Introduction
Xerothermic vegetation forming climax communities in the steppe and forest-steppe zones in South-Eastern Europe and Central Asia has an extra-zonal character in Central Europe. Its localities in Poland are, at least partly, relics of one or several migration waves dating back to the late Pleistocene (Hewitt, 1999; Paul, 2012). The occurrence of chalk grasslands originating due to forest cutting is determined by a specific combination of topographic, climatic, and soil factors. They are usually seminatural communities emerging through the long-term management of the areas. Burning, mowing, and seasonal grazing by native ungulates have for long been influencing the structure and functions of grasslands in several regions.
The gradual changes in the farming landscape observed in Central Europe throughout the twentieth century, particularly in recent decades, have led to a decline in the surface area of grasslands due to expansion of scrubs, while the development of infrastructure has contributed to fragmentation and isolation of grassland patches in the landscape. Limitation or abandonment of grassland management leads to an overgrowing of expansive grass species, which has a negative influence on grasslands. The most expansive species include Brachypodium pinnatum (L.) P. Beauv. (e.g., Bornkamm, 2006; Endresz et al., 2005; Virágh et al., 2011), Calamagrostis epigejos (L.) Roth (Hegedüšová & Senko, 2011; Somodi et al., 2008), and Arrhenatherum elatius L. (Dostálek & Frantík, 2012). The significant competitive abilities (sensu Grime, 1973) of these clonal grasses, accompanied by bush encroachment, successively lead to diminishing the open grassland area and changes in the microclimatic conditions, which enables forest species to enter. Grassland overgrowing strongly limits the presence of small-scale gaps within vegetation that are free from competition (Bullock, 2000; Harper, 1977), and are necessary to maintain and renew populations of many valuable components of xerothermic vegetation. The development of expansive grasses also leads to soil degradation (Holub et al., 2012; Pruchniewicz & Żołnierz, 2017; Virágh et al., 2011). On the other hand, grassland burning events that eliminate dry grass turfs are practiced nowadays in Central, Southern, and Eastern Europe, including Poland. They are an example of illegal, uncontrolled burning (often just for “fun”), in contrast to prescribed (i.e., controlled fire), practiced by humans as a component of modern grassland management in other regions (e.g., Kerby et al., 2007; Pereira et al., 2013; Quintana-Ascencio et al., 2018; Scasta et al., 2016; Valkó et al., 2014).
Despite the small geographical range and area, as well as the encroachment of shrubs, trees, and expansive grasses, chalk grasslands in Poland still represent the most species-rich communities that significantly contribute to a high level of regional biodiversity. They comprise several countrywide rare and vulnerable species with specific requirements and narrow ecological amplitude, which form small, often isolated populations (cf. Kaźmierczakowa et al., 2014). Among these is the ragwort species Senecio umbrosus Waldst. et Kit. (Calvo & Aedo, 2015), Asteraceae, formerly known as S. doria subsp. umbrosus (Chater & Walters, 1976), S. umbrosus (B. Czarnecka, 1995; Karczmarz & Sałata, 1984), and S. macrophyllus (B. Czarnecka, 2009, 2010; B. Czarnecka & Denisow, 2014; B. Czarnecka & Kucharczyk, 2014; Fijałkowski, 1980; Grulich & Hodálova, 1994; and others). It was documented as a new species to the Polish flora in the early 1980s in two regions of southeastern Poland: in Pagóry Chełmskie (Chełmskie Hills), on small chalk islets within calcareous fens (Fijałkowski, 1980), and the Central Roztocze region, in xerothermic grasslands on Biała Góra (White Mountain; Karczmarz & Sałata, 1984). As a rare element of the Polish vascular flora, the species has been included in the red list and red data book in the VU (vulnerable) category of threat (B. Czarnecka & Kucharczyk, 2014; Kaźmierczakowa, 2016). For all these reasons, the species was considered to be an interesting subject for ecological studies.
