MyriAltr – Myriophyllum alterniflorum; MyriSpic – Myriophyllum spicatum; MyriVert – Myriophyllum verticillatum; NajMarn – Najas marina; NuphLute – Nuphar lutea; NuphPuml – Nuphar pumila; NympAlba – Nymphaea alba; NympAlbPr – Nymphaea alba var. purpurea; NympCand – Nymphaea candida; PersAmph – Persicaria amphibia; Pota×Ni – Potamogeton ×nitens; PotmAcut – Potamogeton acutifolius; PotmAlpn – Potamogeton alpinus; Potm×An – Potamogeton ×angustifolius; PotmBerc – Potamogeton berchtoldii; PotmCris – Potamogeton crispus; PotmGram – Potamogeton gramineus; PotmLucn – Potamogeton lucens; PotmNatn – Potamogeton natans; PotmObts – Potamogeton obtusifolius; PotmPerf – Potamogeton perfoliatus; PotmPrae – Potamogeton praelongus; PotmTric – Potamogeton trichoides; SparGram – Sparganium gramineum; SpirPolr – Spirodela polyrhiza; StrtAloi – Stratiotes aloides; StucFilf – Stuckenia filiformis; StucPect – Stuckenia pectinata; UtrcAust – Utricularia australis; UtrcMinr – Utricularia minor; UtrcVulg – Utricularia vulgaris.
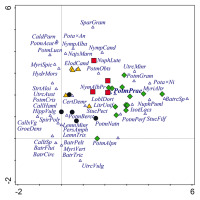
TWINSPAN analysis divided all 31 localities into three groups according to their similarity in species composition (Table 3, Table 1). The synoptic table (Table 1) shows the frequency of the aquatic plant species in each of these groups. Species that occurred in all three clusters included Potamogeton praelongus, P. natans, P. perfoliatus, Nuphar lutea, Elodea canadensis, and Stuckenia pectinata.
According to the DCA (Figure 3), the grouping of the localities based on particular site conditions slightly changes if species composition in the localities is considered. The first two axes in Figure 3 explain 18% of all variability, while the first four axes explain about a quarter of all variability. The most distinctive localities are those in Norway and Poland. Norway has the highest number of localities with the widest range of site conditions and species diversity.
The field measurements showed the lowest electric conductivity in Kvitblikvatnet (18 µS cm−1) and Dovatnet (22.5 µS cm−1) lakes, where the oligotrophic species Isoëtes lacustris and Utricularia minor grow. The highest conductivity was measured in Yttre Svea (140 µS cm−1), Lomsen (138.5 µS cm−1), Storvassbekken (130.4 µS cm−1), and the Malmsjoen lakes (114.6 µS cm−1).
Most Norwegian lakes (except for Yttre Svea and Byavatnet) and the Swedish locality of Spanen belong to the first group of sites characterized as mesotrophic water bodies. These lakes with water depths of more than 1.5 m are characterized by a high content of dissolved oxygen and high pH (7.98–8.76), low conductivity (22–138.5 µS cm−1), and water transparency of 1.1–3.6 m. The mean monthly temperature median for all sites is 1.6 °C, with the lowest from Arvillingen (−0.3 °C) and the highest from Spanen (6.2 °C). The most frequent aquatic plant species were Potamogeton alpinus, P. perfoliatus, Potamogeton ×nitens, and Myriophyllum alternifolium (Table 1). Potamogeton praelongus grows in Spanen Lake together with P. lucens, P. natans, Nymphaea alba, and Nuphar lutea.