This paper reports a part of long-term studies of an island population of the species, conducted on White Mt since 1988. In the initial period of the investigations, there were various trends in the changes in the abundance and mode of dispersion of ramets in certain patches of the S. umbrosus population (B. Czarnecka, 1995). In the following years, the decrease in the number of ramets and the changes in the structure of this population have been suggested to be the effect of an increase in more competitive plants such as B. pinnatum and/or intrinsic traits of the plant (e.g., B. Czarnecka, 2009, 2010). This research focused on the investigation of changes in: (i) spatiotemporal patterns and (ii) the life-stage structure of individuals along with the transformation of grassland vegetation. Special attention was paid to the effects of expansive grass encroachment vs. grassland burning episodes on the population structure.
Material and Methods
Study Site and Species
The studies were carried out on White Mt (50°28′ N, 23°29′ E; 349 m a.s.l.), a vast hill built of Cretaceous marls and loesses (Figure 1). The southwestern, western, and northwestern slopes with different gradients (10°–45°), covered with shallow rendzinas, are overgrown with a mosaic of vegetation: xerothermic grasslands (both loose and low, and tall and dense ones with the dominance of B. pinnatum), bushes and communities of tall herbs, and beech-hornbeam forests with an admixture of Pinus sylvestris L. (B. Czarnecka, 1995, 2009, 2010).
Senecio umbrosus is a large iteroparous (polycarpic) rhizomatous plant with an erect 70–150 cm high (sometimes up to 190 cm) flowering stem and an inflorescence (subcorymb) consisting of numerous heads. The plant flowers in July–August and its inflorescences are visited by many pollen-feeding insects, mainly Apis mellifera (over 50% of insect visits). It produces approximately 30 achenes equipped with a pappus per head and even 1,000–2,000 per fruiting ramet. Senecio umbrosus forms only a transient seed bank in the soil. Diaspores may germinate during the entire growing season depending on moisture conditions. The rate of genet development is very slow, and the life-span of individuals may last a few decades, even up to 70 years (B. Czarnecka, 1995, 2009, 2010; B. Czarnecka & Denisow, 2014; J. Czarnecka & Czarnecka, 2001; B. Czarnecka, unpbl. data).
Data Collection
I observed the first effects of grassland burning in the study area in spring 1988. For that reason, I selected three permanent patches from those established earlier (B. Czarnecka, 1995) to examine the fates of the S. umbrosus population under the influence of possible fire episodes in the future. The following patches of grassland (belt transects divided into 1 × 1-m squares) were analyzed: low, loose, and sun-exposed (LL) – 12 × 4 m = 48 m2 (48 squares); medium-high and medium-dense (MH) – 10 × 4 m = 40 m2 (40 plots); and high and dense (HD) – 12 × 4 m = 48 m2 (48 plots). They differed in soil conditions, initial floristic composition, grassland cover (particularly of B. pinnatum), ragwort cover and density, and light intensity (Table 1 ). The patches MH and HD that had been burned in 1988 were also burned in the next period in an illegal, uncontrolled, and irregular way. All cases of burning took place in early spring or spring (March–April). The LL patch was not burned throughout the study period, which has been noted every year during observations conducted continually since 1988 in different periods of the growing season.
To establish the abundance and spatial distribution of the population, I marked the location of each S. umbrosus ramet of genets exhibiting both unitary (nonclonal) and iterative (clonal) growth on maps at a 1:10 scale. Plants at the youngest life stages (juvenile and immature) always have a unitary growth. In turn, most mature plants (vegetative and generative) exhibit a clonal architecture. Therefore, to standardize the terminology, the term “ramets” was used for all categories denoting the basic demographic unit used in the analyses. To create life-stage pyramids (Gatsuk et al., 1980; Harper, 1977), I took into consideration four life stages of the ramets distinguished earlier (B. Czarnecka, 1995): juvenile (j); young vegetative or immature (im); mature vegetative (v), i.e., prereproductive together with secondary vegetative (in the resting phase between subsequent reproductive episodes); and generative (g) – flowering and fruiting plants. During 21 seasons (1990–2010), the population characters were studied nine times, in 1990, 1991, 1992, 1995, 1998, 2001, 2004, 2007, and 2010. Additionally, changes in the coverage of the shrub/tree layer and the herb layer were recorded, with special attention to the proportion of S. umbrosus and B. pinnatum.
Table 1
Characteristics of the study patches in the Senecio umbrosus population.
Statistical Analysis
To estimate the spatial patterns of the S. umbrosus population, I used the clustering coefficient referred to as Leksis’ index (Steinhaus, 1947), taking into account all ramets of unitary and clonal genets. Friedman and Kruskal–Wallis tests were used to check the significance of the differences in the densities between successive years and the three patches, respectively. The trend line equations for the studied attributes (ramet abundance, density, and Leksis’ index) and the coefficients R and R2 were also calculated. I also analyzed differences in the proportion of juvenile and generative ramets in the total number of ramets between the consecutive study periods and the study patches by means of the two-sided test for differences in two structural indices (u test). Statistical calculations were performed using the Statistica 8 software package.
The multivariate analysis included three groups of components for each study patch: (i) population traits represented by mean density and the proportion of 1-m2 plots occupied by the ragwort, which is a measure of the actual use of environmental resources (Gaston, 1991, 2009) and the Leksis’ index; (ii) vegetation characteristics, i.e., species richness of the herb layer, initial and final cover of the shrub/tree layer, herb layer, S. umbrosus and B. pinnatum, number of fire episodes, years after last burning; and (iii) environmental factors, including soil properties determined in a parallel study (B. Czarnecka, 1995), i.e., proportion of skeleton parts, CaCO3 content, Corg content, pH, and light intensity. In the case of temporal changes in the population and vegetation traits, the data were transformed using the compound annual growth rate (CAGR) adapted from economics (Jassy, 2020):
where V(t0) is the initial value of a trait, V(tn) is the final value of a trait, t0 is the initial year, and tn is the final year of the analyzed period.
According to the length of the gradient from the preliminary detrended canonical analysis (DCA), a linear model with principal components analysis (PCA) was used. To analyze the relationships between the population, vegetation, and habitat factors, multivariate ordination methods in MVSP version 3.1 were used (Kovach, 2005). The input data were standardized.
Results
Changes in Numbers and Spatiotemporal Patterns of the Population
Significant changes in S. umbrosus abundance, density, and mode of spatial distribution of the population were observed (Figure 2A,B, Figure 3A,B, Figure 4A,B, Table 2).
In the loose, never-burned grassland with the greatest initial ragwort density (patch LL), Leksis’ index (I) decreased from 4.51 (1990) to 1.12 (2010). Although all the 1-m2 plots were occupied by S. umbrosus throughout the study period, the proportion of plots characterized by varied density changed distinctly: from the relatively even proportion of plots with 20–50 ramets m−2 in 1990–1995 to only two or three classes in the final study periods, with a dominance of plots with one–five ramets m−2. A nearly random distribution was only recorded in 2004 (I = 1.29) and 2010 (I = 1.12), while a uniform distribution was recorded in 2007 (I = 0.87). It was clumped in the other years. There was a 6.8-fold decrease in abundance, with the greatest value between 2001 and 2004 (by a factor of 3), accompanied by a decline in average density of 25.4 and 8.5 ramets m−2, respectively. The density of the ramets varied significantly among all periods (Friedman test; χ2 = 296.97; p < 0.001).
In patch MH, the abundance increased from 1990 to 1998 by a factor of 1.9, accompanied by an increase in the Leksis’ index from 6.63 (1990) to 14.90 (1998), and declined to 3.05 in 2010. In total, the number of ramets decreased by as much as 3.8 times, with the highest decline between 2004 and 2007 (by a factor of 2.7). The average density increased from 6.4 in 1990 to 12.4 ramets m−2 in 1998 and then dropped to 1.7 ramets m−2 in 2010. The differences were statistically significant (χ2 = 128.02; p < 0.001). Simultaneously, the number of 1-m2 plots occupied by ragwort decreased from 36 to 27 (by 25%), which was accompanied by an increase in the proportion of plots with the lowest number of ramets m−2 (only one–five) to ca. 80% (2007, 2010).