Several localities in Sweden (except for Spanen), Poland (Rogoźno, Uściewierz), and Norway (Yttre Svea and Byavatnet) belong to the second group, which is characterized by higher mean monthly temperature (median 6.2 °C), lower pH (7.4–8.05), higher conductivity (54.7–144.7 µS cm−1), and shade level of 8%–43% during observations in July. These localities are typified by having deep water (1.5–4.5 m) and excellent water transparency (up to 3.6 m). Some P. praelongus lake localities are more shaded by woody vegetation around the lakes (Langen 35%, Trummen 43%). The most frequent aquatic plant species were Potamogeton natans, P. obtusifolius, and Nuphar lutea (Table 1). Potamogeton praelongus grows with Nymphaea candida, Hydrocharis morsus-ranae, Myriophyllum spicatum, Utricularia australis, and Stratiotes aloides in the Polish localities of Rogoźno and Uściewierz. All these localities are affected by fishing and recreation. The P. praelongus population in Rogoźno Lake is influenced by ducks, while the population in Trummen Lake in Sweden is threatened mainly by white fish and crayfish. Trummen Lake is located in the surroundings of Vaxjo, and its current eutrophication is caused by past dumping of local waste from a hospital and several factories. On the other hand, oligotrophic species like Isoëtes lacustris, Littorella uniflora, and Lobelia dortmanna were observed in the locality of Sodra Vixen.
The Czech locations (black points in Figure 2) are obviously distinct from the others; they were more shaded and warmer (mean monthly temperature median of 8.2 °C, observed water temperature in July 16.9–21.8 °C) with very high electrical conductivity (275–410 µS cm−1) during the observations in July. High values of both these water characteristics (temperature 16–26 °C, conductivity 192–333.5 µS cm−1) were also found in three Polish locations, Iłżanka, Jastrząb, and Rotcze, in July. Only one Norwegian location (Dagsjoen) belongs to this group because of its high conductivity (303 µS cm−1). The highest content of dissolved oxygen and the highest pH value was detected in the lido of Jastrząb, which is the most exploited for recreation among the Polish localities in the study. All the locations in this group are the most affected by anthropogenic factors in the whole set and are dominated by natant or unrooted macrophytes (Potamogeton natans, P. berchtoldii, Stuckenia pectinata, Elodea canadensis, Ceratophyllum demersum, Lemna minor, and Persicaria amphibia; Table 1). Potamogeton praelongus grows there also together with Nuphar lutea, as well as Potamogeton crispus and Callitriche spp. in the Czech localities. We observed animal grazing of P. praelongus in all Czech and Polish study locations of this group.
Site conditions significantly impacted the species diversity of vascular plants in particular localities (Figure 4). The first two axes explained 16.14% of the total variation, and these axes also explained 45% of the variability described by the explanatory variables. The explanatory variables affect 35.8% of total data variability. The CCA analysis was statistically significant, with the permutation test of the first axis F = 1.9, p = 0.003 and all axes F = 1.3, p = 0.002. The most shaded populations (average 60%) impacted by higher water temperature (average 18 °C) and electrical conductivity (average 360 µ S cm−1) are in the CR. By contrast, the Norwegian localities are less shaded (average 5%), have lower nutrients (average conductivity 114 µ S cm−1), and are cooler (average temperature 15 °C). Conductivity, shade level, and their interaction are significant factors, while the least significant factors are pH and the content of dissolved oxygen in the water (forward selection). All considered variables together explain 35.8% of the total variation. Of this variation, conductivity contributes 22.8% (F = 2.3, p = 0.001), shade 18.5% (F = 18.5, p = 0.002), air temperature 17.3% (F = 1.7, p = 0.002), water temperature 16.8% (F = 1.7, p = 0.008), and water transparency 15.9% (F = 1.6, p = 0.13). Meanwhile, water depth contributes 15.5% (F = 1.5, p = 0.054), pH 12.1% (F = 1.2, p = 0.219), and oxygen 8.6% (F = 0.8, p = 0.699).
Figure 4
Mutual similarity of localities based on species composition correlated with environmental data. Localities of Potamogeton praelongus in the Czech Republic (black circles), central Poland (yellow triangles), Norway (green diamonds), and south Sweden (red squares). CCA analysis: The first axis explains 9% of the data variability and the first two axes together explain 16.14% of the data variability.
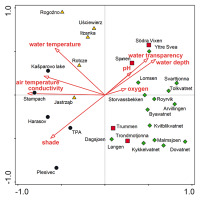
Morphological Parameters of Potamogeton praelongus
Plants in different localities differed significantly in all measured parameters (Table 2, Figure 5). The Czech individuals of Potamogeton praelongus had the widest leaves and the largest leaf area. On the other hand, the length of their shoot internodes was the shortest, as they grow in shallow waters. In the Nordic localities, P. praelongus individuals have longer leaves and longer shoot internodes; the longest ones were found in the Norwegian Lake Tolkvatnet, where individuals were collected from water at depths of 3–4 m.