Figure 2
Dynamics of the Senecio umbrosus population within patch LL. (A) Spatial pattern of ramets; (B) distribution of plots (1 m2) of different ramet density; (C) life-stage structure of the population. n – number of plots occupied by ragwort; N – total number of ramets; j – juvenile ramets; im – young vegetative (immature) ramets; v – mature vegetative ramets; g – generative ramets.
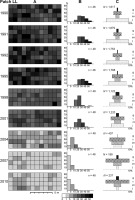
Table 2
Successive changes in abundance, density, and dispersion mode of ramets in the study patches of the Senecio umbrosus population.
In patch HD, an 8.3-fold decrease in abundance was noted with the highest decline between 2004 and 2007 (2.4 times) and between 2007 and 2010 (1.7 times). The values of Leksis’ index declined successively from 11.23 (1990) to 1.72 (2010), and the mean density dropped from 11.8 to only 1.4 ramets m−2, respectively (χ2 = 116.81; p < 0.001). The number of inhabited plots declined from 31 to 23 (a 26% decline). At the end of the study, plots with the lowest number of ramets m−2 dominated (>90% proportion). The differences in densities between the three patches were statistically significant (Kruskal–Wallis test; H = 20.56–84.31; p < 0.001; in 2004: H = 9.90; p < 0.01).
The significant decline in values of the studied attributes (ramet abundance, density, and Leksis’ index) was confirmed by linear regression (Figure 4–Figure 7). The strength of the correlation was very high for all attributes in patches LL (−0.90 < R < −0.94) and HD (−0.95 < R < −0.97), while the correlation for patch MH was strong for abundance and density (R = −0.61) and moderate for Leksis’ index (R = −0.40).
Figure 5
Changes of values and linear regression for abundance, density, and Leksis’ index of the Senecio umbrosus population within patch LL.
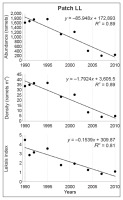
Changes in the Life-Stage Structure of the Population
The transformations in S. umbrosus population abundance and spatial patterns were accompanied by changes in the life-stage structure of ramets (Figure 2C, Figure 3C, Figure 4C, Table 3, Table 4).
In patch LL, the population abundance in 1990–1992 was relatively stable, with dominance of younger ramets (j + im) over the proportion of mature ramets (v + g). In 1995–2001, the ramet dynamics exhibited an oscillatory character, and the proportion of younger to mature plants was more balanced. From 2004 to the end of the study, a dramatic decrease in the proportion of juveniles was noted. The percentage of juveniles changed significantly (u test; p < 0.001), with only a few exceptions (Table 3). The percentage of generative ramets with a maximum value of 10.2% in 1998 and minimum value of 0.2% in 2004, changed significantly in the majority of cases (0.001 < p < 0.05), except for some cases between consecutive seasons (Table 4).
Between 1990 and 2001, the percentage of the youngest ramets within patch MH was relatively high and stable (maximum 68.6% in 1998). Subsequently, it dropped drastically to only 5% in 2010. The differences in the contribution of juveniles between the study years were statistically significant (0.001 < p < 0.05), with some exceptions (Table 3). The proportion of generative ramets changed all the time, but the differences were statistically nonsignificant in the majority of cases (0.001 < p < 0.05; Table 4).
In patch HD, the proportion of younger ramets was relatively high and stable in 1990–1992, i.e., in the period of the greatest abundance. Together with the drastic decline in the numbers during 2001–2007 (Table 2), a sudden drop in the proportion of juveniles took place and no juveniles were observed in 2007. In the majority of cases, the differences in the proportion of juveniles were statistically significant (0.001 < p < 0.05; Table 3). The proportion of reproductive ramets decreased significantly or nonsignificantly until 2004 (0.001 < p < 0.05; Table 4), and no flowering plants were observed in the last two study seasons (Figure 4).
The percentage of juveniles between the study patches differed significantly (u test; 0.001 < p < 0.05), with the exception of MH–LL pairs in 1991 and all patches in 2010, while the differences in the proportion of generative ramets were statistically significant (0.001 < p < 0.05), except for pairs MH–HD (1992, 2007, 2010), MH–LL (1992, 2004), and HD–LL (1991, 1998, 2001).
Table 3
The significance of differences in the percentage of juvenile ramets in the study patches of the Senecio umbrosus population; data analyzed with the u test.
Table 4
The significance of differences in the percentage of generative ramets in the study patches of the Senecio umbrosus population; data analyzed with the u test.
Relationships Between Population Traits, Vegetation Characters, and Environmental Conditions
The use of PCA facilitated determination of the key factors influencing S. umbrosus population traits. Axis 1 of the ordination diagram explains 53%, and the first two axes explain 100% of the cumulative variability of the data. Years after the last burning, shrub/tree cover, and number of burning episodes were statistically significant variables (p < 0.05), with the strongest positive correlations with Axis 1. The number of plots occupied by ragwort, light intensity, and B. pinnatum cover exhibited the strongest negative correlations with this axis. In the case of Axis 2, only light intensity, number of plots colonized by ragwort, and years after last burning were statistically insignificant, whereas the strongest correlation was found for CaCO3 content, density, and cover of ragwort. A negative correlation with this axis was found for Corg content, herb layer cover, and B. pinnatum cover (Table 5).
Table 5
Results of principal component analysis (PCA) based on Senecio umbrosus population traits, vegetation characters, and habitat factors; population and vegetation traits corresponding to temporal changes were transformed using the CAGR.
The greatest effect on the density and Leksis’ index was exerted by the CaCO3 content and burning activity (number of episodes, years after last burning); this was noted especially in patch MH (Figure 8). The highest decrease in the proportion of ragwort in patch HD was caused by an increase in the herb and shrub/tree cover, and a high Corg content was associated with the accumulation of necromass. In the patch LL (the highest initial cover and density of the ragwort), the decline in the contribution of the ragwort was induced by an increase in species richness in the herb layer, in particular, the B. pinnatum cover (Table 1). In turn, high sun exposure, pH, and the proportion of skeleton parts in soil promoted the persistence of a high proportion of the study species (abundance, density, cf. Table 2).
Figure 8
Ordination diagram showing the results of the PCA for the Senecio umbrosus population traits, vegetation characters, and environmental conditions; for details see Table 5.
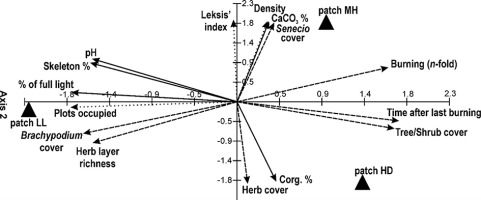
Discussion
Changes in the population abundance, density, and spatial patterns of individuals are considered fundamental in plant demography (Herben & Hara, 2003; Watkinson, 1997). They may follow different spatial and temporal patterns of biotic heterogeneity. The latter depends on hierarchical selection and the competitive ability of plant individuals and tends to be more dynamic than heterogeneity of the abiotic structure of the environment. Environmental heterogeneity in terms of geomorphology, bedrock, and soil depth and texture tends to produce patterns that do not change over time, which contrasts with biotically generated heterogeneity of both aboveground (space, light) and belowground (humidity, nutrients) resources that tends to be dynamic (Herben et al., 2001; Law et al., 2001). The dominant process of competition involves occupation of space (Grubb, 1986), which is strengthened by ecosystem disturbances (Herben & Hara, 2003; Pickett & White, 1985).