Figure 5
Differences in morphological parameters of Potamogeton praelongus in particular groups. Letters a, b, and c characterize group differences based on multiple comparison tests (Kruskal–Wallis Z test with Bonferroni correction). Grouping of study sites follows that shown in Table 3.
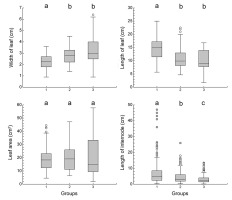
Linear regression revealed that morphometric parameters of P. praelongus individuals were slightly influenced by some water site conditions (Figure 6). The regression model was significant in the case of water conductivity and transparency. Conductivity affected both leaf length (R2 = 0.256, F = 7.569, p = 0.012) and width (R2 = 0.173, F = 4.603, p = 0.043), while water transparency was correlated with leaf length (R2 = 0.175, F = 4.658, p = 0.042).
. Discussion
Sensitivity to Changes in Site Conditions and Impact of Other Organisms
While suitable localities for Potamogeton praelongus have nearly disappeared in the CR, this species survives in Northern Europe in large populations that are noticeably different from those in the CR. In the mid-Holocene, conditions in Central Europe were like the Nordic sites, as confirmed by achenes found in sediments of a Holocene lake near Trebon in South Bohemia (Jankovská, 1980), in the Holocene lake of Sur in western Slovakia (Petr et al., 2013; Potůčková, 2015), in Jezioro Lake in south Poland (Fajer et al., 2012), and in lakes in Greenland (Eisner et al., 1995).
Bennike and Anderson (1998) suggested that temperature changes with increased extremes were the factors that caused P. praelongus to disappear after the early and mid-Holocene in the central part of East Greenland because of subsequent changes in water chemistry.
Seasonal temperature stratification during the growing season is known to occur in large ponds and lakes (Sculthorpe, 1967) while, in small water bodies, water temperature is influenced by many factors like their size, water input, springs, and groundwater fluxes (Sakura, 1993).
In our study (July 2014, 2015), water temperature in the Potamogeton sites ranged from 16 to 26 °C in the Czech and several Polish localities, and from 12.9 to 17.2 °C in the Nordic ones. For example, the summer median water temperature was 14.8 °C in Danish lakes (Lauridsen et al., 2015). The highest water temperature in the Czech localities was detected in shallow backwater pools (temperatures >25 °C continuing for several days), where damage to shoots by overheating was visible (own observations). Vital and undamaged shoots were observed only in the deepest part (more than 1 m) of the backwater pool at Stampach in the Protected Landscape Area (PLA) Kokorinsko (Prausová, Kozelková, Tomášová, Brodský, Havelka, Dvořák, et al., 2017). We observed declining water depth during the summer at all Czech localities, which was reflected in higher water temperature and lower transparency and related to the quantitative increase of phytoplankton and filamentous algae. Increased temperature may lead to prolonged and more intense anoxia phases (Portnoy, 1991), which may stimulate microbial processes (Mulholland et al., 1997) and increase nutrient availability (Pant, 2007), which is related to macrophyte stress (Weltzin et al., 2003). This situation may result in a rapid succession in small backwater pools over a short time, as we observed in PLA Kokorinsko (Harasov, Plesivec, Stampach, Tupadly).
Shading of the water surface was one of the most statistically significant factors in this study. Our measurements showed that P. praelongus prefers sites with more than 40% light. Extensive shading of the water surface decreases the probability of aquatic plant occurrence. On the other hand, submerged macrophytes in shallow lakes can form dense vegetation that controls the phytoplankton biomass through several mechanisms, including allelopathic interactions (Gross, 2003), nutrient limitation (van Donk et al., 1993), and shading, as well as by sheltering the zooplankton that can graze on and reduce the phytoplankton (Burks et al., 2002; Schriver et al., 1995; Timms & Moss, 1984).