Throughout the study period, varied dynamics of the numbers, spatial patterns, and life-stage structure were observed in analyzed patches of the S. umbrosus population. The highly clumped spatial organization pattern (I > 10) was characteristic of a part of the population area within the habitat mosaic, in which shrub/tree groups were accompanied by smaller or larger clusters of ragwort ramets (e.g., patches MH and HD), with solitary plants growing in the open grasslands between these groups. Fluctuations in the clumping degree in these patches might be related to irregular fire episodes and periods of intense B. pinnatum overgrowing and accumulation of its necromass. The gradual decrease in abundance within these patches was accompanied by a decrease in the clustering coefficient and gradual equalization of the spatial distribution of ramets, with pronounced dominance (80%–90%) of plots with one–five ramets. At all times, in the initially low, loose, and sunlit grassland (patch LL), the clustering coefficient was lower than that within the other two patches. Starting in 1998, the mode of spatial distribution was closer to random and even uniform in this patch, with dominance of plots with the lowest number of ramets m−2.
Initially, a large proportion of younger ramets in all the patches suggested the expansion phase of the population. In the 1990s, the number of ramets persisted at a relatively stable level, or even increased periodically, which is typical for the fluctuation phase of population growth. The increase was caused mainly by the recruitment of new individuals and, to a lesser extent, through expansion of mature individuals; genets may produce one–five new rosettes, the most frequent number being one or two (B. Czarnecka, 1995; and unpbl. data). Together with changes in the life-stage structure (from dominance of juveniles to the dominance of adult genets, partly with iterative growth), the foraging ability of older genets intensifies the self-thinning process. In age- and size-structured populations, particularly at higher densities, the competition between genets becomes asymmetric: The larger (older) competitors suppress the growth of the smaller (younger) individuals, but the latter have no adverse effect on the former (DeMalach et al., 2016; de Ross & Persson, 2003; Ehlers & Bilde, 2019; Law et al., 1997; Weiner, 1990).
In the last decade, the fate of the population differed in particular fragments of the area, depending on the initial composition and structure of vegetation, the number of fire episodes, and the degree of succession of scrub and grassy vegetation. The most stable abundance and life-stage structure were noted in patch MH. Seedling recruitment and establishment of juveniles were influenced by burning dry grass turfs (six times; every year in 2003–2006), accompanied by a slight decline in B. pinnatum cover. During the 21 years, the number of ramets decreased 3.8-fold in patch MH and as much as 8.3-fold in patch HD. The latter patch, which exhibited a high cover of B. pinnatum and considerable shading by shrubs and an older pine stand, was burned four times, with the last events in 2003 and 2004. Subsequently, the thick litter layer (mainly B. pinnatum shoots) underwent rotting with time, limited the input of diaspores to the soil surface, inhibited germination, and led to the increased death of juveniles, resulting in the disappearance of these individuals in 2007. In the unburned patch LL, a 6.8-fold decrease in abundance was observed, but the spatial distribution was relatively more uniform (all 1-m2 plots occupied by ragwort). In the early 1990s, the species growing in loose grassland achieved very high cover, even up to 60%–80% (B. Czarnecka, 1995, 2009). Younger individuals dominated (over 60%), but their proportion declined successively to only a few percent.
The formation of a persistent litter layer is one of the features of the competitive strategy of plants (Grime, 1973, 1979). Necromass may create a physical barrier, impede the recruitment of seedlings, and exert an impact on plant community structure and interspecific relationships (Donath & Eckstein, 2008; Facelli & Pickett, 1991; Loydi et al., 2015; Quested & Eriksson, 2006). With water deficit in the substrate, lower amounts of litter can promote more intensive seed germination and seedling survival (Facelli & Pickett, 1991), and may improve microsite conditions with respect to temperature amplitude (Violle et al., 2006). This was the case in patch MH, where six fire events eliminated excess litter and the moderate cover of B. pinnatum facilitated the development of younger S. umbrosus individuals.