In most localities, P. praelongus grows in neutral to slightly alkaline waters (the CR: pH 7.1–8.4; Poland: pH 8.2–9.8; Norway and Sweden: pH 7.4–9.4). The chemical reaction of water (pH) and water movement are related to the availability of carbon dioxide in water by maintaining the equilibrium between inorganic carbon and carbon dioxide (Bornette & Puijalon, 2011). Potamogeton praelongus is able to use HCO3− ions efficiently as a source of carbon for photosynthesis, which gives it an advantage when growing in hard water (Husák & Adamec, 1998). In all Czech localities, calcium concentration ranges from 50 to 60 mg L−1 (Prausová, Kozelková, Tomášová, & Brodský, 2017). Total alkalinity higher than 1.2 mekv L−1 is beneficial for this species (Husák & Adamec, 1998).
A high ion content may signify not only hard but also eutrophicated water. The highest conductivity was measured in the Czech localities (275–401 µ S cm−1) and the Polish Iłżanka lagoon (333 µ S cm−1), while values of 192–281 µ S cm−1 were measured in other Polish localities. While eutrophication of the Czech localities and the Polish Iłżanka lagoon comes from the surrounding agricultural landscape, the Polish locality lido of Jastrząb is affected by recreation activities. Eutrophic water in the Czech P. praelongus localities is determined by N and P concentrations, with (NO3−) N concentration between 0 and 14 mg L−1, and (PO43−) P concentration between 30 and 1,000 µ g L−1 (Prausová, Kozelková, Tomášová, & Brodský, 2017). Conductivity values are significantly lower in the Nordic sites, e.g., 99.4 µ S cm−1 in Finland (Rintanen, 1996), and 70.5–137 µ S cm−1 in Greenland (Vöge, 2002). In our study, lakes with the highest trophy in Norway and Sweden were distinguished by having an electrical conductivity higher than 100 µ S cm−1 (10 lakes), while it was less than 100 µ S cm−1 in lakes with the lowest trophic level (eight lakes).
We observed the negative influence of animals (mainly grazing) on P. praelongus in several of the study locations. It is known (Husák & Kaplan, 1997) that the last but one native population of P. praelongus in the CR (Jezuitske Lake) was destroyed by Ctenopharyngodon idella. The current Czech and several Polish locations for P. praelongus are endangered by aquatic birds (especially ducks), while the population in Trummen Lake in Sweden is threatened mainly by white fish and crayfish (Strand, 2017). Although ducks grazed P. praelongus in other Polish localities (e.g., Rogoźno Lake), only the upper parts of shoots were damaged, owing to the depth of the water.
Species Composition and Biodiversity of Plant Communities
A low number of vascular plant species is a typical feature of the association of Potametum praelongi Hild 1959 in the frame of the Potamion Miljan 1933 alliance (Šumberová, 2011). Based on the similarity of vascular plant species composition in the 31 studied localities, we determined three groups that differed in site conditions, mainly electrical conductivity, water depth, and transparency. In nutrient-rich conditions, competition for light between phytoplankton and macrophytes results in reduced cover and even the disappearance of macrophytes (Declerck et al., 2005; Muylaert et al., 2010; Scheffer et al., 1993). Potamogeton praelongus grows in water bodies with water depths of 0.26–1.05 m in the Czech localities, while water depths of 1.5–4.5 m were found in most of the observed Nordic ones. Species typical of shallow waters can be found in most of the Czech localities, e.g., Callitriche sp., Calliergonella cuspidata, Batrachium sp., Lemna sp., Spirodela sp., while Potamogeton species, Myriophyllum alterniflorum, and Nuphar pumila grow in deep waters in the Norwegian and Swedish lakes. Czech natural localities, e.g., river oxbows, have similar site conditions and macrophyte plant communities to the most eutrophicated Polish localities (Iłżanka lagoon and the lido of Jastrząb), and one part of the Swedish restored site in Trummen Lake (Strand, 2017). The decline of P. praelongus in eutrophic sites is connected to the spread of competitive macrophytes, like Nuphar lutea (Prausová, 2016; Prausová, Kozelková, Tomášová, Brodský, Havelka, Dvořák, et al., 2017) and littoral species like Alopecurus aequalis, Mentha aquatica, Ranunculus flammula, Veronica beccabunga, or Phragmites australis in small and shallow pools, as is known from Poland (Nowak & Nowak, 2004), the CR (Prausová et al., 2011; Prausová, Kozelková, Tomášová, Brodský, Havelka, Dvořák, et al., 2017), Finland (Rintanen, 1996), Estonia (Mäemets et al., 2010), and Lithuania (Balevičienė & Balevičius, 2006). There has been a continual expansion of the broadleaved Nuphar lutea in the last native Czech locality of P. praelongus, spreading over more than 70% of the water surface. The same situation was found in Kasparovo Lake with a reintroduced population of P. praelongus in the Orlice River floodplain (Prausová, 2016; Prausová, Kozelková, Tomášová, Brodský, Havelka, Dvořák, et al., 2017). In artificial backwater pools planted with a P. praelongus outdoor rescue culture in PLA Kokorinsko, the succession was considerably faster than in river oxbows or lakes, with P. praelongus, P. natans, or P. lucens being suppressed by Calliergonella cuspidata in 7–10-year-old backwater pools (Prausová, Kozelková, Tomášová, Brodský, Havelka, Dvořák, et al., 2017).