After grassland burning, together with litter reduction, studies have shown that B. pinnatum cover increased (Bornkamm, 2006; Kahmen et al., 2002; Moog et al., 2002; Ryser et al., 1995), the species richness of vegetation declined (Köhler et al., 2005; Ryser et al., 1995), or the species composition remained unchanged (Kahmen et al., 2002; Liira et al., 2009; Moog et al., 2002). Grassland burning on White Mt temporarily eliminated the excess litter, but simultaneously, B. pinnatum cover in patch HD increased during the study period from 40% to 70%. Species richness of the herb layer was similar at the beginning and end of the study, with 47 and 46 species, respectively, while the decline in B. pinnatum cover in patch MH was accompanied by a noticeable decrease in species richness (52 vs. 41).
In the unburned patch LL, the overgrowing of low and loose grassland by high grass species accompanied by massive seeding of Scots pine and the gradual transition of its new growth into undergrowth as well as shrub encroachment from both the foot and top of the hill, resulted in changes in habitat conditions (light, temperature, and soil moisture). Some woodland plants colonized open habitat from the beech-hornbeam-pine forest. This increased the species richness of the grassland from 53 to 70 species, with a simultaneous decline in the cover of valuable grassland components, particularly S. umbrosus (B. Czarnecka, 2009, 2010; B. Czarnecka & Kucharczyk, 2014). The decline in density, successive death of juveniles, and absence of seedling recruitment of ragwort were caused by transformation of the “initially” low and loose grassland into a high and compact type with a considerable proportion of expansive grass species. Among them, B. pinnatum is an immanent component of xerothermic grasslands on a majority of the slopes of White Mt. Within patch LL and its vicinity, its initial cover was very low, although it exhibited a high growth rate. For instance, one of the patches of this grass had a diameter of ca. 2 m in the early 1990s and occupied several dozen square meters at the end of the study period (B. Czarnecka, unpbl. data). In turn, C. epigejos (ruderal species) colonized grassland from abandoned arable fields, thereby increasing its cover from 5% to over 20%. Arrhenatherum elatius (meadow grass), which was absent at the beginning of the studies, reached a cover of ca. 10% at the end of the study period. These grass species contribute to the deposition of a persistent litter layer with a very slow decomposition rate due to high insolation and low moisture.
Therefore, burning of litter does not necessarily promote the development of dicotyledonous species such as S. umbrosus; instead, it may promote the growth of B. pinnatum, a grass species with significant competitive abilities that is relatively fire-resistant due to its underground stolons (e.g., Bornkamm, 2006; Moog et al., 2002). In resprouting grasses, plants previously burned for several years produced proportionally more biomass below ground, suggesting a greater capacity for recruitment and resprouting than unburned individuals (Simpson et al., 2019). However, the intensified spread of B. pinnatum is determined not only by the fire factor and competitive abilities but also, to a large extent, by the habitat conditions in a patch. As shown by multiyear observations on White Mt, B. pinnatum prefers slightly richer soils, i.e., mineral-humic soils, according to its ecological indicator H value of 2 (Zarzycki et al., 2002). These are properties of the habitat within patch HD and similar ones (medium-deep brown rendzina), while patches MH and LL are located on much poorer shallow proper rendzina (B. Czarnecka, 1995; and unpbl. data). This grass species does not seem to be attached exclusively to exposed habitats with full light, as indicated by its L value of 5 (Zarzycki et al., 2002). In habitats with moderate light and even in half-shade, but which are slightly more fertile, B. pinnatum spreads very efficiently and achieves higher growth parameters.