Morphological Adaptations and Probability of Generative Reproduction in Relation to Site Conditions
Elongated shoot height and increased specific leaf area are morphological adaptations of macrophytes to increasing water depth (Fu et al., 2012, 2014; Maberly, 1993). Leaf area could be an indicator of light-limiting conditions favoring plants with a large leaf area, such as large-leaved or dense-leaved plants, over plants with a small leaf area (Stefanidis & Papastergiadou, 2019). In our study, Central European and Nordic P. praelongus individuals significantly differed in their shoot length, the shape, width, and length of leaves, and length of shoot internodes. Czech individuals had the widest leaves and the largest leaf area probably because of low water transparency and the shortest shoot internodes due to the low water depth in all localities. Exposure to currents or waves strongly influences plant growth, development, reproduction, and clonal growth (Doyle, 2001; Strand & Weisner, 2001). The minimum light requirement of submerged macrophytes is linked to their growth form (Middelboe & Markager, 1997). The length of submerged shoots is limited by water depth; thus, the internodes and whole shoots are considerably shorter in Czech than in Nordic individuals. Leaf length was significantly influenced by conductivity and water transparency, while leaf width was affected by electrical conductivity and the level of shading of the surface. High conductivity probably indicates better plant nutrition and related intensive growth, which can be reflected in leaf size. Wider leaves and larger leaf areas are important to ensure enough insolation for photosynthesis in the Czech localities.
Although the reproduction of P. praelongus was not a focus of our study, we observed that the Nordic populations rarely reproduced with achenes. Although achenes of P. praelongus gathered in Czech localities can germinate after interruption of their dormancy (Prausová et al., 2013; Prausová, Sikorová, & Šafářová, 2015), seed germination success and subsequent growth of the plantlets in natural sites in Central Europe is expected to be relatively low because of low water transparency, threats by herbivores and competitive organisms (algae, mosses, vascular plants, ducks, and fish), and disturbances (such as floods). However, small plantlets that had germinated from seeds were observed in natural localities in Norway (Prausová, Kozelková, Tomášová, Brodský, Havelka, Pitelková, & Hašler, 2017).
Recommendations for Nature Conservation in the CR
In the CR, rescue programs to support the most endangered species, like P. praelongus, are on-going (Prausová, Kozelková, Tomášová, Brodský, Havelka, Dvořák, et al., 2017). The effectiveness of the programs depends heavily on better knowledge of the life-history traits of the species, which will be of great importance for environmentally friendly waterways engineering (Bornette & Puijalon, 2011). Activities implemented as part of the rescue program include not only site- and population monitoring and selection of potential localities for planting P. praelongus shoots from in-vitro culture and rescue cultivation but also protection of the last native site of this species in the CR. Temporarily Protected Area has been incorporated into the Natura 2000 network as the Natural Monument Orlice since 2017. Revitalization of this site was realized in winter 2018/2019. The aim of the revitalization was to decrease the trophic level by the excavation of mud and efficient protection against pollution from various sources (e.g., wastewater treatment plants), to differentiate water depth, eliminate incoming sediments, and reduce the cover of competitive macrophytes, riparian vegetation, and shading trees.