After fire, the population size of nonsprouting species fluctuates more than that of sprouting species. In addition, species that are slow to mature are particularly vulnerable to fire when populations are burned before they have first flowered and set seeds (Bond, 2001). Ragwort is a “steppe” species with a very long life-span (B. Czarnecka, 1995, 2009; and unpbl. data). It remains in the vegetative phase for a long time and creates a bank of persistent juveniles (Grime, 1979). The effect of burning on seedling appearance and establishment of new genets seems to be ambiguous. Fires may act at a relatively large spatial scale (DeSimone & Zedler, 1999; Pickett & White, 1985) or they can be patchy (as in the study area) and create complex mosaics of both unburned and burned patches that affect plant regeneration (Menges et al., 2017 and literature cited therein). Seedling recruitment is associated with rather small-scale disturbances, i.e., small gaps suitable for germination and seedling survival (B. Czarnecka, 1995, 2009; DeSimone & Zedler, 1999; Seabloom et al., 2005).
Periodical disturbances such as burning may contribute to changes in availability of resources and soil properties. After fire, cation nutrients in ash tend to be mobile and in a plant-available form (Pereira et al., 2012). Their presence leads to increases in soil pH, which are smaller in neutral and alkaline soils than in acidic soils (Bond, 2001; Rodríguez et al., 2009). The soil reaction in the study area is in the pH range of 7.38 to 7.63 (B. Czarnecka, 1995), and the pH increases do not seem to be substantial. A reduction in litter ensures greater solar radiation, and higher pH leads to an increase in the microbial activity and the rate of mineralization, but the increased availability of nutrients after fire episodes is rather short-term (Bond, 2001). The reduction of necromass by burning and changes in soil properties (Bond, 2001; Granged et al., 2011; Pereira et al., 2012; Rodríguez et al., 2009) alter hydrological conditions and may lead to greater soil erosion and nutrient loss with water runoff; for example, after heavy rainfall, especially on slopes with high inclination, as on White Mt (30°–40° within patches MH and HD).
In the case of species forming persistent seed banks, the recruitment of seedlings after fire depends mostly on dormant seeds (Ooi et al., 2007, 2014; Pausas et al., 2004). When the species forms only a transient seed bank in soil, such as S. umbrosus (J. Czarnecka & Czarnecka, 2001), it requires seed dispersal from unburned patches, similar to other species from Asteraceae (Rodrigo et al., 2012). A probable shortage of both seeds and safe sites was observed, particularly in the neighboring patches MH and HD at the end of the study period. A drastic decrease in the proportion of juveniles was noted after the last four and two grassland burning events in patches MH and HD, respectively. The shortage of seeds was also caused by a decline in the number of generative ramets, and the absence thereof within patch HD (2007 and 2010). The lack of diaspores can also be caused by water runoff on steep slopes, depending on rainfall and soil characteristics (Bochet et al., 2015).
Conclusions
Population abundance, structure, and organization are affected by species traits and habitat conditions. A drastic decline in the S. umbrosus abundance within all grassland patches was accompanied by a decrease in the clustering coefficient and gradual equalization of the spatial distribution of individuals. The temporal transformations in population abundance and spatial patterns were accompanied by changes in life-stage structure, with a significant drop in the proportion of both juvenile and generative ramets.
In addition to environmental causes, such as an increase in the coverage of expansive grasses and grassland overgrowing by shrubs and trees, the significant decline in S. umbrosus population abundance seems to be caused by processes occurring at the genet level, as suggested earlier. The most important factors include plant senescence, weakened biotic (reproductive) potential, lower proportion of generative ramets, frequency of flowering episodes, and individual fecundity.
As shown by long-term observations of the S. umbrosus population, the effect of burning on seedling recruitment and new genet establishment seems to be ambiguous. It would be necessary to conduct carefully planned research on the impact of fire at the plant population and community levels. However, due to the ban on burning so-called wasteland in Poland, burning xerothermic grasslands is a controversial agricultural treatment, which is still insufficiently tested. Furthermore, this method is difficult to apply because of the extra-zonal character of xerothermic vegetation and usually the low area of patches of grasslands. In turn, other methods have been used in patches of valuable xerothermic grasslands, i.e., cutting shrubs and tree saplings or extensive grazing of sheep, horses, or goats introduced in recent years. Nevertheless, it is believed that occasional burning treatments applied for 5–10 years may support grazing and contribute to preservation of the most valuable components of grasslands.